Introduction
Savanna is an heterogeneous vegetation in the tropics and subtropics, usually characterised by a continuous C4 herbaceous layer with a discontinuous C3 tree stratum, where fire is a prevalent endogenous disturbance (Lehmann et al. Reference Lehmann, Archibald, Hoffmann and Bond2011, Reference Lehmann, Anderson, Sankaran, Higgins, Archibald, Hoffmann, Hanan, Williams, Fensham, Felfili, Hutley, Ratnam, San Jose, Montes, Franklin, Russell-Smith, Ryan, Durigan, Hiernaux, Haidar, Bowman and Bond2014, Pausas and Bond, Reference Pausas and Bond2020). Tropical savannas are distributed in Africa, Australia and South America (Figure 1a) under different fire regimes and dynamics, covering approximately 20% of the terrestrial surface (Archibald et al. Reference Archibald, Lehmann, Gómez-Dans and Bradstock2013, Reference Archibald, Bond, Hoffmann, Lehmann, Staver, Stevens, Scogings and Sankaran2019, Lehmann et al. Reference Lehmann, Archibald, Hoffmann and Bond2011). In South America, savannas are mainly distributed in the Cerrado (Borghetti et al. Reference Borghetti, Barbosa, Ribeiro, Ribeiro, Walter, Scogings and Sankaran2019, Critical Ecosystem Partner Fund, 2018, Lehmann et al. Reference Lehmann, Archibald, Hoffmann and Bond2011, Olson et al. Reference Olson, Dinerstein, Wikramanayake, Burgess, Powell, Underwood, D’amico, Itoua, Strand, Morrison, Loucks, Allnutt, Ricketts, Kura, Lamoreux, Wettengel, Hedao and Kassem2001), a phytogeographic domain that covers approximately 2 million km2 (Figure 1b). The Cerrado harbours 12,363 species of angiosperm in Brazil; more than half of this diversity (6,939 species) belongs to savannas (cerrado sensu lato vegetation), which corresponds to approximately 95% of angiosperm species in the Brazilian savannas and includes over 1,000 species of trees (Flora and Funga of Brazil, 2022).
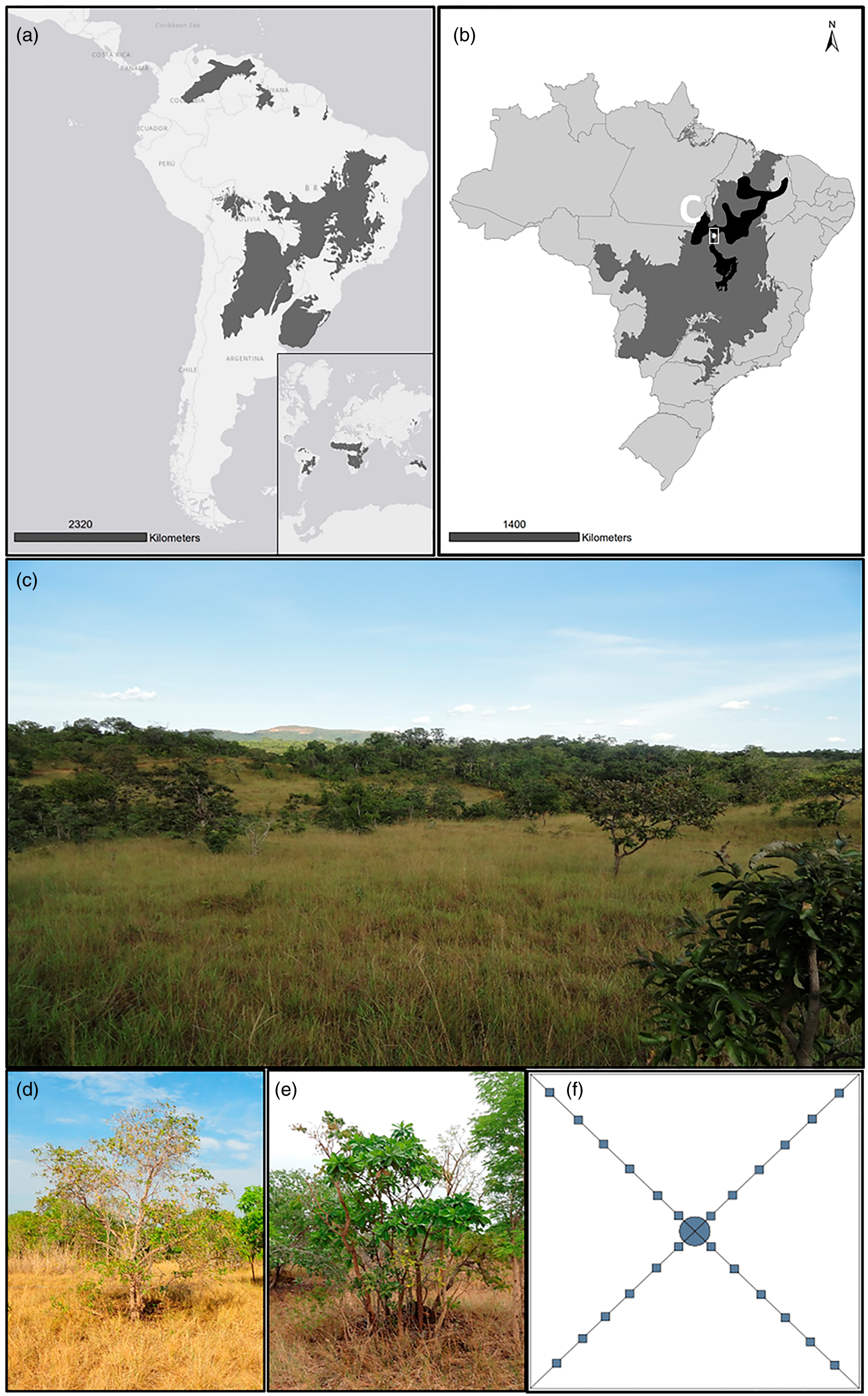
Figure 1. Study area, vegetation, sample design and target plants. (a) Savanna biome area (dark grey) with emphasis on South America (WWF 2019). (b) Cerrado domain area (dark grey; IBGE 2019) and Cerrado Biosphere Reserve (black; MMA, 2019) in Brazil, showing the study area. (c) Landscape in the study area. (d) Hancornia speciosa. (e) Himatanthus obovatus. (f) Scheme of sample plots: – plot limits;
– target species;
– subplots.
More than half of the native vegetation cover in the Cerrado domain is already converted to croplands, mainly of soybean, and pasturelands or has been changed to hydroelectric dams, urban areas or mining. Most of the remaining area (>80%) is not covered by pristine vegetation and remains under intense anthropic pressure, mainly because of the agribusiness expansion (INPE, 2019, Klink and Machado, Reference Klink and Machado2005, Lahsen et al. Reference Lahsen, Bustamante and Dalla-Nora2016, Overbeck et al. Reference Overbeck, Vélez-Martin, Menezes, Anand, Baeza, Carlucci, Dechoum, Durigan, Fidelis, Guido, Moro, Munhoz, Reginato, Rodrigues, Rosenfield, Sampaio, Barbosa da Silva, Silveira, Sosinski, Staude, Temperton, Turchetto, Veldman, Viana, Zappi and Müller2022, Strassburg et al. Reference Strassburg, Brooks, Feltran-Barbieri, Iribarrem, Crouzeilles, Loyola, Latawiec, Oliveira Filho, Scaramuzza, Scarano, Soares-filho and Balmford2017). Conservation units protect less than 10% of the Cerrado (ICMBio, 2019, Lahsen et al. Reference Lahsen, Bustamante and Dalla-Nora2016), which will not be effective in the face of current land use and the effects of climate change (Velazco et al. Reference Velazco, Villalobos, Galvão and De Marco Júnior2019). Over 30% of the remaining Cerrado is estimated to be devastated by 2050, leading to the extinction of hundreds of plant species (Strassburg et al. Reference Strassburg, Brooks, Feltran-Barbieri, Iribarrem, Crouzeilles, Loyola, Latawiec, Oliveira Filho, Scaramuzza, Scarano, Soares-filho and Balmford2017). In the last two or three decades, the Cerrado agricultural frontiers have comprised mainly savannas in the states of Maranhão (MA), Tocantins (TO), Piauí (PI) and Bahia (BA), a region known as MATOPIBA (Pivello et al. Reference Pivello, Vieira, Christianini, Ribeiro, Menezese, Berlinck, Melog, Marengo, Tornquist, Tomas and Overbeck2021, Sano et al. Reference Sano, Rosa, Scaramuzza, Adami, Bolfe, Coutinho, Esquerdo, Maurano, Narvaes, Oliveira Filho, Silva, Victoria, Ferreira, Brito, Bayma, Oliveira and Bayma-Silva2019). This region comprises the most significant remnants of original vegetation in the Cerrado, but its flora remains little known (Santana and Simon, Reference Santana and Simon2022).
The boundaries between fire-prone savannas and fire-impending forests can be sharp and dynamic. Under intermediate rainfall and mild seasonality, similar resource conditions can support contrasting fire feedback loops and lead to the establishment of either savannas or forests as alternative stable vegetation types (‘biomes’ in Staver et al. Reference Staver, Archibald and Levin2011 or ‘ecosystems’ in Buisson et al. Reference Buisson, Le Stradic, Silveira, Durigan, Overbeck, Fidelis, Fernandes, Bond, Hermann, Mahy, Alvarado, Zaloumis and Veldman2019, see also Bond et al. Reference Bond, Bradley and Lee2016, Dantas et al. Reference Dantas, Hirota, Oliveira and Pausas2016, Le Stradic et al. Reference Le Stradic, Roumet, Durigan, Cancian and Fidelis2021, Midgley et al. Reference Midgley, Lawes and Chamaillé-Jammes2010, Pausas and Bond, Reference Pausas and Bond2020, Reis et al. Reference Reis, Lenza, Marimon, Gomes, Forsthofer, Morandi, Marimon Junior, Feldpausch and Elias2015, Touboul et al. Reference Touboul, Staver and Levin2018, Veldman et al. Reference Veldman, Buisson, Durigan, Fernandes, Le Stradic, Mahy, Negreiros, Overbeck, Veldman, Zaloumis, Putz and Bond2015). A large portion of South America is estimated to be under bi-stability, showing mainly savannas (low tree cover) in the Brazilian Cerrado domain and especially forests (high tree cover) in the eastern Amazonia domain (Staver et al. Reference Staver, Archibald and Levin2011). On a continental scale, the savanna vegetation is often reduced to a couple of functional groups, namely graminoid (mainly C4 grasses) and woody plants (mainly C3 trees). Hence, local patterns and processes that characterise heterogeneous landscapes are usually neglected (e.g. Levick and Rodgers, Reference Levick and Rogers2011), and the impact of interactions within life forms in structuring and maintaining the plant community assemblage is overlooked.
Recruitment is critical to savanna structure and dynamics (e.g. Borghetti et al. Reference Borghetti, Barbosa, Ribeiro, Ribeiro, Walter, Scogings and Sankaran2019), but this process has yet to be further investigated (Midgley et al. Reference Midgley, Lawes and Chamaillé-Jammes2010). Fire selectively affects recruitment more than established trees: it helps to maintain a low tree cover and favours the recruitment and persistence of savanna specialists to the detriment of forest specialists (Midgley et al. Reference Midgley, Lawes and Chamaillé-Jammes2010). Plant–plant interactions, such as competition and facilitation, within life forms can also be essential for recruitment, community assembling and vegetation structuring (Tatsumi et al. Reference Tatsumi, Cadotte and Mori2019, Tilman, Reference Tilman2004, Vega-Álvarez et al. Reference Vega-Álvarez, García-Rodríguez and Cayuela2019). However, their influence in savannas remains poorly explored and discussed (see Chagas et al. Reference Chagas, Rapini and Collevatti2020, Flake et al. Reference Flake, Honda, Pilon, Hoffmann and Durigan2022, Le Stradic et al. Reference Le Stradic, Roumet, Durigan, Cancian and Fidelis2021). Here, we evaluated the influence of tree-on-tree interactions and the altitude effect (abiotic conditions) on tree recruitment in a savanna within the MATOPIBA region in Central Brazil.
First, we compare the species number, abundance and composition of juvenile and adult trees in woody communities associated with two species of non-forest trees that are commonly found in savannas: Hancornia speciosa Gomes and Himatanthus obovatus (Müll. Arg.) Woodson (Apocynaceae). While H. speciosa seems to interact negatively with the neighbourhood occupation, H. obovatus seems to interact positively. The contrasting effects of these target species in the associated plant community were inferred from field observations and supported through laboratory tests on allelopathy (Gonçalves, Reference Gonçalves2016, Uhlmann et al. Reference Uhlmann, Oliveira and Santos2018). More recently, the number of rare species surrounding these trees also supported their opposite influence on the selectivity of coexisting species. In particular, H. speciosa acted as a filter, reducing the richness and abundance of surrounding trees while promoting evenness in the associated tree communities (Chagas et al. Reference Chagas, Rapini and Collevatti2020). By comparing juvenile and adult trees associated with these species, we aim to identify patterns of species turnover and changes in the community structure, providing evidence of the regional species pool and the potential factors involved in tree recruitment (Datry et al. Reference Datry, Bonada and Heino2015, Grime, Reference Grime1998, Salles and Schiavini, Reference Salles and Schiavini2007). Finally, we assess the relative importance of tree-on-tree interactions and abiotic conditions (on a local scale, embodied by the altitude) on the structure and diversity of woody communities.
Variation in the species number is a key parameter for assessing environmental restoration efforts. Still, it is equally important to consider species composition and abundance, as they impact the functioning and structuring of plant communities (Chagas and Pelicice, Reference Chagas and Pelicice2018, Grubb, Reference Grubb1977, Pausas and Bond, Reference Pausas and Bond2021, Ploughe et al. Reference Ploughe, Jacobs, Frank, Greenler, Smith and Dukes2019). Both negative and positive interactions can influence species composition, increase species richness and decrease population size, albeit in different strata (Hutchinson, Reference Hutchinson1967, Tilman, Reference Tilman2004, Vega-Álvarez et al. Reference Vega-Álvarez, García-Rodríguez and Cayuela2019, Whittaker, Reference Whittaker1969). Given the biotic filter imposed by H. speciosa and H. obovatus, we anticipate distinct patterns of tree recruitment in their surrounding compared to communities not associated with them. Consequently, we hypothesise that plant interactions will play a more significant role than altitude in shaping adult communities, whereas their influence on juvenile communities may be less pronounced.
Materials and methods
Study area
This study was conducted in a savanna of Central Brazil, in Porto Nacional, state of Tocantins, in 2016. The study area is in the Cerrado Biosphere Reserve (Figures 1b and 1c), delimited by the Man and the Biosphere programme of the United Nations Educational, Scientific and Cultural Organization (Brito and Silva Reference Brito and Silva2019). Savanna plots were set up in three reserves of private rural properties with low anthropic activity, classified as cerrado sensu stricto (Ribeiro and Walter, Reference Ribeiro, Walter, Sano, Almeida and Ribeiro2008) and natural occurrences of Hancornia speciosa and Himatanthus obovatus. The reserves encompass ∼ 109, 40.5 and 14.5 ha, forming a polygon of ∼ 8,500 ha between 10º33′00″–10º48″30″S and 48º20′00″–48º26′00″W, with plots distributed in polygons of ∼ 23.5, 33.5 and 5 ha, respectively. Soils in the three reserves are oligotrophic and acidic, with low organic matter and high levels of aluminium (Pinheiro et al. Reference Pinheiro, Coimbra, Silva and Ferreira2018). Microenvironmental differences within and between sites were homogenised by the use of replicas at local and regional scales. However, abiotic factors, such as temperature, are not expected to vary enough locally to affect our results significantly. Although often seen as a prime determinant of savannas, soil properties are extremely uniform below 10–15 cm and are not associated with changes in the Cerrado composition and physiognomy (Ruggiero et al. Reference Ruggiero, Batalha, Pivello and Meirelles2002). Fire was recorded in these three reserves in the years 2004, 2007, 2008, 2010, 2011, 2012, 2014, 2017 and 2019 (Chagas and Pelicice, Reference Chagas and Pelicice2018; INPE, 2019).
Target species
Hancornia speciosa (Figure 1d), popularly known as ‘mangabeira’, produces fruits (‘mangabas’) that are used by the locals and small industries for candies, juices and jams. It is also a source of latex for traditional medicine. The species blooms from September to November, with fruit dispersal only after riped and fallen, from November to January. In Brazil, H. speciosa occurs in the Amazon, Caatinga, Atlantic Forest and Cerrado phytogeographic domains, reaching Paraguay, Bolivia, Peru and Venezuela (Almeida et al. Reference Almeida, Nogueira, Borges, Prado and Gonçalves2016, Collevatti et al. Reference Collevatti, Rodrigues, Vitorino, Lima-Ribeiro, Chaves and Telles2018, Flora and Funga of Brazil, 2022, Monachino, Reference Monachino1945).
Himatanthus obovatus (Figure 1e) is popularly known as ‘Tiborna’. The plant is used in folk medicine as an antitumour agent and has immunomodulatory properties. It blooms throughout the year, with a peak from October to December, and the seeds are wind dispersed. The species is also used for reforestation because of its rapid growth. In Brazil, H. obovatus occurs in the Amazon, Caatinga and Cerrado phytogeographic domains, reaching Bolivia (Flora and Funga of Brazil, 2022, Plumel, Reference Plumel1991, Soares et al. Reference Soares, Cavalcante, Romero and Bandeira2016).
Sampling
In each of the three reserves, 30 plots of 10 × 10 m were set up (Figure 1f): ten plots with an adult individual of H. speciosa in the centre (Hancornia plots), ten with an adult individual of H. obovatus (Himatanthus plots) and ten without any of the target species (Control plots), but with a wood species of similar height in the centre of the plot. Plots were at least 10 m distant from each other, and plots of the same type (Hancornia, Himatanthus or Control) had a minimum distance of 20 m. A map showing the plot distribution is available in Chagas et al. (Reference Chagas, Rapini and Collevatti2020), and the coordinates and altitude of the plots are available in Supplementary Information S1. To quantify adult trees, in each plot, we collected all plants with stem perimeter at ground ≥10 cm (3.18 cm of diameter) or height ≥1 m (Felfili et al. Reference Felfili, Rezende, Silva and Silva2000), including dead individuals. Frames of 0.5 × 0.5 m were used to quantify seedlings and saplings (juveniles hereafter), including individuals <10 cm in circumference and <1 m in height (Mueller-Dombois and Ellenberg, Reference Mueller-Dombois and Ellenberg1974). The frames were distributed within the plots, from the centre to the angles of the plot, at 1 m far from each other, comprising four transects with six frames, totalling 24 frames per plot (Figure 1f). We recorded all woody species within each subplot (Felfili et al. Reference Felfili, Rezende, Silva and Silva2000) and counted the number of individuals for each species. The vouchers are deposited in the herbarium of Universidade Estadual de Feira de Santana (HUEFS), with duplicates sent to the herbarium of Universidade Federal do Tocantins (HTO). The species were identified in the least inclusive taxonomic categories and classified according to the APG IV (2016).
Data analysis
For each type of plot (Hancornia, Himatanthus or Control), we calculated species richness (number of species; Tokeshi, Reference Tokeshi1993) and abundance (number of individuals; Magurran, Reference Magurran2004). To standardise species richness, we calculated rarefaction curves based on plot sampling effort and 500 randomisations of the original matrix of each plant stage (juvenile and adult trees) separately, using the Jackknife1 estimator implemented in the package EstimateSWin910 (Colwell, Reference Colwell2017). For adult trees, we used the rarefaction curves of Chagas et al. (Reference Chagas, Rapini and Collevatti2020). We also evaluated the species richness extrapolated to twice the sampling effort using the package iNEXT (iNterpolation and EXTrapolation; Chao et al. Reference Chao, Gotelli, Hsieh, Sander, Ma, Colwell and Ellison2014). The relative abundance was calculated for each species (Mueller-Dombois and Ellenberg, Reference Mueller-Dombois and Ellenberg1974). We tested the data distribution using the Shapiro–Wilk test to corroborate the normality assumption (Crawley, Reference Crawley2013). We then used the Kruskal–Wallis H test (non-parametric test for non-normally distributed data) to compare the median and interquartile range of measures among plot types and plant stages (p ≤ 0.05). We applied a posteriori Dunn’s test to compare the median using Bonferroni adjustment to control the error rate (Dinno, Reference Dinno2017). The analyses were performed in R3.4.4 (R Core Team, 2019).
Generalised linear models were used to test the effect of target species, height of the central tree and altitude on the number of individuals, species richness and relative abundance of juveniles and adults in the communities. The link function for normal distribution and residual distributions of models was evaluated to select the quasi-Poisson distribution as the most suitable (Crawley, Reference Crawley2013). The number of individuals, species richness and relative abundance was selected as response variables in separate models for juveniles and adults. The predictors were represented by two continuous explanatory variables (altitude as an abiotic factor and height of the central plant as a biotic factor) and one categorical variable factor (i.e. plot). We also obtained a null model with only the intercept, and all variables in a single model formed the global model.
The models were compared using the Akaike information criterion (AIC). Those with the lowest AIC correction value for finite samples (Δ AICc) were considered the most suitable for the data (Burnham and Anderson, Reference Burnham and Anderson2002). Models with Δ AICc<2.0 were considered equally plausible to explain the variation in observed data (Burnham and Anderson, Reference Burnham and Anderson2002, Burnham et al. Reference Burnham, Anderson and Huyvaert2011). We also obtained the corrected Akaike weight (wAICc), indicating the relative suitability of the models (Portet, Reference Portet2020). Analyses were performed in R3.4.4, using the packages vegan, APE and lme4. To estimate predictor coefficients on a comparable scale, we used the package jtools (Long, Reference Long2020).
To assess the similarity in species composition and abundance among plots, we applied the UPMGA (unweighted pair group method with arithmetic mean) based on Jaccard (Jaccard, Reference Jaccard1908) and Bray–Curtis coefficients (Bray and Curtis, Reference Bray and Curtis1957). Cluster confidence was calculated based on bootstrap, using 1000 replications in PAST 3.23 (Hammer et al. Reference Hammer, Harper and Ryan2001). For each plot, we calculated beta diversity and its turnover and nestedness components between juveniles and adults using the package betapart (Baselga, Reference Baselga2010), also implemented in R3.4.4.
Results
We recorded 5,173 individuals belonging to 110 tree species: 76 species (2,264 individuals) as juveniles and 101 (2,909 individuals) as adults. Fifty-seven species (804 individuals) of juveniles and 85 species of adults (598) were recorded in Hancornia plots, 60 (746) of juveniles and 76 (1,077) of adults in Himatanthus plots and 60 (714) of juveniles and 78 (1,232) of adults in Control plots (Figure 2; Supplementary Information S2). Considering juvenile and adult trees in the three types of plots, 34 species (29.35%) were found exclusively as adults and nine (7.33%) exclusively as juveniles; 67 species (63.30%) were recorded in both stages. Hancornia plots had more exclusive species (15) than Himatanthus (5) and Control (3) plots; the three types of plots shared 33 (30.27%) species. Control plots showed more dead individuals (11) than Hancornia (5) and Himatanthus (9) plots (Supplementary Information S2).

Figure 2. Sample design and results. Number of individuals (ind.) and species (spp.) in the woody community and associations of Hancornia, Himatanthus and Control plots, according to juvenile and adult trees.
The three plot types showed similar richness for juveniles when the sampling effort is controlled, tending to stabilisation after a rapid initial accumulation (Figure 3a). If twice the number of plots was collected for each type (extrapolated species richness), we expected an increase of seven species (64 vs 57) for juveniles in Hancornia plots, five (65 vs 60) in Himatanthus plots and 15 (75 vs 60) in Control plots (Figure 3a). The Hancornia plots showed higher richness for adult tree species when sampling effort is controlled; Himatanthus and Control plots showed similar richness, with the three types of plots tending to stabilise after a rapid initial accumulation (Figure 3b). If twice the number of plots was collected for each type (species richness extrapolated), increases of seven (92 vs 85) species were estimated for adults in Hancornia plots, five (81 vs 76) in Himatanthus plots and 15 (93 vs 78) in Control plots (Figure 3b).

Figure 3. Expected number of species obtained from a rarefaction analysis based on the sampling effort (10 × 10 m plots) in Hancornia, Himatanthus and Control plots: (a) juvenile and (b) adult trees.
Compared to Control plots, Hancornia plots showed lower abundance (number of individuals) and Himatanthus plots higher relative abundance in adults. No parameter analysed here showed significant differences between Control and Hancornia or Himatanthus plots for juveniles (Table 1). Relative abundance was higher in adults than in juveniles in all types of plots. However, Control plots showed relatively higher numbers of adult individuals (abundance) and species (richness), a difference not observed in Hancornia and Himatanthus plots. In contrast, Himatanthus plots showed a higher adult relative abundance, a relative difference not shared with Control and Hancornia plots (Table 2).
Table 1. Comparison between plot types (Hancornia, Himatanthus and Control) according to plant stages (juveniles and adults), showing median and interquartile range of number of individuals (abundance), number of species (richness) and relative (species) abundance

Medians and interquartile ranges followed by the same letter did not differ based on Kruskal–Wallis H test (i.e. p > 0.05); ** indicates p < 0.01.
abMedians and interquartile ranges followed by the same letter did not differ based on Kruskal–Wallis H test (p < 0.05).
Table 2. Comparison between life stages (juveniles and adults) according to each type of plot (Hancornia, Himatanthus and Control), showing median and interquartile range of number of individuals (abundance), number of species (richness) and relative (species) abundance

* Indicates p < 0.05, ** p <0.01 and *** p < 0.001, based on Kruskal–Wallis H test.
In juvenile trees, the best-fit models contained only the altitude variable with a significant positive effect for the number of individuals (∼ 85%, Est. 0.008 p < 0.001), number of species (∼ 65%, Est. 0.005 p < 0.001) and relative abundance (∼ 65%, Est. 0.005 p < 0.001) (Fig. 4; Table 3). In adults, the best-fit models for the number of individuals (∼ 50%), number of species (∼ 27%) and relative abundance (∼ 53%) contained the variable plot (target species), in combination with altitude and height of the central plant, only altitude (though not significantly better than a null hypothesis) or alone, respectively (Figure 4; Table 3). For adults, the significant effects observed in the number of individuals were produced by Hancornia (Est. −0,682 p < 0.001) and the height of central plant (Est. −0,150 p < 0.01), in the number of species by Hancornia (Est. −0.306 p < 0.05) and altitude (Est. 0.004 p < 0.05) and in relative abundance by Himatanthus (Est. 0.378 p < 0.01; Table 3).

Figure 4. Influence of abiotic (altitude) and biotic (plots and height of the central plant) factors in explanatory models for the number of individuals (abundance), number of species (richness) and relative (species) abundance of (a) juvenile and (b) adult trees in Hancornia, Himatanthus and Control plots indicated by the corrected Akaike weight (wAICc); * indicates the most suitable models (Table 4 and Supplementary Information S3).
Table 3. Parameters and p-values estimated in the generalised linear model explaining the number of individuals, number of species and relative abundance of the influence of abiotic (altitude) and biotic (plots and height of central plant) factors in juvenile and adult trees

1Difference between Control plots and Hancornia (Han) and Himatanthus (Him) plots.
* Indicates p-values < 0.05, ** p-values < 0.01 and *** p-values < 0.001.
Similarity coefficients, both Jaccard and Bray–Curtis, highly supported clusters of juvenile and adult trees (Figure 5). Within these groups, Himatanthus plots were more dissimilar for juveniles and Hancornia plots more dissimilar for adults when compared to Control plots (Figure 5a); the higher dissimilarity of Hancornia plots for juveniles with Bray–Curtis was not supported (i.e. <50% bootstrap; Figure 5b)

Figure 5. Dendrogram of similarity between woody species for juvenile and adult trees: (a) Jaccard coefficient, based on species presence and absence matrix and (b) Bray–Curtis coefficient, based on species abundance matrix.
Beta diversity between juveniles and adults was similar in the three types of plots, ranging from 0.281 in Hancornia plots to 0.294 in Himatanthus plots. However, the dissimilarity between juveniles and adults in Hancornia plots was mainly due to nestedness than turnover, whereas turnover in Himatanthus and Control plots was higher than nestedness (Table 4).
Table 4. Beta diversity (total dissimilarity) and their components (turnover and nestedness) between juvenile and adult trees in Hancornia, Himatanthus and Control plots

Discussion
Our results support the hypothesis that Hancornia speciosa and Himatanthus obovatus act as biotic filters, influencing tree recruitment in woody communities within savannas. Therefore, they confirm that plant–plant interactions, extending beyond the extensively discussed complex tree–grass coexistence (e.g. Archibald et al. Reference Archibald, Lehmann, Gómez-Dans and Bradstock2013, Chagas and Pelicice, Reference Chagas and Pelicice2018, Dantas et al. Reference Dantas, Hirota, Oliveira and Pausas2016, Flake et al. Reference Flake, Honda, Pilon, Hoffmann and Durigan2022, Holdo and Nippert, Reference Holdo and Nippert2023, Lehmann et al. Reference Lehmann, Archibald, Hoffmann and Bond2011, Levick and Rogers, Reference Levick and Rogers2011, Midgley et al. Reference Midgley, Lawes and Chamaillé-Jammes2010, Sankaran, Reference Sankaran2019, Sankaran et al. Reference Sankaran, Ratnam and Hana2004, Scholes and Archer, Reference Scholes and Archer1997, Sharpe, Reference Sharpe1992), also play a role in shaping savanna dynamics within life forms.
The distinct species composition patterns observed in juveniles and adults, along with lower tree abundance and a higher number of rare tree species during the adult stage, resulting in higher evenness (see Chagas et al. Reference Chagas, Rapini and Collevatti2020), provide further evidence for the selective impact of Hancornia speciosa on the woody community. The beta diversity between juveniles and adults in H. speciosa-associated communities, primarily driven by nestedness, strengthens this hypothesis. Hence, Hancornia speciosa seems to restrict the coexistence of tree species and limit their abundance, as traditionally expected in communities primarily driven by negative interactions (Hutchinson, Reference Hutchinson1967, Tilman, Reference Tilman2004, Whittaker, Reference Whittaker1967, Reference Whittaker1969). Consequently, H. speciosa appears to function as a biotic filter during the development of woody plants.
The negative interactions of H. speciosa with neighbouring communities during the recruitment result in the exclusion of less-tolerant species, reducing tree richness and constraining populations of tolerant species. Consequently, the abundance of adults in the vicinity is kept lower, reducing the direct competition with other trees (see references in Schöb et al. Reference Schöb, Michalet, Cavieres, Pugnaire, Brooker, Butterfield, Cook, Kikvidze, Lortie, Xiao, Al Hayek, Anthelme, Cranston, García, Le Bagousse-Pinguet, Reid, le Roux, Lingua, Nyakatya, Touzard, Zhao and Callaway2014, Verdú et al. Reference Verdú, Jordano and Valiente-Banuet2010). The lower abundance associated with H. speciosa possibly explains why fewer dead trees are found closer to this species. The vacant space and increased light resulting from plant exclusion in its vicinity are expected to facilitate new colonisations and the dominance of tolerant species, leading to higher abundances of juvenile trees and herbs (Grime, Reference Grime1998, Lima and Gandolfi, Reference Lima and Gandolfi2009). The reduced tree cover favours the grass layer and consequently the likelihood of fires occurring closer to H. speciosa. However, no significant differences were found in the richness and abundance of herbs (Chagas et al. Reference Chagas, Rapini and Collevatti2020) and juvenile trees associated with this species. Since germination is generally less affected by the negative influence of allelochemicals compared to plant growth (Gui Ferreira and Aquila, Reference Gui Ferreira and Aquila2000, and references therein), selection pressure is probably more intense during development. This could explain the observed differences in species composition and abundance between juvenile and adult trees (Barnes and Archer, Reference Barnes and Archer1999, Salles and Schiavini, Reference Salles and Schiavini2007).
The higher dissimilarity of juvenile composition in the community surrounding Himatanthus obovatus suggests that this species has a greater influence on initial recruitment compared to Hancornia speciosa (Barnes and Archer, Reference Barnes and Archer1999, Peláez et al. Reference Peláez, Dirzo, Fernandes and Perea2019). It likely modifies the environment by providing shading, which in turn reduces surrounding temperature and water loss, thereby facilitating initial recruitment (Bruno et al. Reference Bruno, Stachowicz and Bertness2003, Stachowicz, Reference Stachowicz2001). Positive plant–plant interactions typically involve individuals from the upper strata (shrubs and trees) influencing the components of the lower stratum (herbs and juvenile trees; Barnes and Archer, Reference Barnes and Archer1999, Peláez et al. Reference Peláez, Dirzo, Fernandes and Perea2019, Vega-Álvarez et al. Reference Vega-Álvarez, García-Rodríguez and Cayuela2019). However, altitude was found to have a greater influence than target species in explaining the variation in communities of juvenile trees.
These positive or negative effects of biotic interactions observed in this study may have implications beyond the taxonomic attributes of plant communities (i.e. species richness, species composition, dissimilarity pattern) under different target species and control treatment. For example, it has been shown that density-dependent biotic factors can affect the phylogenetic structure of plant communities (Campos et al. Reference Campos, Schaefer, Pontara, Senra, Viana, Oliveira, Candido and Villa2021, Carrión et al. Reference Carrión, Gastauer, Mota and Meira-Neto2017, Galván-Cineros et al. Reference Galván-Cisneros, Gastauer, Massante, Villa and Meira-Neto2023). Specifically, in a scenario of high floristic similarity (i.e. phylogenetically related species are ecologically more similar), their ecological traits can be conserved within evolutionary niches, and then facilitation can be the primary process determining phylogenetic overdispersion (Cavender-Bares et al. Reference Cavender-Bares, Kozak, Fine and Kembel2009, Webb et al. Reference Webb, Ackerly, McPeek and Donoghue2002). However, facilitation also can promote phylogenetic evenness if ecological traits are predominantly convergent (Carrión et al. Reference Carrión, Gastauer, Mota and Meira-Neto2017, Cavender-Bares et al. Reference Cavender-Bares, Kozak, Fine and Kembel2009). Furthermore, we presume that the higher dissimilarity of juveniles is shaped by colonisations and stochastic recruitment processes (i.e. under neutrality-based stochastic hypotheses; Cavender-Bares et al., Reference Cavender-Bares, Kozak, Fine and Kembel2009; Webb et al., Reference Webb, Ackerly, McPeek and Donoghue2002), which can determine a random phylogenetic structure simultaneously with environmental factors in the Cerrado.
Implications for conservation
The MATOPIBA region intersects the Amazon agricultural frontier within the eastern and southern Amazonia, commonly called the ‘arc of deforestation’. This vast area is experiencing intense degradation in the Cerrado–Amazonia transition (Marques et al. Reference Marques, Marimon Junior, Marimon, Matricardi, Mews and Colli2020). The deforestation in this region can have implications for the climate, potentially leading to prolonged and warmer dry seasons in the region (Costa and Pires, Reference Costa and Pires2010, Marengo et al. Reference Marengo, Jimenez, Espinoza, Cunha and Aragão2022). The savannas of Tocantins studied here are located in the centre of an extensive area characterised by bi-stable tree cover conditions (Staver et al. Reference Staver, Archibald and Levin2011), at the interface between two phytogeographic domains, one currently covered mainly by savannas and the other dominated by forests; they are under significant threats from land use (Marques et al. Reference Marques, Marimon Junior, Marimon, Matricardi, Mews and Colli2020, Pivello et al. Reference Pivello, Vieira, Christianini, Ribeiro, Menezese, Berlinck, Melog, Marengo, Tornquist, Tomas and Overbeck2021, Sano et al. Reference Sano, Rosa, Scaramuzza, Adami, Bolfe, Coutinho, Esquerdo, Maurano, Narvaes, Oliveira Filho, Silva, Victoria, Ferreira, Brito, Bayma, Oliveira and Bayma-Silva2019) and local climate change (Costa and Pires, Reference Costa and Pires2010).
Fire is a recurrent disturbance in this region, impacting areas of varying sizes and resulting in landscapes with patches at different stages of regeneration. Consequently, the savannas consist of mosaics of associations at different successional stages, with distinct species composition and abundance (Chagas and Pelicice, Reference Chagas and Pelicice2018, Silva et al. Reference Silva, Loiola, Rosatti, Silva, Cianciaruso and Batalha2011). Effective fire management is essential for maintaining the diversity of vegetation types and plant diversity within this mosaic (Abreu et al. Reference Abreu, Hoffmann, Vasconcelos, Pilon, Rossatto and Durigan2017, Arnan et al. Reference Arnan, Cerdá and Rodrigo2020, Buisson et al. Reference Buisson, Le Stradic, Silveira, Durigan, Overbeck, Fidelis, Fernandes, Bond, Hermann, Mahy, Alvarado, Zaloumis and Veldman2019, Durigan et al. Reference Durigan, Pilon, Abreu, Hoffmann, Martins, Fiorillo, Antunes, Carmignotto, Maravalhas, Vieira and Vasconcelos2020, Pivello et al. Reference Pivello, Vieira, Christianini, Ribeiro, Menezese, Berlinck, Melog, Marengo, Tornquist, Tomas and Overbeck2021). However, the composition and structure of plant communities can also influence soil nutrients as well as nutrient turnover and availability (Pellegrini, Reference Pellegrini2016). Consequently, they play a role in shaping the heterogeneity of tree cover and the distribution of fire regimes in savanna landscapes.
Differences observed between juvenile and adult trees in our study support the negative influence of H. speciosa on the neighbouring plant community and suggest the possibility of a positive interaction between H. obovatus and the surrounding plant community (Barnes and Archer, Reference Barnes and Archer1999, Peláez et al. Reference Peláez, Dirzo, Fernandes and Perea2019). In regions where H. speciosa is more prevalent, there is a tendency for trees to be less abundant compared to the average savanna, which potentially increases the risk of fires and reduces the likelihood of forest encroachment. Both species act as structuring agents in regenerating communities and contribute to the formation of successional mosaics of associations, resulting in higher levels of beta diversities in fire-prone savannas.
Tree recruitment in savannas can be directly influenced by the composition and abundance of adult trees, but it is also shaped by environmental filters and limiting biotic interactions (Cadotte and Tucker, Reference Cadotte and Tucker2017, Lortie et al. Reference Lortie, Brooker, Choler, Kikvidze, Michalet, Pugnaire and Callaway2004). In the absence of disturbance, tree-on-tree competition is a potential limiting factor for tree cover, thereby impacting tree–grass ratios (Sankaran et al. Reference Sankaran, Ratnam and Hana2004). This study demonstrated that local tree-on-tree interactions can also drive the composition and structure of woody communities in savannas with intense fire regimes. These interactions affect tree cover and contribute to landscape heterogeneity at a regional scale. Considering the spatial distribution of tree species and their associated communities is crucial for understanding savanna dynamics and should be noticed in management plans and restoration projects. Therefore, tree-on-tree interactions should be taken into account when implementing burning patch mosaics in savannas to ensure the persistence of ecological processes essential for biodiversity maintenance.
Supplementary material
The supplementary material for this article can be found at https://doi.org/10.1017/S0266467423000196
Acknowledgements
We thank Cássia Beatriz Munhoz and Rafael Costa for their suggestions to improve the initial version of the manuscript.
Financial support
This study is part of the first author’s PhD thesis, developed at PPGBot-UEFS, with a fellowship from the Conselho Nacional de Desenvolvimento Científico e Tecnológico – Brazil (CNPq) and support from the Aperfeiçoamento de Pessoal de Nível Superior – Brazil (CAPES) – Finance Code 001. CNPq productivity grants have continuously supported AR (Fellowship nº. 307396/2019-3) and RGC. RGC was supported by a Visiting Research Fellowship from São Paulo Research Foundation (FAPESP, process nº 2022/10760-1).
Competing interests
None.
Ethical statement
None.