Introduction
Zygotes with more or less than 2PN are considered to be abnormally fertilized. The most common form of multipronuclear zygotes are 3PNs (Feenan & Herbert, Reference Feenan and Herbert2006). 3PN zygote formation in IVF mostly depends on polyspermia. 3PN oocyte formation occurs more often after ICSI procedures, than after IVF protocols (Staessen & Van Steirteghem, Reference Staessen and Van Steirteghem1997). As a single sperm is injected into the oocyte during ICSI, the reason for 3PN formation is unclear. It has been suggested that the origin of the extra pronucleus is related to an inability to extrude the second polar body (2PB) (Staessen & Van Steirteghem, Reference Staessen and Van Steirteghem1997; Porter et al., Reference Porter, Han, Tucker, Graham, Liebermann and Sills2003; Feenan & Herbert, Reference Feenan and Herbert2006).
The meiotic cycle, fertilization, early events of segmentation and embryogenesis all proceed depending on oocyte factors such as maternal RNA, proteins, organelles, and antioxidants, therefore variation in oocyte quality affects embryonic development (Acton et al., Reference Acton, Jurisicova, Jurisica and Casper2004). Functional competency of organelles is crucial for the quality of oocyte. As in other mammalian oocytes, mitochondria, which are responsible for energy production in eukaryotic cells, are leading in quantity in the human oocyte (Acton et al., Reference Acton, Jurisicova, Jurisica and Casper2004; Thouas et al. , Reference Thouas, Trounson, Wolvetang and Jones2004). Mitochondrial malfunction has also been suggested for the decreased IVF success rate in some studies (Acton et al. , Reference Acton, Jurisicova, Jurisica and Casper2004). When cultured MII stage oocytes were exposed to light for some periods, altered mitochondrial morphology, decreased membrane potential, and inhibited blastocyst development were detected in zygotes developed from these oocytes (Thouas et al. , Reference Thouas, Trounson, Wolvetang and Jones2004).
Changes in the cytoskeleton organization emerge during resumption of the second meiotic cycle of the oocyte and in post-fertilization events. Microtubules are responsible for chromosome organization and mitochondria relocation (Yaffe et al. , Reference Yaffe, Harata, Verde, Edison, Toda and Nurse1996; Kim et al. , Reference Kim, Chung, Cha and Chung1998; Veselska & Janısch, Reference Veselska and Janısch2001; Tremoleda et al. , Reference Tremoleda, Van Haeften, Stout, Colenbrander and Bevers2003; Velilla et al. , Reference Velilla, Rodriguez-Gonzale, Vidal and Paramio2005). Radial microtubule clusters are involved in forming and keeping cellular asymmetry during oogenesis, oocyte maturation and early embryogenesis. The centrosome is known to be the microtubule organization centre (MTOC) of the cell (Combelles & Albertini, Reference Combelles and Albertini2001). Centrosomes in mammalian oocytes are devoid of centrioles and are merely comprised of pericentrioler material (PCM), which is able to form microtubules. γ-Tubulin is a member of the tubulin superfamily, is found in MTOC structures and plays a key role in microtubule commencement and formation of the meiotic spindle (Lee et al. , Reference Lee, Miyano and Moor2000; Combelles & Albertini, Reference Combelles and Albertini2001). Meng et al. (Reference Meng, Fan, Zhong, Zhang, Li, Chen and Sun2004) detected aggregation of γ-tubulin foci in the oocyte cytoplasm and emergence of microtubule arrays from these aggregates in mouse oocytes. γ-Tubulin foci alter their location due to oocyte maturation phases during development and are related to the oocyte cortex and meiotic spindle (Lee et al. , Reference Lee, Miyano and Moor2000; Combelles & Albertini, Reference Combelles and Albertini2001). As for microtubules, microfilaments have important roles during meiotic resumption of MII oocytes, meiotic spindle migration and maintenance of the peripheral localization of the meiotic spindle for asymmetric division and early embryo development. In mammalian oocytes, this thick microfilament domain may function for meiotic spindle upkeep and chromosomal organization (Kim et al. , Reference Kim, Chung, Cha and Chung1998; Veselska & Janısch, Reference Veselska and Janısch2001; Velilla et al. , Reference Velilla, Rodriguez-Gonzale, Vidal and Paramio2005).
ICSI manipulation can cause cytoskeletal damage in zygotes and lead to 3PN (Tremoleda et al. , Reference Tremoleda, Van Haeften, Stout, Colenbrander and Bevers2003). Cortical actin filament organization and spindle generator centrosome location may have important roles during second polar body extrusion in the human oocyte. Furthermore, highly polarized mitochondrial membrane potential may be related to PN formation and PB extrusion. Although various studies have been conducted to investigate the cause of formation of 3PN zygotes after ICSI, there is still a question whether this is due to manipulation or to oocyte-related issues. In this study, actin filament and γ-tubulin localization was examined by immunohistochemistry; the inner membrane potential of mitochondria was evaluated by JC-1 staining, and oocyte ultrastructure was examined by transmission electron microscopy.
Materials and methods
3PN oocytes used in this study were collected from patients (age range 23–47 years) who presented to the IVF centres of the International Hospital, Zeynep Kamil Hospital, and 29 Mayıs Hospital, Turkey, and who had signed the informed consent donation form. In total, 21 oocytes were evaluated in this study.
Electron microscopy
3PN oocytes were fixed using 1% glutaraldehyde solution (Sigma, cat. no. G-777) at 4°C. After rinsing with phosphate-buffered saline (PBS; Sigma, cat. no. P4417) to enable microscopic oocyte recovery, we put each 3PN oocyte into a drop of egg albumin and exposed it to 1% osmium tetroxide vapour (Merck, cat. no. 124505) to coagulate and perform secondary fixation of the oocyte. After fixation, coagulated egg albumin containing the 3PN oocytes was rinsed with PBS and then processed through a graded acetone series. After rinsing with PBS, the oocytes were treated with an acetone:epon mixture in ratios of 1:1, and 1:3, and finally with pure epon (Fluka, cat. no. 45359) for 1 h. The samples were then embedded into epon-containing capsules. The capsules were incubated at 60°C in an incubator (Heraeus) for 18 h for epon polymerization. Ultramicrotomes (Leica EM UC7) and glass knives were used to take ultra-thin sections. Sections were placed on 100-mesh copper and nickel grids. After staining with uranile acetate for 30 min and lead nitrate for 10 min, sections were examined using a Jeol JEM 1011 transmission electron microscope. The images were transferred to a Soft Imaging System Analysis program with a Megaview III digital camera and pictures were taken.
Immunofluorescence study
The 3PN oocytes were fixed in 4% paraformaldehyde prior to staining for immunofluorescence microscopy. After rinsing with PBS, the oocytes were treated with 0.5% Triton-X (Fluka 93418) solution for 30 min. Oocytes were treated with 1% human serum albumin (HSA; Sigma A1653) for 20 min to block non-specific staining and then rinsed with PBS. For γ-tubulin staining, anti-γ-tubulin primary antibody (Sigma, cat no. T5192) was used at a 1:400 dilution in 1% HSA. Oocytes were embedded into a drop of antibody and incubated for 1 h at room temperature. After primary antibody binding, oocytes were rinsed with PBS and incubated with FITC-labelled secondary antibody (Sigma, cat. no. F0382) diluted at 1:1000 in HSA for 1 h in the dark at room temperature. Filamentous actin staining was achieved using phalloidin–fluorescein isothiocyanate (FITC) antibody (Sigma, cat. no. P5282) for 1 h in the dark at room temperature. After incubation, all oocytes were rinsed with PBS. For nuclear staining, 4′,6-diamidino-2-phenylindole (DAPI) containing anti-bleaching agent (Invitrogen Molecular Probes, cat. no. P36935) was applied for 10 min. Oocytes were evaluated and photographed using an Olympus BX51 Research Microscope equipped with a DP72 camera, which was controlled by Olympus DP2-TWAIN software.
Mitochondria membrane potential evaluation
Mitochondrial inner membrane potential was measured using the JC-1 (Sigma CS0390) potential sensitive fluorescent stain. A stock solution was prepared with resolved JC-1 powder in dimethyl sulphoxide (DMSO) at 10 mg/ml concentration and stored at −20°C. A working solution from the kit was made up by mixing a 6 µl stock solution, 1 ml pure water, and 0.25 ml staining buffer. The working solution was added to the culture medium at a 1:1 ratio. Oocytes were incubated in this mixture for 20 min at 37°C. The oocytes were then rinsed with diluted buffer solution. Oocytes were examined and photographed using an Olympus BX51 Research Microscope equipped with a DP72 camera, which was controlled by Olympus DP2-TWAIN software. Oocytes were examined with an U-MWU2 filter cube. High membrane potential mitochondria were seen as an orange-red colour, low membrane potential mitochondria were coloured green.
Results
Electron microscopy
The oocytes that were examined had microvilli on their plasma membrane. Partial or no cytoplasmic halos in the oocyte cortex were observed in 27% and 73% oocytes respectively (Fig. 1a and Fig. 1b). The cortical region of some oocytes had mitochondria related to large vesicles of smooth endoplasmic reticulum (SER-V) in 45% of the oocytes and tubule aggregates in 27% of the oocytes (SER-T) (Fig. 1c and Fig. 1d). In total, 15% of the oocytes had a few cortical granules on their cortical area. The oocytes contained large vacuoles in the cortical region. Ruptured and dilated endoplasmic reticulum (ER) vesicles were detected in 25% of the oocytes (Fig. 1e and Fig. 1f). Aggregation of annulate lamellae was detected in the cytoplasm in close proximity to PNs and also internalized in the PNs associated with genetic material (Fig. 1g and Fig. 1h) in 18% of the oocytes.

Figure 1 (a, b) (a) Cytoplasmic halo in the cortical region of 3PN oocyte. Partial halo formation was scored as halo negative (asterisk), or (b) halo positive (asterisks). (c, d) SER-V and SER-T can still be seen in the cytoplasm. (e) Dilated large SER vesicles (asterisk) in the ooplasm of 3PN oocytes. (f) Ruptured membranes of dilated SER vesicles (arrows) were seen. (g, h) (g) Annulate lamella were seen near the pronuclei also in the pronuclei (h). AL, annulate lamella; mv, microvilli; nm, nuclear membrane; npb, nuclear precursor body; PN, pronucleus; PVS, perivitelline space; ser, smooth endoplasmic reticulum; SER-V, smooth endoplasmic reticulum – large vesicles; SER-T, smooth endoplasmic reticulum – tubule aggregates; ZP, zona pellucida.
Abundant, round or oval-shaped mitochondria were seen around the pronuclear areas, which were sparse in the peripheral area of the cytoplasm. Although there were intact mitochondria with evident cristae and double membranes, mitochondria in 64% of the oocytes with excess intense matrix were also detected (Fig. 2a and Fig. 2b ). Some mitochondria had non-clear cristae, vacuolization in the matrix and degeneration were detected in all oocytes. Multilamellar bodies were also detected in the matrices of those degenerated mitochondria in 64% of the oocytes (Fig. 2c , d). All examined oocytes contained numerous lipofuscin granules in their cytoplasm (Fig. 2e , f). Microtubules were seen in the cytoplasm of all oocytes especially close to the PNs. Spermatic centrioles were also detected near the paternal PN.

Figure 2 (a) Mitochondria have intense matrices (white asterisk) and degenerated mitochondria (black asterisk). (b) Initiation of vacuole formation (arrow) in mitochondria. (c) Vacuolization of mitochondria localized near the pronuclei. (d) Multilamellar body formation in mitochondrial matrices. (e, f) Large lipofuscin granules were seen in the ooplasm of 3PN oocytes. LPG, lipofuscin granule; m, mitochondria; mlb, multilamellar body; nm, nuclear membrane; npb, nuclear precursor body; PN, pronucleus; ser, smooth endoplasmic reticulum.
Maternal, paternal, and extra PN were found mostly in the central location, although eccentrically located PNs in oocytes (18%) were recorded. One PN was located peripherally that had not yet completed its formation. Pronuclear membranes appeared intact. nucleolar precursor bodies (NPBs) were detected close to PNs. Some NPBs had fibrillary form and some were granular. An investigation of serial sections showed that each PN had more than three NPBs. The majority of NPBs showed polarization close to the nuclear membrane but some has peripheral polarization. Non-polarized NPBs were also present (Fig. 3).

Figure 3 Localizations of nucleolar precursor bodies from serial section evaluation. Different coloured circles represent granular and fibrillary NPBs in serial sections. Full circle, fibrillary form; asterisk type circle, granular form; black, first section; red, second section; blue, third section; purple, fourth section.
Immunofluorescence staining
Actin filament distribution was mainly detected at the cortical region of oocytes and no actin filaments were seen around the PNs (Fig. 4a , b).
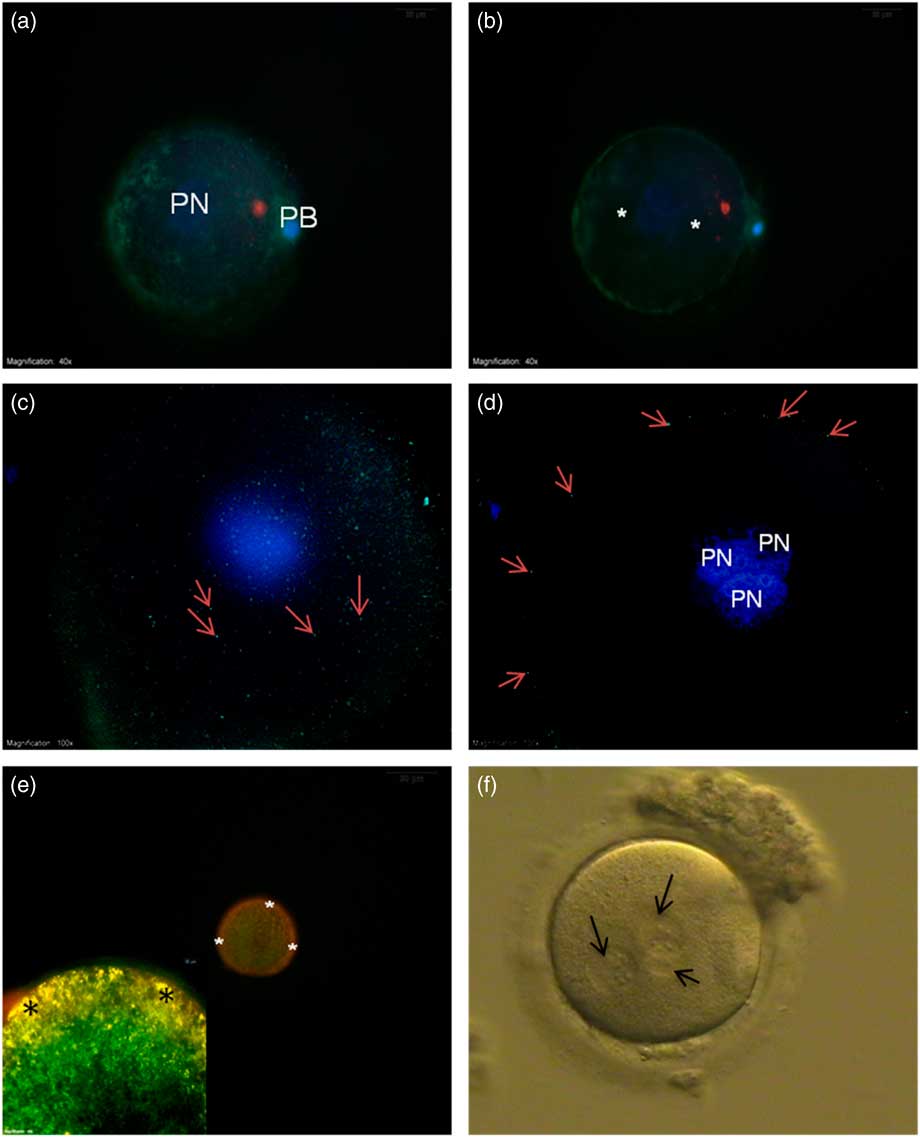
Figure 4 (a, b) FITC–phalloidin-labelled actin filaments (green) from the superficial (a) and central axis (b). No staining were seen around the pronuclei (asterisk). (c, d) γ-Tubulin staining from the superficial (c) and central axis (d) was only seen at the cortical region of the oocyte (arrows). (e, inset) Mitochondrial membrane potential. JC-1 aggregate accumulation at the cortical region shows yellow-orange high membrane potential (asterisk). (f) Bright field microscope image of 3PN oocyte (arrows – pronuclei). DAPI, blue. PB, polar body; PN, pronucleus.
γ-Tubulin was distributed beneath the plasma membrane around the whole oocyte appearing as small particles (Fig. 4c , d).
In the mitochondrial potential evaluation, low-polarized mitochondria with green fluorescent staining were detected around the PNs. In contrast, yellow-orange fluorescing highly polarized mitochondria were localized at the subplasmalemmal region (Fig. 4e ).
Discussion
Abnormal fertilization is one of the major problems in assisted reproductive technology (ART). Usually, 3PN formation after IVF is caused by fertilization with two sperm, however the cause of 3PN formation after ICSI is still a subject of debate. Due to ethical concerns we cannot use 2PN oocytes as a control but we compared our results with studies reported in the literature that represented features of 2PN oocytes (Zamboni et al. , Reference Zamboni, Mishell, Bell and Baca1966; Kim et al. , Reference Kim, Chung and Day1997; Sathananthan et al. , Reference Sathananthan, Selvaraj, Girijashankar, Ganesh, Selvaraj and Trounson2006; Branzini et al. , Reference Branzini, Lavolpe, Nodar and Rawe2007).
Absence of halos in the zygotes has been shown to lead to poor quality blastocysts, lower rates of blastocyst formation and pregnancy failure (Stalf et al. , Reference Stalf, Herrero, Mehnert, Manolopoulos, Lenhard and Gips2002; Zollner et al. , Reference Zollner, Zollner, Hartl, Dietl and Steck2002; Ebner et al. , Reference Ebner, Moser, Sommergruber, Gaiswinkler, Wiesinger, Puchner and Tews2003; Depa-Martynow et al. , Reference Depa-Martynow, Jedrzejczak and Pawelczyk2007). Cytoplasmic halos are formed by microtubule mediated transport of organelles from the oocyte periphery (Depa-Martynow et al. , Reference Depa-Martynow, Jedrzejczak and Pawelczyk2007). In our study we observed that only 27% of 3PN oocytes had a partial halo and 73% showed total loss of the cytoplasmic halo, which is a sign of poor zygote quality. Our ultrastructural findings for mitochondria and smooth endoplasmic reticulum were similar to the results found by Bruin et al. (Reference Bruin, Dorland, Spek, Posthuma, Haaften, Looman and Velde2004), who reported age-related negative effects on oocytes (mitochondria vacuolization, increased matrix density, cristae loss and ruptured membranes of the SER). Another interesting finding was for NPBs. The number and distribution of the NPBs have been reported to be important in estimating pronuclear oocyte the quality (Scott et al. , Reference Scott, Alvero, Leondires and Miller2000). We observed more than three, dispersed NPBs in serial sections in all pronuclei; this may be another altered feature of 3PN oocytes and can guide our future studies on pronuclei formation. Ultrastructure findings in 3PN oocytes, such as lipofuscin granule accumulation, absence of cytoplasmic halo, multilamellary body formation and degenerated mitochondria, were considered as signs of poor oocyte quality.
Recent studies have shown that the membrane potential of subplasmalemmal localized mitochondria is higher than for other mitochondria in the cytoplasm of MII stage oocytes and early embryos. Low membrane potential mitochondria are localized around PNs. These high-polarized mitochondria are thought to be responsible for oocyte and preimplantation stage embryo development, sperm penetration, cortical granule exocytosis, but they are not associated with sperm binding to the oolemma (Van Blerkom, Reference Van Blerkom2006; Van Blerkom & Davis, Reference Van Blerkom and Davis2007). In our study, JC-1 staining showed that mitochondria located at the subplasmalemmal region of the 3PN oocytes had high membrane potential, which is consistent with earlier reports and our electron microscopy findings (Van Blerkom, Reference Van Blerkom2006; Van Blerkom and Davis, Reference Van Blerkom and Davis2007).
3PN oocyte formation was probably due to the failure of second polar body extrusion. Polar body extrusion depends on migration of the meiotic spindle from the centre to the cortical region by actin filaments (Ai et al. , Reference Ai, Li, Schatten and Sun2009; Brunet & Verlhac, Reference Brunet and Verlhac2011; Levi et al. , Reference Levi, Kaplan-Kraicer and Shalgi2011; Yi et al. , Reference Yi, Unruh, Deng, Slaughter, Rubinstein and Li2011). Eccentrically located meiotic spindles might have loosened their position and migrated to the oocyte centre, therefore the second polar body could not be extruded, generating the extra pronucleus (3PN) in the ooplasm. For this reason we evaluated cytoskeleton elements such as microtubules and microfilaments, which have regulatory roles on 2PB extrusion. Microfilaments distribute at the oocyte cortex and around centrally localized pronuclei in pronuclear stage oocytes (Kim et al. , Reference Kim, Chung and Day1997, Branzini et al. , Reference Branzini, Lavolpe, Nodar and Rawe2007). In our study, FITC-labelled actin filaments were found only in the cortical region of 3PN oocytes, but not around pronuclei. This finding suggested that actin dynamics of 3PN oocytes might have been impaired.
The microtubule organizing centre is essential for precise cytokinesis and defects in MTOC and can be a cause of cell division abnormalities. The MTOC contains centrosomal proteins such as γ-tubulin and nuclear mitotic apparatus protein (NuMA). Abnormal γ-tubulin aggregation can cause chromosomal anomalies (Manandhar et al. , Reference Manandhar, Schatten and Sutovsky2005; Schatten & Sun Reference Schatten and Sun2009, Reference Schatten and Sun2010). For this reason we evaluated MTOC localization via anti-γ-tubulin antibody staining. Van Blerkom & colleagues (Reference Van Blerkom, Davis, Merriam and Sinclair1995) showed one or two labelled MTOC areas around centrally located PNs at 2PN zygotes. We detected strong γ-tubulin staining in the subplasmalemmal region of 3PN oocytes. This type of positional and quantitative disturbance can be related to the earlier cytokinesis in 3PN oocytes compared with 2PN oocytes, reported in time-lapse studies (Joergensen et al. , Reference Joergensen, Agerholm, Hindkjaer, Bolund, Sunde, Ingerslev and Kirkegaard2014).
Of course, several other factors other than those associated with oocytes, such as sperm diploidy, oocyte duplication or duplication of the spermatozoon in the oocyte, or destruction of the mitotic spindle during ICSI manipulation, should also be considered. Probability of the occurrence of these factors is very low and can be ignored, because standard culture conditions for all oocytes were provided.
We evaluated fine structural morphology, mitochondrial activity and cytoskeletal features of 3PN oocytes after ICSI to confer any association between 3PN formation and mitochondrial membrane potential and cytoskeletal abnormalities. Previous studies have reported that oocytes containing lipofuscin granules in their cytoplasm showed reduced fertilization rates (Otsuki et al. , Reference Otsuki, Nagai and Chiba2007). In our study, lipofuscin granules were observed in all oocytes. We also examined some ultrastructural abnormalities, i.e. lack of cytoplasmic halo, multilamellar body formation, diminished cytoskeletal fine filaments, disrupted γ-tubulin accumulation and degenerated mitochondria, all as features of poor oocyte quality. The mitochondrial membrane potential of intact mitochondria was similar to that of 2PN oocytes. Overall these findings suggest that 3PN formation may be related to poor oocyte ultrastructure and disrupted cytoskeleton but not to mitochondrial activity. Notably, lipofuscin granules, which can be seen with a modulation contrast light microscope, may alert physicians when making changes to stimulation protocols and timing of injections and raise the success rates in patients with 3PN oocytes.
Financial support
This work was supported by Istanbul University, Scientific Research Projects (no. 3287) and by the Scientific Research Projects Coordination Unit of Istanbul University, project no. 45657.
Conflicts of interest
There is no conflict of interest that could be perceived as prejudicing the impartiality of the research reported.
Ethical standards
The study was approved by the Istanbul University Istanbul Faculty of Medicine ethical committee on 22 October 2008. All procedures contributing to this work comply with the ethical standards of the relevant national and institutional committees on human experimentation and with the Helsinki Declaration of 1975, as revised in 2008.