Introduction
Guanidino acetic acid (GAA), also referred to as glycoamine, guanidino acetic acid or guanidinoacetate, is an important metabolic intermediary product in vertebrate animal tissues that plays a key role in energy metabolism as a precursor of creatine (Michiels et al., Reference Michiels, Maertens, Buyse, Lemme, Rademacher, Dierick and De Smet2012; Ostojic et al., Reference Ostojic, Niess, Stojanovic and Obrenovic2013). GAA is formed from arginine and glycine catalysed by the enzyme l-arginine: glycine amidinotransferase and this reaction takes place mainly in the kidney, from where GAA is transported to the liver through blood circulation (Dilger et al., Reference Dilger, Bryant-Angeloni, Payne, Lemme and Parsons2013). In the liver, GAA receives a methyl group from S-adenosylmethionine and is catalysed by N-guanidino acetate methyltransferase to form creatine (Wyss and Kaddurah-Daouk, Reference Wyss and Kaddurah-Daouk2000; Komoto et al., Reference Komoto, Takata, Yamada, Konishi, Ogawa, Gomi, Fujioka and Takusagawa2003). Creatine is an important energy buffer in growing animals v. adults, serving as an energy shuttle where high-energy phosphates are brought to sites of rapid adenosine tri-phosphate (ATP) utilization in cells with high energy demands (Brosnan et al., Reference Brosnan, Wijekoon, Warford-Woolgar, Trottier, Brosnan, Brunton and Bertolo2009). However, de novo synthesis of creatine accounts for only about two-thirds of the requirement for optimal energy metabolism while the remainder must come from exogenous sources. It seems that a lack of creatine would reduce cell performance and finally productive performance in animals, since creatine plays a key role in energy metabolism (Delanghe et al., Reference Delanghe, e Slypere, De Buyzere, Robbrecht, Wieme and Vermeulen1989; Khakran et al., Reference Khakran, Chamani, Foroudi, Sadeghi and Afshar2018).
While the exogenous creatine was added to broilers’ feed in the form of creatine monohydrate, the source was not considered stable during feed manufacturing processes (Baker, Reference Baker2009; Zhang et al., Reference Zhang, Li, Gao, Lin, Wang, Zhu, Gao and Zhou2014). In contrast, GAA is a more suitable feed additive compared with creatine because it is less expensive and more chemically stable than creatine. Recently, the use of GAA as a feed additive in animal diets has gained much interest due to its improvement of meat quality. Wang et al. (Reference Wang, Shi, Shan and Zhang2012) reported that increasing dietary supplementation of GAA could increase the muscle pH value and water holding capacity in growing–finishing pigs. Liu et al. (Reference Liu, Li, Li, Gao, Zhang, Gao and Zhou2015) reported that dietary supplementation with 1.0 g/kg GAA could improve meat quality of finishing pigs via delaying muscle glycolysis. In addition, Dilger et al. (Reference Dilger, Bryant-Angeloni, Payne, Lemme and Parsons2013) reported that GAA was also able to be used as an efficacious replacement for dietary arginine for young chicks. Arginine is the fifth limiting amino acid in maize-soybean diets for poultry (Waguespack et al., Reference Waguespack, Powell, Bidner, Payne and Southern2009), which plays a key role in nitric oxide and protein synthesis, growth and development in vertebrata (Wu and Morris, Reference Wu and Morris1998). Ringel et al. (Reference Ringel, Lemme and Araujo2008) proposed that GAA may improve growth and feed efficiency when it was added into maize-soybean meal diets in broilers, and similar positive effects of supplemented GAA have also been confirmed in broiler performance (Hess et al., Reference Hess, Ringel, Lemme, Araujo, Tserveni-Goussi, Yannakopoulos, Fortomaris, Arsenos and Sossidosu2008; Michiels et al., Reference Michiels, Maertens, Buyse, Lemme, Rademacher, Dierick and De Smet2012; Heger et al., Reference Heger, Zelenka, Machander, de la Cruz, Lešták and Hampel2014). Previous studies suggest that GAA addition to broiler diets are beneficial because it has large amounts of arginine which may be spared (Baker, Reference Baker2009; Waguespack et al., Reference Waguespack, Powell, Bidner, Payne and Southern2009). However, GAA exerted its positive effects on growth performance not only in arginine-deficient diets but also in arginine-sufficient diets (Michiels et al., Reference Michiels, Maertens, Buyse, Lemme, Rademacher, Dierick and De Smet2012). So far, the mechanism of action of GAA supplementation in broilers is still not well known.
The small intestine is an important site of nutrient absorption in animals, and better development of this organ may be beneficial for nutrient absorption (Mekbungwan et al., Reference Mekbungwan, Yamauchi and Thongwittaya2002) and better animal performance (Swiatkiewicz and Hanczakowska, Reference Swiatkiewicz and Hanczakowska2006). After formation or absorption from the small intestine, GAA is converted into creatine via guanidinoacetic methyltransferase and then phosphorylated to phosphocreatine, which is necessary to maintain energy homoeostasis in cells (Wyss and Kaddurah-Daouk, Reference Wyss and Kaddurah-Daouk2000; Ostojic et al., Reference Ostojic, Niess, Stojanovic and Obrenovic2013). Therefore, it was hypothesized that GAA supplementation would be responsible for beneficial effects on small intestine development in arginine-sufficient diet, thereby resulting in better performance of broilers. Thus, the current study aimed at assessing the effects of dietary supplementation of GAA on growth performance, thigh meat quality and development of small intestine in broilers.
Materials and methods
Broilers and management
Before onset of the trial, birds were individually wing-tagged, then distributed randomly to experimental cages. The experimental cages were floored with stretch mesh and were provided with nipple drinkers and trough feeders. The lighting programme was set at natural light. Temperature, relative humidity and other experimental parameters were approved by the Animal Ethics Committee of Anhui Science and Technology University (Fengyang, China). Meanwhile, broilers were vaccinated routinely against infectious bronchitis and Newcastle disease, but no type of medication was administered during the entire experimental period.
Experimental design
In this trial, a total of 360 1-day-old female Partridge-Shank broiler chicks were distributed randomly to four treatment groups of 90 birds each. Partridge-Shank is a breed with relatively smaller live weight, which has a unique taste and is popular among consumers. For detailed information see https://baike.so.com/doc/6305673-6519201.html. For each treatment, six replicates were conducted and 15 broilers were assigned to each experimental pen. During the experiment, broilers had ad libitum access to feed and water, and the feed was offered three times daily at 06.00, 12.00 and 18.00 h, respectively. The nutrient levels of the diet (maize-soybean meal diet) corresponded to the NRC (1994) recommended requirements for broilers (Table 1). Based on dry matter (DM) of the formulated diet, each group received GAA (Guangzhou Rongmu Biotechnology Co., Guangzhou, China) dosages of 0, 0.4, 0.8 and 1.2 g/kg in the diet. The experiment lasted 60 days, and feed intake was recorded daily by weighing feed offered and refused based on the pen level. Before and after the experiment, live weight of all broilers was recorded to calculate the daily body gain weight and feed efficiency. Thirty broilers of each group were selected randomly and slaughtered to determine the thigh meat quality and development of the small intestine of broilers.
Table 1. Ingredient and chemical composition of the basal diet fed to broilers

a Multivitamins, providing vitamin A 52 000 IU, vitamin B1 1.8 mg, vitamin B2 18.0 mg, vitamin B12 25.0 mg, vitamin D3 10 600 IU, vitamin E 2.0 mg, vitamin K3 6.0 mg, nicotinic acid 50.0 mg, folic acid 400.0 mg and d-pantothenic acid 35.0 mg per kg.
b Micronutrients, providing Cu 1.0 g, Fe 3.0 g, Se 0.2 g, Zn 3.4 g, I 0.2 g and Mn 13.4 g per kg.
c Metabolizable energy was estimated according to NRC (1994).
Chemical analysis
The composition and chemical analysis of the diet are presented in Table 1. According to AOAC (2007), diet samples were analysed for DM, crude protein, calcium and phosphorus. Metabolizable energy was estimated according to NRC (1994), while dietary amino acids were analysed according to the method of Llames and Fontaine (Reference Llames and Fontaine1994).
Determination of muscle pH value and colour
Thigh muscles (consisting of the biceps femoris, semitendinosus, semimembranosus and tensor fasciae latae) for analysis of meat quality were excised immediately after slaughter and stored at 4 °C. The muscle pH values of the thigh meat were measured after 45 min by inserting the electrodes of a portable meter (HI8424, Beijing Hanna Instruments Science & Technology Co. Ltd, Beijing, China) into multiple thigh muscles. A colorimeter (Spectrophotometer, CR-10, Minolta, Tokyo, Japan) was used to measure meat colour, in terms of its lightness (L*), redness (a*) and yellowness (b*), perpendicular to the muscle surface.
Determination of water holding capacity
The water holding capacity of the thigh muscles was estimated using drip loss and cooking loss, and drip loss was determined as described by An et al. (Reference An, Leng, Gong, Wang, Li and Wang2017). Thigh muscles were trimmed to 5 × 3 × 1 cm and blotted to remove the surface water. They were placed in air-filled plastic bags, which were fastened to avoid evaporation and hung vertically in a refrigerator at 4 °C for 24 h. Thigh samples were weighed before and after this procedure, and the drip loss was calculated as follows:

Cooking loss was measured following the method described by Liu et al. (Reference Liu, Li, Li, Gao, Zhang, Gao and Zhou2015). Samples from thigh muscles were vacuum-packed in polyethylene vacuum bags then cooked by immersion in a water bath at 70 °C for 45 min, followed by cooling in cold-running tap water to room temperature and then patted dry. The differences between initial and final weights were calculated as cooking loss (g/100 g).
Texture profile analysis
The texture of the cooked thigh muscle was determined according to both the recommended Texture Profile Analysis tests guidelines (Overview of Texture Profile Analysis, http://texturetechnologies.com/resources/texture-profile-analysis) and the method described by Naji et al. (Reference Naji, Amadou, Abbas, Zhao, Shi and Le2013). Meat samples were homogenized through 6 mm plates, then chicken thigh meat patties (5 cm diameter, 2 cm thickness and 30 g weight) were prepared. Half of each thigh patty was cooked to an internal temperature of 75 °C to prepare the cooked meat samples. The centres of the meat samples were compressed twice to 0.90 of their original height with a texture analyser (Model A-XT2, Stable Micro Systems, Surrey, UK) attached to a needle (15 mm external diameter) at a test speed of 1.00 mm/s and a trigger force of 0.001 kg, and the holding time between compressions was 5 s.
In the present trial, hardness was expressed as the peak force of the first compression, cohesiveness was expressed as the area of work during the second compression divided by the area of work during the first compression, and springiness was measured by the distance of the detected height during the second compression divided by the original compression distance. Gumminess and chewiness were calculated as follows:


Estimation of tenderness
In the current trial, tenderness of the thigh muscles was estimated using shear force value and was determined as described by An et al. (Reference An, Leng, Gong, Wang, Li and Wang2017). Before measurement, the thigh meat was trimmed to 3.0 × 1.0 × 0.5 cm, parallel to the muscle fibre. Then the thigh meat was sheared perpendicularly to the muscle fibre at a head speed of 200 mm/min with a digital muscle tenderness meter (C-LM3B, Northeast Agricultural University, Haerbin, China), and the maximal force required to shear the cores was recorded in Newton (N).
Intestine histological analysis
After determination of weight and length, small intestine samples were immediately spread as follows from: (1) the apex of the duodenum, (2) midway between the point of entry of the bile ducts and Meckel's diverticulum (jejunum) and (3) 10 cm proximal to the caecal junction (ileum). The removed tissues were fixed with 10% buffered formalin, then stained with haematoxylin and eosin and embedded in paraffin. The villus height (from the tip of the villi to the villus crypt junction) and crypt depth (defined as the depth of the invagination between adjacent villi) were evaluated under a light microscope. Data were acquired with a Carl Zeiss Axioskop microscope (Carl Zeiss GmbH, Jena, Germany) and a ZVS-47DE CDD camera (Optronics Inc., Goleta, USA) connected by an RGB line to a GraBIT PCI graphic card (Soft Imaging System GmbH, Munster, Germany) installed in a standard PC computer.
For determination of thigh muscle fibre diameter, the thigh muscles were cut into 5-μm thick sections using a Leica microtome (Nussloch, Germany), and stained with haematoxylin and eosin. The fibre diameter of thigh muscle was assessed under a light microscope (BX51, Olympus Japan Inc., Tokyo, Japan) using computer-based Image Pro Plus 6.0 software (Media Cybernetic, Silver Springs, MD, USA).
Statistical analysis
At the conclusion of each growth assay, broilers and feeders were weighed, and live weight gain, feed intake and feed efficiency (i.e. gain-to-feed ratio) were calculated based on each replicate pen of chicks.
Other data of the current experiment were also analysed with PROC MIXED statement of SAS 9.4 (Statistical Analysis for Windows, SAS Institute Inc., Cary, NC, USA). Linear and quadratic effects of treatments indicated by orthogonal contrasts were used to evaluate effects of GAA supplementation. Duncan's multiple range test was conducted to determine the significance level of comparison between treatment means, and difference was considered significant at P ⩽ 0.05. The model including random and fixed effects was as follows:

where Y ij is the dependent variable, μ is the overall mean, B i is the random effect of broilers (i = 30), G j is the fixed effect of the GAA supplementation (j = 0, 0.4, 0.8 and 1.2 g/kg) and ε ij is the error term.
Results
Effects of GAA on growth performance of broilers
Increasing dietary supplementation of GAA quadratically increased final live weight (P = 0.006) and daily body weight gain (P = 0.006) and linearly increased gain-to-feed ratio (P < 0.001) of broilers, but linearly decreased daily feed intake of broilers (P = 0.003) (Table 2).
Table 2. Effects of dietary supplementation of GAA on growth performance of 60-day-old broilers

Results are means of six pens corresponding to 90 broilers for each treatment.
a Contrast, supplemental effect of GAA; linear, linear effect of GAA; quadratic, quadratic effect of GAA.
Effects of GAA on thigh meat quality of broilers
Increasing dietary supplementation of GAA quadratically increased muscle pH values (P = 0.012) and fibre diameter (P = 0.036), but decreased drip loss (P = 0.045) and cooking loss (P = 0.002) as well as shear force value (P = 0.027) of thigh muscles (Table 3). Supplementation of GAA in broilers diet did not improve thigh muscle colour including brightness, redness and yellowness.
Table 3. Effects of dietary supplementation of GAA on thigh meat quality of 60-day-old broilers

Results are means of six pens corresponding to 30 broilers for each treatment.
a Contrast, supplemental effect of GAA; linear, linear effect of GAA; quadratic, quadratic effect of GAA.
Increasing dietary supplementation of GAA quadratically decreased hardness (P = 0.002), gumminess (P = 0.039) and chewiness (P < 0.001) of thigh meat but did not influence neither cohesiveness nor springiness (Table 4).
Table 4. Effects of dietary supplementation of GAA on texture of cooked thigh meat of 60-day-old broilers

Results are means of six pens corresponding to 30 broilers for each treatment.
a Contrast, supplemental effect of GAA; linear, linear effect of GAA; quadratic, quadratic effect of GAA.
Effects of GAA on development of small intestine of broilers
Increasing dietary supplementation of GAA (Table 5) quadratically increased duodenal weight index (P = 0.019), villus height (P = 0.039) and width (P = 0.010) and ratio of duodenal villus height to crypt depth (P < 0.001) but decreased duodenal crypt depth (P < 0.001).
Table 5. Effects of dietary supplementation of GAA on development of duodenum of 60-day-old broilers
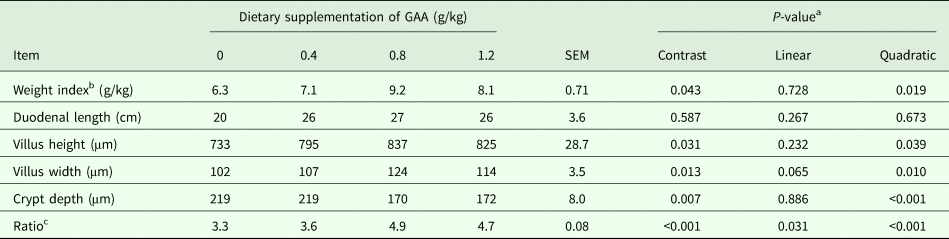
Results are means of six pens corresponding to 30 broilers for each treatment.
a Contrast, supplemental effect of GAA; linear, linear effect of GAA; quadratic, quadratic effect of GAA.
b Weight index was calculated as: weight index (g/kg) = duodenal weight (g)/live weight (kg).
c Ratio of duodenal villus height to crypt depth.
Increasing dietary supplementation of GAA (Tables 6 and 7) quadratically increased both jejunal (P = 0.042) and ileal (P = 0.020) villus height, jejunal (P = 0.032) and ileal (P = 0.024) villus width and ratio of villus height to crypt depth (P < 0.001), but decreased jejunal and ileal crypt depth (P < 0.001).
Table 6. Effects of dietary supplementation of GAA on development of jejunum of 60-day-old broilers

Results are means of six pens corresponding to 30 broilers for each treatment.
a Contrast, supplemental effect of GAA; linear, linear effect of GAA; quadratic, quadratic effect of GAA.
b Weight index was calculated as: weight index (g/kg) = jejunal weight (g)/live weight (kg).
c Ratio of jejunal villus height to crypt depth.
Table 7. Effects of dietary supplementation of GAA on development of ileum of 60-day-old broilers

Results are means of six pens corresponding to 30 broilers for each treatment.
a Contrast, supplemental effect of GAA; linear, linear effect of GAA; quadratic, quadratic effect of GAA.
b Weight index was calculated as: weight index (g/kg) = ileal weight (g)/live weight (kg).
c Ratio of ileal villus height to crypt depth.
Discussion
GAA is a more suitable feed additive compared with creatine and arginine because it is less expensive than either of those compounds and is more chemically stable than creatine (Dilger et al., Reference Dilger, Bryant-Angeloni, Payne, Lemme and Parsons2013). In addition, evidence suggests that GAA may have growth and feed efficiency promoting properties when added to practical maize-soybean meal diets (Ringel et al., Reference Ringel, Lemme and Araujo2008). In the current study, growth performance and development of the small intestine were used as sensitive indicators of nutritional absorption and utilization efficiency to demonstrate the improving effects of GAA in maize-soybean meal diet for broilers, which may extend understanding of the possible reason of dietary supplementation of GAA for feed efficiency v. weight gain of broilers.
Growth performance of broiler response to dietary supplementation of GAA
The current study showed that GAA-supplemented broilers refused greater amounts of rations than GAA un-supplemented broilers, and this was in agreement with the previous research of Mousavi et al. (Reference Mousavi, Afsar and Lotfollahian2013), who reported that GAA reduced feed intake of broilers from 23 to 40 days of age. Similar reduction of feed intake was also observed in turkeys over the entire 21-week experimental period with increasing GAA inclusion levels (Lemme et al., Reference Lemme, Gobbi, Helmbrecht, Van Der Klis, Firman, Jankowski and Kozlowsk2010). In the current study, decreased feed intake in GAA-supplemented broilers may be explained in two ways: firstly, since the GAA was added to the feed, it may have modified the taste and thus might have resulted in greater refusals for the GAA-supplemented broilers. Another reason could be that GAA is an energy source and may increase dietary energy concentration. Lopez and Leeson (Reference Lopez and Leeson2008) reported that broilers display an exceptional ability to control their energy intake by regulating their feed intake when diet energy concentration changes. Thus, increasing dietary supplementation of GAA would result in reduced feed intake of broilers.
The small intestine is one of the most important sites of nutrient absorption and better development of this organ may be beneficial for nutrient absorption (Mekbungwan et al., Reference Mekbungwan, Yamauchi and Thongwittaya2002) as well as better performance in animals (Swiatkiewicz and Hanczakowska, Reference Swiatkiewicz and Hanczakowska2006). In the present trial, both the height and width of intestinal villi in GAA-supplemented broilers were increased quadratically, which would be beneficial for nutrient absorption, thus resulting in greater growth performance of broilers. In agreement with Lemme et al. (Reference Lemme, Ringel, Rostagno and Redshaw2007a, Reference Lemme, Ringel, Sterk and Young2007b) current results found that feeding GAA may improve the feed conversion ratio and weight gain of broilers. Despite this, inferior growth performance was still seen in the present trial compared with modern chicks (i.e. commercial chickens with higher growth rates). Commonly, modern chickens will achieve weights of at least 2000 g by an age of 35 days or less. However, the present average live weights were less than 2000 g after 60 days’ growth, and this may be related to the genetics of the broilers (female Partridge-Shank) used in the present trial.
Lemme et al. (Reference Lemme, Ringel, Rostagno and Redshaw2007a) reported that the optimum supplemental concentration of GAA ranged from 0.6 to 1.2 g/kg DM of feed, whereas 6.0 g/kg GAA would reduce feed consumption and consequently weight gain (Tossenberger et al., Reference Tossenberger, Rademacher, Németh, Halas and Lemme2016). These reasons may account for the quadratic effect of GAA supplementation, and the present trial indicated that the optimum supplemental concentration of GAA was 0.8 g/kg DM of feed for broilers.
Meat quality of broiler response to dietary supplementation of GAA
Meat quality is affected by a number of factors including genetic, environment, feed, slaughtering conditions, processing and storage of meat and feed additives have been reported as an important factor to influence physical and chemical characteristics as well as sensory and microbial quality of meat (Nasir and Grashorn, Reference Nasir and Grashorn2010). In the current study, GAA-supplemented broilers had greater thigh muscle pH values, and this may be due to inhibition effects of GAA on activity of hexokinase. It is well known that hexokinase is one of the rate-limiting enzymes in glycolysis reaction, which catalyses the conversion of glucose to glucose-6-phosphate and finally results in accumulation of lactic acid (Scheffler and Gerrard, Reference Scheffler and Gerrard2007). After slaughter, as muscle is converted to meat, the oxygen in the muscles is gradually exhausted and anaerobic glycolysis occurs, which favours the production of lactic acid, resulting in declining tissue pH (Bertol et al., Reference Bertol, Ellis, Ritter and McKeith2005). However, dietary supplementation of GAA in the period pre-slaughter may reduce the activity of hexokinase and production of lactic acid (Liu et al., Reference Liu, Li, Li, Gao, Zhang, Gao and Zhou2015), therefore maintaining pH value of thigh muscles post mortem in GAA-supplemented broilers.
In the current study, GAA-supplemented broilers had greater water-holding capacity of thigh muscles, which may be associated with GAA-induced enhancement of muscle pH. The ability of fresh meat to retain moisture is arguably one of the most important quality characteristics of raw products, but this water-holding capacity of muscle is usually affected by alteration of cell structure and lowering of muscle pH (Offer and Knight, Reference Offer, Knight and Lawrie1988). Huff-Lonergan and Lonergan (Reference Huff-Lonergan and Lonergan2005) noted that once the muscle pH has reached the isoelectric point of the major proteins, especially myosin, the net charge of the protein becomes zero, meaning the numbers of negative and positive charges on the proteins are essentially equal. These negative and positive groups within the protein are attracted to each other, resulting in a reduction in the amount of water that can be attracted and held by that protein. Additionally, pH-induced lateral shrinkage of the myofibril may also lead to expulsion of water from the myofibrillar structure into the extra-myofibrillar spaces and ultimately out of the muscle (Bendall and Swatland, Reference Bendall and Swatland1988). The greater muscle pH value found in the current work v. improved water-holding capacity was enhanced by GAA supplementation, which is consistent with the results of both Wang et al. (Reference Wang, Shi, Shan and Zhang2012) and Liu et al. (Reference Liu, Li, Li, Gao, Zhang, Gao and Zhou2015), who reported that dietary supplementation of GAA for finishing pigs could increase meat pH value and improve muscle water-holding capacity.
Meat colour is an important parameter of meat quality characteristics, and it influences meat purchasing decisions more than any other quality factor because consumers use discolouration as an indicator of freshness and wholesomeness. In the present trial, dietary supplementation of GAA did not improve thigh muscle colour, which is in line with observations by Liu et al. (Reference Liu, Li, Li, Gao, Zhang, Gao and Zhou2015). As with meat colour, meat tenderness also has a large impact on consumers’ satisfaction with chicken meat. In the current study, increasing dietary supplementation of GAA may reduce shear force value of thigh muscles, which may be due to improved cell energy metabolism since supplemental GAA could increase the content of ATP (Liu et al., Reference Liu, Li, Li, Gao, Zhang, Gao and Zhou2015). As muscle is converted to meat, the ATP in the muscles is gradually exhausted and irreversible cross-bridge formation between myosin heads and actin, as well as the occurrence of rigor mortis (Bowker et al., Reference Bowker, Grant, Forrest and Gerrard2000). In contrast, increased ATP provided indirectly by dietary supplementation of GAA may provide sufficient energy for muscle contraction, which could result in excessive insertion of thin myofilaments mainly consisting of actin (Michiels et al., Reference Michiels, Maertens, Buyse, Lemme, Rademacher, Dierick and De Smet2012). Ultimately, shear force value of thigh muscles would be reduced significantly.
Texture profile analysis uses a double compression cycle to simulate the first and second bites, similar to a human subject, may provide insights into how the meat behaves when chewed. The current study found that dietary supplementation of GAA may lead to a decline in hardness, gumminess and chewiness in cooked thigh meat of GAA-supplemented broilers, which may be associated with GAA-induced improvement of water-holding capacity in thigh muscles. Battula et al. (Reference Battula, Schilling, Vizzier-Thaxton, Behrends, Williams and Schmidt2008) reported that higher muscle water-holding capacity could reduce liquid outflow, loss of soluble nutrients and flavour, therefore the meat would not become hard and tasteless. In view of meat quality response to GAA, the present trial indicated that increasing dietary supplementation of GAA was of benefit for improvement of meat quality in boilers.
Small intestine development of broiler response to dietary supplementation of GAA
In the present trial, broilers fed a diet with higher GAA were observed to have better development of the small intestine, particularly greater height and width of intestinal villi, and this may be due to the stimulating effects of GAA on the intestinal barrier function. Generally, intestinal villus cells are held together by tight and adherens junctions in the apical junctional complex, structures that are in close contact with the F-actin filaments of the cytoskeleton, and energy provision is very important for their maintenance (Ivanov et al., Reference Ivanov, Parkos and Nusrat2010). It is well known that the gastrointestinal tract is a metabolically active system that requires a large amount of oxygen (Yen et al., Reference Yen, Nienaber, Hill and Pond1989), and the intestinal epithelial cells will be exposed to a hypoxic environment in inflammatory states (Brosnan and Brosnan, Reference Brosnan and Brosnan2016). After absorption from the small intestine, GAA provided in the diet will be converted into creatine, which is important to promote intestinal epithelial restitution and ameliorate mucosal inflammation via enhanced cellular energetics of intestinal epithelial cells (Glover et al., Reference Glover, Bowers, Saeedi, Ehrentraut, Campbell, Bayless, Dobrinskikh, Kendrick, Kelly, Burgess, Miller, Kominsky, Jedlicka and Colgan2013). In addition, dietary supplementation of GAA may improve cell energy metabolism not only via increasing content of creatine but also phosphocreatine and ATP, and these increasing metabolites may improve energy utilization for cells accretion and thus protein yield (Lemme et al., Reference Lemme, Ringel, Sterk and Young2007b; Ringel et al., Reference Ringel, Lemme and Araujo2008). The present results are consistent with the report of Kodambashi Emami et al. (Reference Kodambashi Emami, Golian, Rhoads and Danesh Mesgaran2017), who reported that dietary supplementation of GAA for male broilers could improve jejunal villus surface area via increasing villus height and width under cold temperature.
Previous studies have suggested that GAA may spare arginine and help protein synthesis, which finally increases meat yield (Baker, Reference Baker2009; Waguespack et al., Reference Waguespack, Powell, Bidner, Payne and Southern2009). In the present trial, despite the basal diet meeting arginine requirements (12.5 g/kg) of broilers recommended by NRC (1994), increasing dietary supplementation of GAA may still improve feed efficiency versus weight gain of broilers. The present result, that GAA could also exert positive effects on growth performance of broilers in arginine-sufficient diets, is consistent with Michiels et al. (Reference Michiels, Maertens, Buyse, Lemme, Rademacher, Dierick and De Smet2012). Taking important absorption function of small intestine into consideration, the present results indicate that the positive effects of GAA supplementation on performance of broilers may be mainly derived from its stimulating function on better development of the small intestine.
Conclusions
The present experiment has shown that better development of the small intestine of broilers can be achieved by increasing dietary supplementation of GAA. Achieving this, increasing dietary supplementation of GAA may improve both growth performance and meat quality of broilers.
Author ORCIDs
Q. C. Ren, 0000-0002-8885-286X
Acknowledgements
The authors appreciate the financial support from the Natural Science Foundation of Anhui Provincial Education Department (no. KJ2016SD15) and the Scientific Research Foundation of Anhui Science and Technology University (no. 1409).
Conflict of interest
There were no conflicts of interest in the current study.
Ethical standards
This study was approved by the Ethics Committee of Anhui Science and Technology University (ECASTU-2015-P08).