Introduction
Bavenite is a common secondary mineral formed typically after altered beryl in granitic pegmatites, though it is also commonly found as tabular crystals or coatings on crystals of feldspar and quartz in miarolitic pockets with no apparent relation to an altered primary Be mineral (Černý, Reference Černý and Grew2002). An exceptional occurrence has been described from a Ca-skarn (Manning et al., Reference Manning, Putthapiban and Suensilpong1983). The composition and crystal structure of bavenite has been discussed in several papers (Hawthorne and Huminicki, Reference Hawthorne, Huminicki and Grew2002; Černý, Reference Černý and Grew2002; Lussier and Hawthorne, Reference Lussier and Hawthorne2011 and references therein) demonstrating variable concentrations of Be and Al via the substitution T(3)Be T(4)Si O(2)OH = T(3)Si T(4)Al O(2)O (Černý, Reference Černý and Grew2002). Friis et al. (Reference Friis, Makovicky, Weller and Lemée-Cailleau2010) originally formulated the bohseite end-member as Ca4Be3AlSi9O25(OH)3 on the basis of a sample from the Ilímaussaq complex, Greenland. However, subsequent analyses revealed that this formula did not represent the maximum Be content possible, and consequently end-member bohseite was redefined as Ca4Be4Si9O24(OH)4 (Hålenius et al.; Reference Hålenius, Hatert, Pasero and Mills2015; Szełęg et al., Reference Szełęg, Zuzens, Hawthorne, Pieczka, Szuszkiewicz, Turniak, Nejbert, Ilnicki, Friis, Makovicky, Weller and Lemée-Cailleau2017).
A very high incompatibility with major and most minor and accessory minerals, with the notable exception of cordierite, sapphirine, staurolite and margarite (Grew et al., Reference Grew, Yates, Shearer, Hagerty, Sheraton and Sandiford2006) is caused by the relatively small ionic size and low charge of Be2+ cations. In addition, the compositional variability of fluids, particularly those associated with granitic pegmatites, results in a diverse suite of Be minerals (Hawthorne and Huminicki, Reference Hawthorne, Huminicki and Grew2002; Grew, Reference Grew and Grew2002; Grew and Hazen, Reference Grew and Hazen2014). Because externally imposed chemical potentials govern the stability of Be minerals together with P–T-conditions (e.g. Barton, Reference Barton1986; Grew, Reference Grew and Grew2002; Wang and Li, Reference Wang and Li2020), the individual Be minerals and their mineral assemblages can serve as useful geochemical mineral indicators (Burt, Reference Burt1978; Wood, Reference Wood1992; Markl and Schumacher, Reference Markl and Schumacher1997; Markl, Reference Markl2001; Černý, Reference Černý and Grew2002; Barton and Young, Reference Barton, Young and Grew2002; Franz and Morteani, Reference Franz, Morteani and Grew2002; Rao et al., Reference Rao, Wang and Hu2011; Palinkaš et al., Reference Palinkaš, Wegner, Čobić, Palinkaš, Barreto, Váczi and Bermanec2014; Uher et al., Reference Uher, Ozdín, Bačík, Števko, Ondrejka, Rybnikova, Chládek, Fridrichová, Pršek and Puškelová2022). Bavenite–bohseite has not been studied experimentally or treated in thermodynamic studies which have been performed solely in the BeO–Al2O3–SiO2–H2O (BASH) system (e.g. Hsu, Reference Hsu1983; Franz and Morteani, Reference Franz and Morteani1984; Barton, Reference Barton1986) and in the Na–BASH system (Markl, Reference Markl2001). Černý (Reference Černý and Grew2002) assumed an alkaline rather than an acidic environment for the origin of bavenite.
Beryllium minerals are typical accessory phases in the granitic pegmatites classified by Černý and Ercit (Reference Černý and Ercit2005). Beryl is by far the most abundant primary Be mineral (Černý, Reference Černý and Grew2002; London, Reference London2008; Grew and Hazen, Reference Grew and Hazen2014) and only sporadically minor to very rare primary phenakite, chrysoberyl, gadolinite- and helvine-group minerals, rhodizite, londonite, hurlbutite, beryllonite, hambergite (Černý, Reference Černý and Grew2002) and milarite-group minerals (Novák et al., Reference Novák, Cícha, Čopjaková, Škoda and Galiová2017a) also occur in granitic pegmatites. Bavenite–bohseite is one of the most abundant secondary Be minerals in LCT (Li–Cs–Ta) and NYF (Nb–Y–F) pegmatites together with bertrandite and less common milarite-group minerals, bityite, phenakite and several rare species (Černý, Reference Černý and Grew2002; Grew and Hazen, Reference Grew and Hazen2014). Bavenite–bohseite also occurs in alkaline pegmatites (Grice et al., Reference Grice, Rowe, Poirier, Pratt and Francis2009; Friis et al., Reference Friis, Makovicky, Weller and Lemée-Cailleau2010).
We examined textural and paragenetic position, mineral assemblages, composition, and Raman spectra of bavenite–bohseite from a variety of granitic pegmatites in the Bohemian Massif, Czech Republic to determine paragenetic and morphological types, and compositional variability of the bavenite–bohseite series. These were utilised as indicators of fluid composition and mobility of elements (Ca, Be and Al) in hydrothermal conditions, as well as potential sources of fluids. In addition, the mineral assemblages of bavenite–bohseite samples were studied in detail and used below to discuss the stability field of bavenite–bohseite compared to Be minerals in the BASH system.
Brief description of the examined granitic pegmatites
Internal structure
Bavenite–bohseite was examined from granitic pegmatites in various geological regions of the Bohemian Massif, Czech Republic: Moldanubian Zone (11 sites); Silesicum (2 sites); and Lugicum (2 sites) (Fig. 1; Table 1). Intragranitic NYF pegmatite dykes from Vepice IV and several dykes from the Třebíč durbachite pluton (Table 1) evolved from parental melagranite via transitional contacts inwards: a thin granitic unit; a graphic unit; a blocky K-feldspar; and a central miarolitic pocket (Qz+Ab+Kfs) developed at the Vepice IV pegmatite. Nests of albite, typically adjacent to quartz core, occur locally in the Třebíč pegmatites. The pegmatites Kracovice, Vlastějovice, Dražice, Drahonín IV and Věžná I (Table 1) exhibit, from the contact inwards, an outer medium-grained granitic unit, a coarse-grained inner unit with abundant graphic intergrowths (Qz+Kfs, Qz+Ab, rarely Qz+Tur, Qz+Crd), and blocky K-feldspar. Irregular aggregates of albite as well as a well-defined albite unit commonly replace blocky K-feldspar. Miarolitic pockets (Qz+Ab+Kfs) occur in Vlastějovice (rare) and Drahonín IV (common) pegmatites. At the Kracovice, Drahonín IV and Věžná I pegmatites, a quartz core is also developed (Table 1). The Ruprechtice pegmatite is known from only a single large fragment of massive quartz from the quartz core and adjacent coarse-grained unit (Kfs+Qz+Ab+Ms). The pegmatite dyke at Nýznerov is composed of a fine-grained border unit, a dominant coarse-grained unit with biotite in the outer portion and muscovite + beryl in the inner portions, and a small quartz core with rare primary phenakite. Two pegmatites from Maršíkov near Šumperk differ in size and degree of tectonic/metamorphic overprint (Table 1). The Schinderhübel I pegmatite forms a thin zoned dyke evolving from a strongly deformed medium-grained wall unit into a coarse-grained intermediate unit locally with saccharoidal albite and ultimately into a small quartz core (Černý et al., Reference Černý, Novák and Chapman1992; Chládek et al., Reference Chládek, Uher and Novák2020, Reference Chládek, Uher, Novák, Bačík and Opletal2021). In the Scheibengraben pegmatite dyke, up to ~10 m thick, individual pegmatite units (coarse-grained, graphic, blocky K-feldspar) are rather irregularly distributed; late saccharoidal albite extensively replaced the other pegmatite units (Novák et al., Reference Novák, Černý and Uher2003) and the tectonic overprint is weak to moderate.
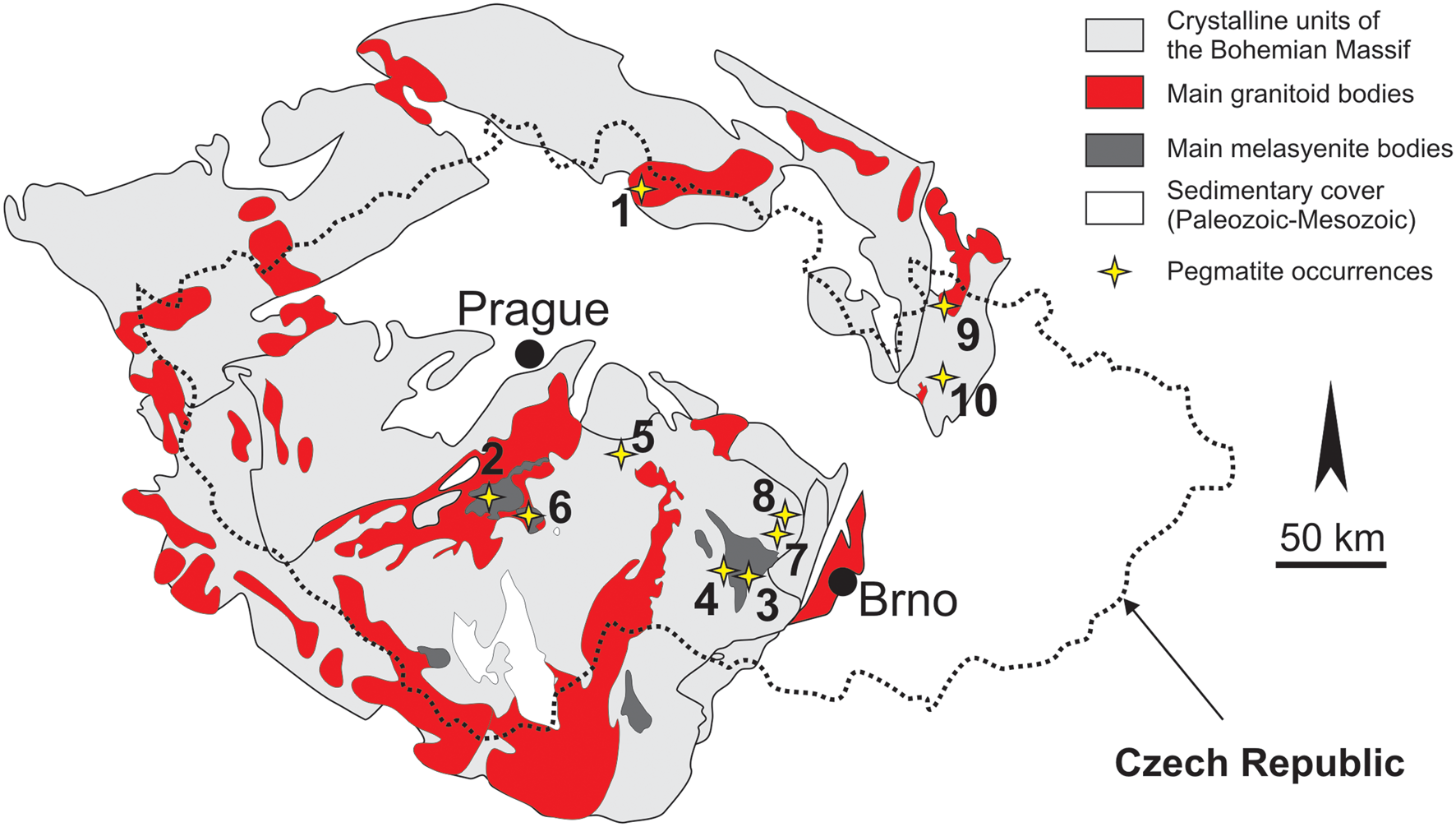
Figure 1. Schematic geological map of the Bohemian Massif with the locations of the investigated pegmatites: 1 – Ruprechtice; 2 – Vepice IV; 3 – Číměř I and III, Klučov III, Kožichovice I and II; 4 – Kracovice; 5 – Vlastějovice; 6 – Dražice; 7 – Drahonín IV; 8 – Věžná I; 9 – Maršíkov Schinderhübel I, Scheibengraben; and 10 – Nýznerov.
Table 1. Characteristics of the pegmatites investigated.
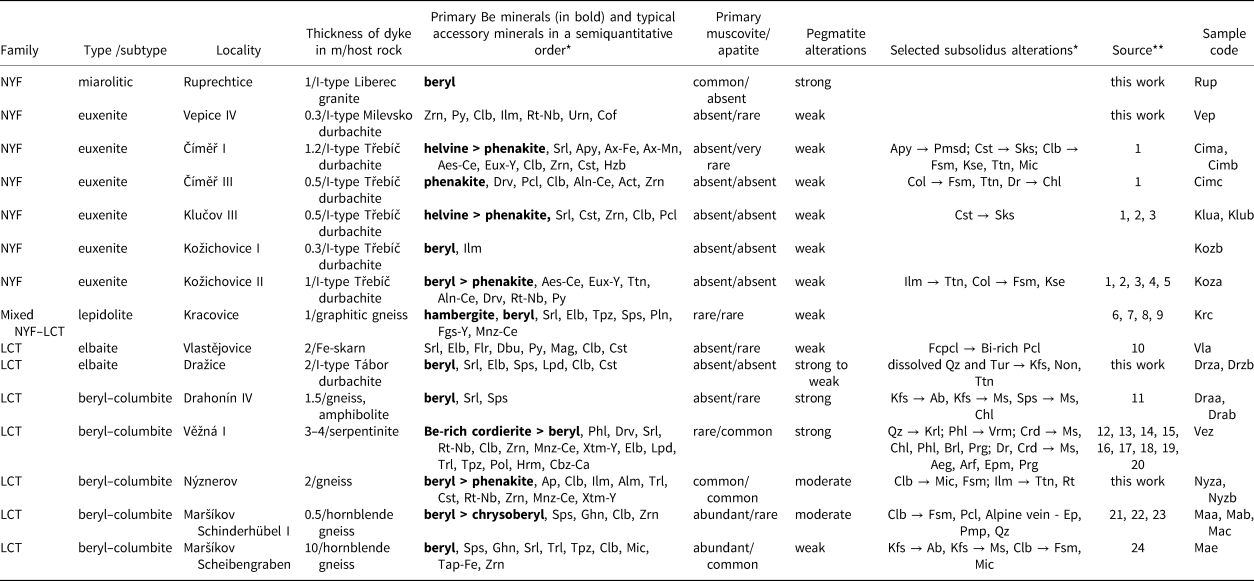
* Symbols according to Warr (Reference Warr2021).
** Sources: 1 – Zachař et al. (Reference Zachař, Novák and Škoda2020); 2 – Škoda et al. (Reference Škoda, Novák and Houzar2006); 3 – Novák et al. (Reference Novák, Škoda, Filip, Macek and Vaculovič2011); 4 – Novák and Filip (Reference Novák and Filip2010); 5 – Výravský et al. (Reference Výravský, Škoda and Novák2019); 6 – Němec (Reference Němec1990); 7 – Čopjaková et al. (Reference Čopjaková, Škoda, Vašinová-Galiová, Novák and Cempírek2015); 8 – Novák et al. (Reference Novák, Škoda, Gadas, Krmíček and Černý2012); 9 – Novák et al. (Reference Novák, Burns and Morgan1998); 10 – Novák et al. (Reference Novák, Kadlec and Gadas2013); 11 – Sojka (Reference Sojka1969); 12 – Černý (Reference Černý1960); 13 – Černý Reference Černý1963); 14 – Černý (Reference Černý1965); 15 – Novák et al. (Reference Novák, Gadas, Cempírek, Škoda, Breiter, Kadlec, Loun and Toman2015b); 16 – Dosbaba and Novák (Reference Dosbaba and Novák2012); 17 – Toman and Novák (Reference Toman and Novák2018); 18 – Toman and Novák (Reference Toman and Novák2020); 19 – Gadas et al. (Reference Gadas, Novák, Galiová, Szuszkiewicz, Pieczka, Haifler and Cempírek2020); 20 – Čopjaková et al. (Reference Čopjaková, Prokop, Novák, Losos and Gadas2021); 21 – Černý et al. (Reference Černý, Novák and Chapman1992); 22 – Chládek et al. (Reference Chládek, Uher, Novák, Bačík and Opletal2021); 23 – Dolníček et al. (Reference Dolníček, Nepejchal and Novák2020); and 24 – Novák et al. (Reference Novák, Černý and Uher2003).
Primary Be minerals, their mineral assemblages and compositions
Primary Be minerals as precursors of secondary bavenite–bohseite have been described from numerous localities (e.g. Třebíč Pluton – Zachař, Reference Zachař2021). At the Třebíč euxenite-type pegmatites, two distinct assemblages of primary Be minerals were recognised by Zachař et al. (Reference Zachař, Novák and Škoda2020); the assemblage B (beryl ± phenakite) and the assemblage HD (helvine–danalite ± phenakite). Beryl and helvine–danalite typically do not occur within the same pegmatite body (Table 1). Beryl forms prismatic greenish crystals, up to 5 cm in length, in albite and quartz (Novák and Filip, Reference Novák and Filip2010; Zachař et al., Reference Zachař, Novák and Škoda2020). Strongly-altered equidimensional grains to subhedral crystals of reddish brown (fresh) to dark brown (altered) helvine–danalite, up to 5 cm in size, occur in tourmaline-bearing pegmatites from a graphic unit, a blocky unit, and albite-rich portions. Rare prismatic colourless crystals of phenakite, up to 3 cm in length, typically from massive quartz, are usually fresh (Zachař et al., Reference Zachař, Novák and Škoda2020). No primary Be mineral has been identified at the Vlastějovice (Novák et al., Reference Novák, Kadlec and Gadas2013) and Vepice IV pegmatites. Beryl is the sole primary Be mineral at the Dražice, Drahonín IV, Ruprechtice and Scheibengraben pegmatites (Table 1). Rare hambergite (Němec, Reference Němec1990; Novák et al., Reference Novák, Burns and Morgan1998), chrysoberyl (Černý et al., Reference Černý, Novák and Chapman1992) and phenakite were identified together with dominant beryl in the Kracovice, Schinderhübel I and Nýznerov pegmatites, respectively; however, typically these minerals were not altered (Table 1). Phenakite and hambergite are evidently primary minerals, whereas chrysoberyl is a product of tectono-metamorphic overprinting (Černý et al., Reference Černý, Novák and Chapman1992). Beryl forms short to long prismatic subhedral to euhedral crystals, 1–10 cm in diameter. Secondary pockets formed from dissolved prismatic crystals of beryl at the Drahonín IV pegmatite were up to ~40 cm in length (Sojka, Reference Sojka1969). A radial aggregate of strongly altered beryl from Ruprechtice, up to ~10 cm in diameter, is located at the contact of massive quartz and a coarse-grained unit. Beryl- and Be-enriched cordierite occur in the Věžná I pegmatite (Table 1). Less commonly beryl forms greyish prismatic crystals, up to 20 cm in length, typically strongly altered. Primary Be minerals at all examined localities are enclosed mostly in albite, in albitised K-feldspar, or in massive quartz of the quartz core typically adjacent to an albite-rich unit.
Representative compositions of primary beryl and helvine–danalite from some localities are given in Tables 2 and 3. Contents of Na, Mg and Fe are relatively elevated in beryl from pegmatites of the Třebíč Pluton and in Nýznerov, whereas beryl from Kracovice and Dražice pegmatites is close to the ideal composition, or is only slightly enriched in Na. Compositons of of helvine–danalite are close to the midpoint between both end-members with negligible Zn contents. Phenakite compostions are very close to the ideal formula (Zachař et al., Reference Zachař, Novák and Škoda2020).
Table 2. Representative compositions of primary beryl (see Table 1 for sample codes).
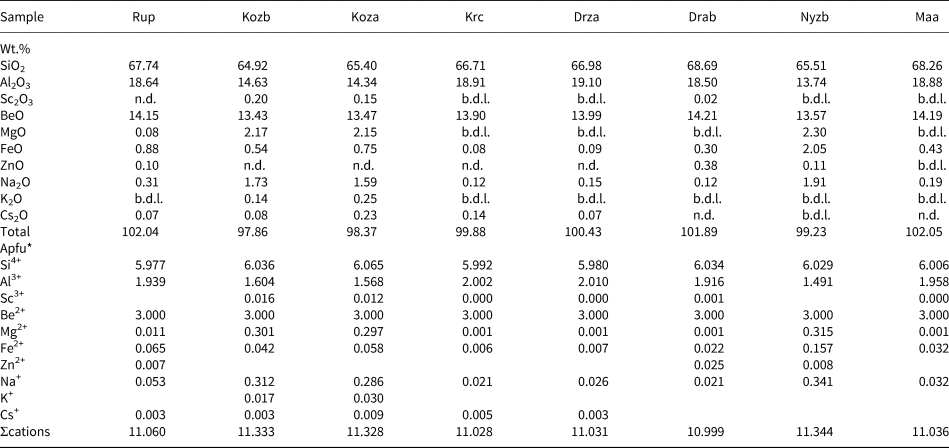
*The formulae were calculated on the basis of T + M = 8 apfu and stoichiometric Be = 3 apfu.
b.d.l. – below detection limit; n.d. – not detected
Table 3. Representative compositions of primary helvine–danalite (see Table 1 for sample codes).
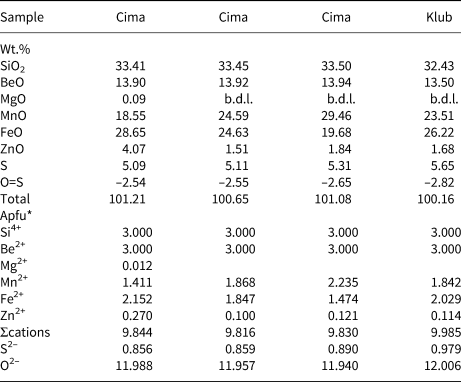
*The formulae were calculated on the basis of Si = 3 apfu and stoichiometric Be = 3 apfu.
b.d.l. – below detection limit.
Samples and analytical methods
The samples of bavenite–bohseite were obtained from several sources including the mineralogical collections of the Moravian Museum, Brno (8) and the National Museum, Prague (4), and the research collections of A. Zachař (5), M. Nepejchal (2) and M. Novák (2). The abbreviations for the minerals used in the text and figures are after Warr (Reference Warr2021).
Analyses of the samples were performed using Cameca SX-100 electron microprobes operating in the wavelength-dispersive (WDS) mode at the National Museum, Prague (1), and the Department of Geological Sciences, Masaryk University Brno, Czech Republic (2). Bavenite–bohseite was analysed using an acceleration voltage of 15 kV, beam current of 10 nA (1) or 15 nA (2), and 2–5 μm beam diameter (1, 2). The following analytical lines and standards were used: apatite (PKα); wollastonite (SiKα, CaKα); sanidine (AlKα); (1) hematite or (2) andradite (FeKα); (1) diopside or (2) Mg2SiO4 (MgKα); (1) rhodonite or (2) spessartine (MnKα); albite (NaKα); (1) LiF or (2) topaz (FKα); and halite (ClKα). The peak counting times were usually between 20 and 30 s, those of both backgrounds were between 10 and 15 s. Raw intensities were converted to the concentrations of oxides using the automatic ‘PAP’ matrix-correction procedure (Pouchou and Pichoir, Reference Pouchou, Pichoir and Armstrong1985), including model calculation of BeO to the analytical sum of 100 wt.% and correction of overlaps for P/Ca and Rb/Si. Contents of other elements measured, which are not included in the tables (Ti, Sb, V, Cr, Pb, Zn, Ba, Sr, Cu, Co, Ni, Cs, Rb, K and N), were analysed quantitatively, however the values were below the detection limit (0.04–0.08 wt.% for most elements). Calculation of empirical formula in atoms per formula unit (apfu) was modified according to Lussier and Hawthorne (Reference Lussier and Hawthorne2011) and Szełęg et al. (Reference Szełęg, Zuzens, Hawthorne, Pieczka, Szuszkiewicz, Turniak, Nejbert, Ilnicki, Friis, Makovicky, Weller and Lemée-Cailleau2017) due to the presence of additional minor cations. The calculation was normalised to a sum of 13 cations except for Na and Ca. The Be content was then iterated until Na+Ca = Al+Be+Mg+Fe+Mn. The water content is based on charge balance, assuming 28 anions. The mol.% of the bavenite component was calculated from the ivbAl value, which is total Al (apfu) minus ivaAl, the latter substituting for Si (assuming Si+ivaAl = 9 apfu).
Raman spectra of all the samples were obtained with a LabRam HR Evolution Raman spectrometer system by HORIBA (Horiba Jobin Yvon) with a Peltier-cooled CCD detector and Olympus BX–41 microscope at room temperature, at the Department of Geological Sciences, Masaryk University, Brno. Spectra were calibrated using the Rayleigh line with the 532 nm emission of a Nd:YAG laser. Excitations at 473 nm and 633 nm were chosen to verify the measurements and to eliminate possible analytical artefacts caused by laser-induced photoluminescence. We have recorded the full Raman spectra by scanning the entire range of interest 100–4000 cm−1 using a diffraction grating with 600 grooves per mm, an entrance slit of 100 μm, a confocal hole at 200 μm or 100 μm and a 50 × objective lens. The diameter of the spot for the measurement is ~1 μm. Acquisition time was set to 20 or 40 s and four accumulations. Data were processed with Seasolve PeakFit 4.1.12 and LabSpec 6 software. Band fitting was done after appropriate background correction, assuming Lorentzian–Gaussian band shapes.
Results
Textures and mineral assemblages
We recognised several types of bavenite–bohseite distinct in their relation to an obvious or potential primary Be precursors, overall mineral assemblage and morphology (Table 4): type 1 primary hydrothermal bavenite–bohseite from miarolitic pockets; and two types of secondary bavenite–bohseite i.e. – type 2 proximal in pseudomorphs after a primary Be mineral and type 3 distal on brittle tectonic fractures and fissures of a host pegmatite generally close to altered beryl (Figs 2, 3; see also Novák et al., Reference Novák, Čopjaková, Dosbaba, Galiová, Všianský and Staněk2015a).

Figure 2. BSE images of primary and secondary Be minerals from pegmatites of the Třebíč Pluton. (a) Helvine enclosed in albite replaced by a fine-grained aggregate of proximal bohseite from Číměř I. (b) Phenakite replaced by proximal bohseite, note inclusions of pyrochlore, Číměř III. (c) and (d) Proximal and ‘near-distal’ bavenite–bohseite overgrowing and replacing zoned beryl, Kožichovice II. (e) Secondary proximal milarite and bohseite after helvine, Číměř I. (f) Complex proximal secondary assemblage after beryl with common bavenite–bohseite, bertrandite, K-feldspar and rare gismondine, Kracovice.
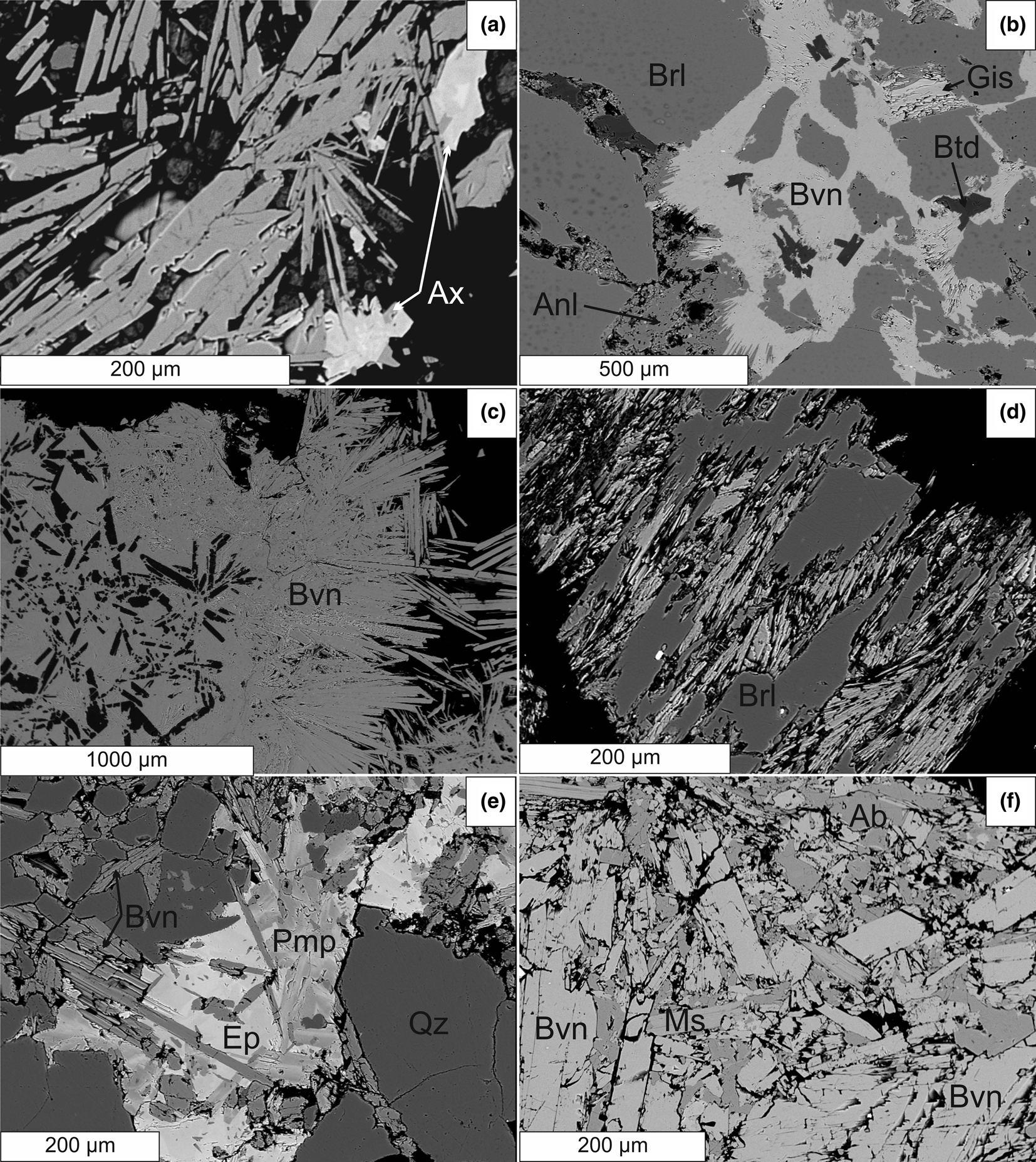
Figure 3. BSE images of primary and secondary Be minerals from the pegmatites Vlastějovice, Drahonín and Maršíkov Schinderhübel I. (a) Hydrothermal bavenite from a miarolitic pocket associated with heterogeneous grains of axinite-Mn from Vlastějovice. (b) Beryl replaced by proximal bavenite–bohseite with small euhedral grains of bertrandite, gismondine and analcime, Drahonín IV. (c) Aggregate of proximal bavenite–bohseite with euhedral crystals of bertrandite, Drahonín IV. (d) Proximal bavenite–bohseite after beryl, Maršíkov Schinderhübel I. (e) Distal bavenite–bohseite in fracture from massive quartz, note associated epidote and pumpellyite and euhedral quartz, Maršíkov Schinderhübel I. (f) Distal bavenite–bohseite associated with muscovite and albite, Maršíkov Schinderhübel I.
Table 4. Mineral assemblages of bavenite–bohseite.
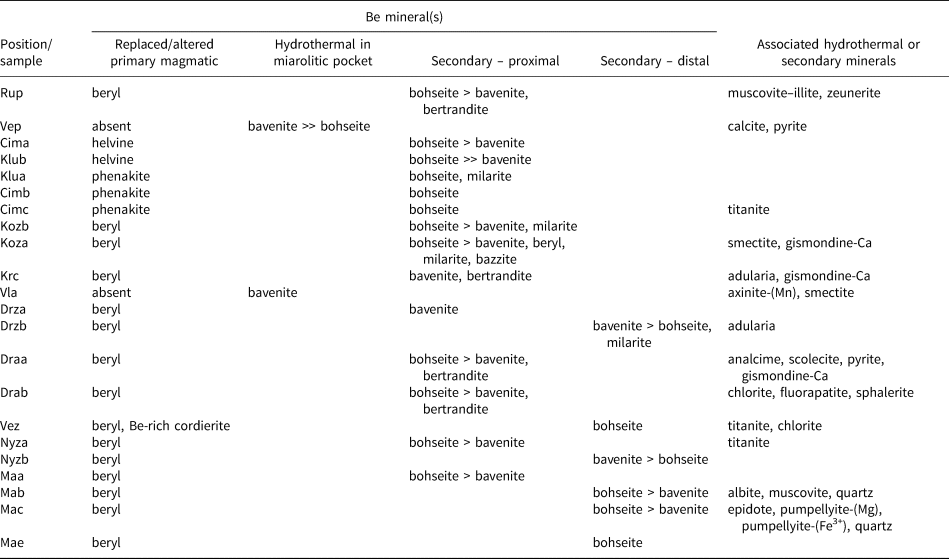
Radial aggregates of tabular or fibrous crystals of bavenite, up to 1.5 cm in size, overgrowing crystals of primary K-feldspar, albite, or quartz in miarolitic pockets, up to 5 cm in size, from the Vlastějovice and Vepice IV pegmatites represent the hydrothermal miarolitic type. The hydrothermal minerals associated with bavenite–bohseite in miarolitic pockets include, at Vlastějovice, zoned, Sn-enriched (≤4.67 wt.% SnO2) axinite-(Mn) + late smectite (Fig. 3a), and at Vepice IV, abundant calcite + pyrite (Table 4).
Proximal secondary Be minerals directly replacing a primary Be mineral in pseudomorphs are typical in pegmatites of the Třebíč Pluton (Table 4; Fig. 2a–e). Bavenite–bohseite in thin veinlets cutting primary Be minerals and as rare masses in helvine–danalite, is the most widespread mineral and predominates over minor to rare milarite, agakhanovite, bazzite, secondary beryl, secondary phenakite, secondary danalite, bertrandite and gismondine-Ca (Table 4; see also Novák and Filip, Reference Novák and Filip2010; Zachař et al., Reference Zachař, Novák and Škoda2020). Rare chalk-like coatings of secondary bavenite–bohseite also are developed on the surface of crystals or very close (<~1–2 mm) to the crystals of altered beryl (Fig. 2d; Kožichovice II – Novák and Filip, Reference Novák and Filip2010; Zachař et al., Reference Zachař, Novák and Škoda2020). Typical distal fissure-filling assemblages of secondary Be minerals developed on brittle tectonic fractures and fissures have not been found in this pegmatite region.
The following pegmatites contain only proximal bavenite–bohseite. At Ruprechtice, radial aggregates after beryl consist of proximal white bavenite–bohseite and colourless crystals of bertrandite in a mass of dominant secondary fine-grained greenish muscovite–illite + K-feldspar, and rare zeunerite. Bavenite–bohseite and bertrandite are not associated spatially and beryl is present in large relics. At the Kracovice pegmatite, rare grains of beryl are replaced by radial aggregates of bavenite + euhedral bertrandite; rare aggregates of K-feldspar and gismondine-Ca are enclosed in bavenite (Fig. 2f). At the Drahonín IV pegmatite, two distinct proximal secondary bavenite–bohseite assemblages were recognised: (1) rare bavenite–bohseite + euhedral bertrandite in thin veinlets replacing beryl are associated typically with abundant analcime, rare scolecite, laumontite and gismondine-Ca (Fig. 3b), and very rare fluorapatite + chlorite (Table 4); (2) abundant massive aggregates of bavenite–bohseite with euhedral crystals of bertrandite occur in secondary pockets after almost completely dissolved large crystals of beryl (Fig. 3c). Beryl is almost exclusively directly replaced by bavenite–bohseite (Kracovice, Drahonín IV) (Figs 2f, 3b) and bertrandite was only found exceptionally in a direct contact with the replaced beryl.
At the pegmatite outcrops from Dražice, beryl is mostly fresh. It is strongly altered or replaced completely by proximal bavenite–bohseite only if the beryl crystal is cut by or located close (<~5 cm) to brittle tectonic fractures which continue to the host rock. Distal bavenite is developed exclusively on such fractures and is associated with abundant late K-feldspar (adularia). Proximal bavenite–bohseite replaces beryl, whereas euhedral crystals of bertrandite are enclosed in a bavenite matrix. Very rare milarite was found in a small vug close to a distal bavenite + K-feldspar aggregate. In the Věžná I pegmatite, rare crystals and aggregates, <2 mm in size, and fine-grained coatings of bohseite are associated with titanite and chlorite on fractures of blocky K-feldspar + quartz and have no apparent relationship to a possible primary Be-rich precursor (see also Černý, Reference Černý1965).
Rare radial and chalk-like aggregates of proximal bavenite–bohseite are developed after altered crystals of beryl, relics of which are abundant in the Nýznerov pegmatite (Table 4). Volumetrically dominant bavenite–bohseite was observed only locally in the assemblage bertrandite + K-feldspar ± chlorite. In the Maršíkov pegmatites, proximal bavenite–bohseite in pseudomorphs after beryl was found at the Schinderhübel I pegmatite (Fig. 3d). Radial aggregates of chalky white distal bavenite–bohseite on tectonic fractures apparently not associated with other secondary minerals is the most common type (Table 4). They commonly occur between large flakes of primary muscovite at the Schinderhübel I pegmatite (Fig. 3f), whereas the textural relations of bavenite–bohseite with associated albite, muscovite and quartz on fractures are not clear. They are probably fragments of the fracture surface where late bavenite–bohseite crystallised (see also Dolníček et al., Reference Dolníček, Nepejchal and Novák2020); however, the euhedral habit of quartz grains (see Fig. 3e) indicates that it recrystallised during or slightly before the formation of bavenite–bohseite. Only scarcely, is bavenite–bohseite associated closely with Sr-enriched epidote (≤1.75 wt.% SrO) + F-enriched (≤0.45 wt.% F) pumpellyite-(Mg) to pumpellyite-(Fe3+) on Alpine-type hydrothermal veins, ~3 mm thick, cutting a small quartz core at the Schinderhübel I pegmatite (Fig. 3e). Bavenite–bohseite is developed mostly at the external part of the breccia as a matrix of euhedral grains of recrystallised quartz (Fig. 3e) coeval with epidote and pumpellyite.
We also explored the abundance of primary muscovite in the pegmatites as an indicator of the peraluminous nature and acidic conditions in granitic pegmatites (e.g. London, Reference London2008). Primary muscovite is absent in all NYF pegmatites, both elbaite-subtype pegmatites Dražice and Vlastějovice, and it is rare at the Věžná I and Kracovice pegmatites (Table 1). In contrast, it is very abundant at the pegmatites from Maršíkov and it is associated locally with distal bavenite–bohseite (Fig. 3f; Table 4). Primary muscovite is common at Ruprechtice and Nýznerov. Further, the abundance of fluorapatite, a sink for Ca in late stages of pegmatite evolution (Martin and de Vito, Reference Martin and De Vito2014), was investigated. Primary apatite is mostly absent in NYF pegmatites and very rare in a majority of the examined LCT pegmatites; it is common only at the Nýznerov, Věžná I and Scheibengraben pegmatites (Table 1). Very rare, thin veinlets of late fluorapatite associated with proximal bavenite–bohseite + bertrandite were found in the Drahonín IV and Dražice pegmatites (Table 4).
Composition of bavenite–bohseite
Compositions were obtained using electron probe micro-analyses (EPMA), hence, the contents of Be and H2O were calculated from stoichiometry according to the modified procedure of Lussier and Hawthorne (Reference Lussier and Hawthorne2011). The measured concentrations of Al are critical for the discussion, whereas Na, Fe, Mn, P and F are of secondary importance as only trace amounts are usually present (Table 5). Data obtained during our study and from the published papers of Novák and Filip (Reference Novák and Filip2010), Dolníček et al. (Reference Dolníček, Nepejchal and Novák2020) and Zachař et al. (Reference Zachař, Novák and Škoda2020) revealed high variability in Al (and Be) and significant heterogeneity of some samples (Fig. 4). Unfortunately, the similar atomic numbers of the elements in bavenite–bohseite minerals make standard back-scattered electron (BSE) images less useful for the study of zoned structures of bavenite–bohseite crystals.
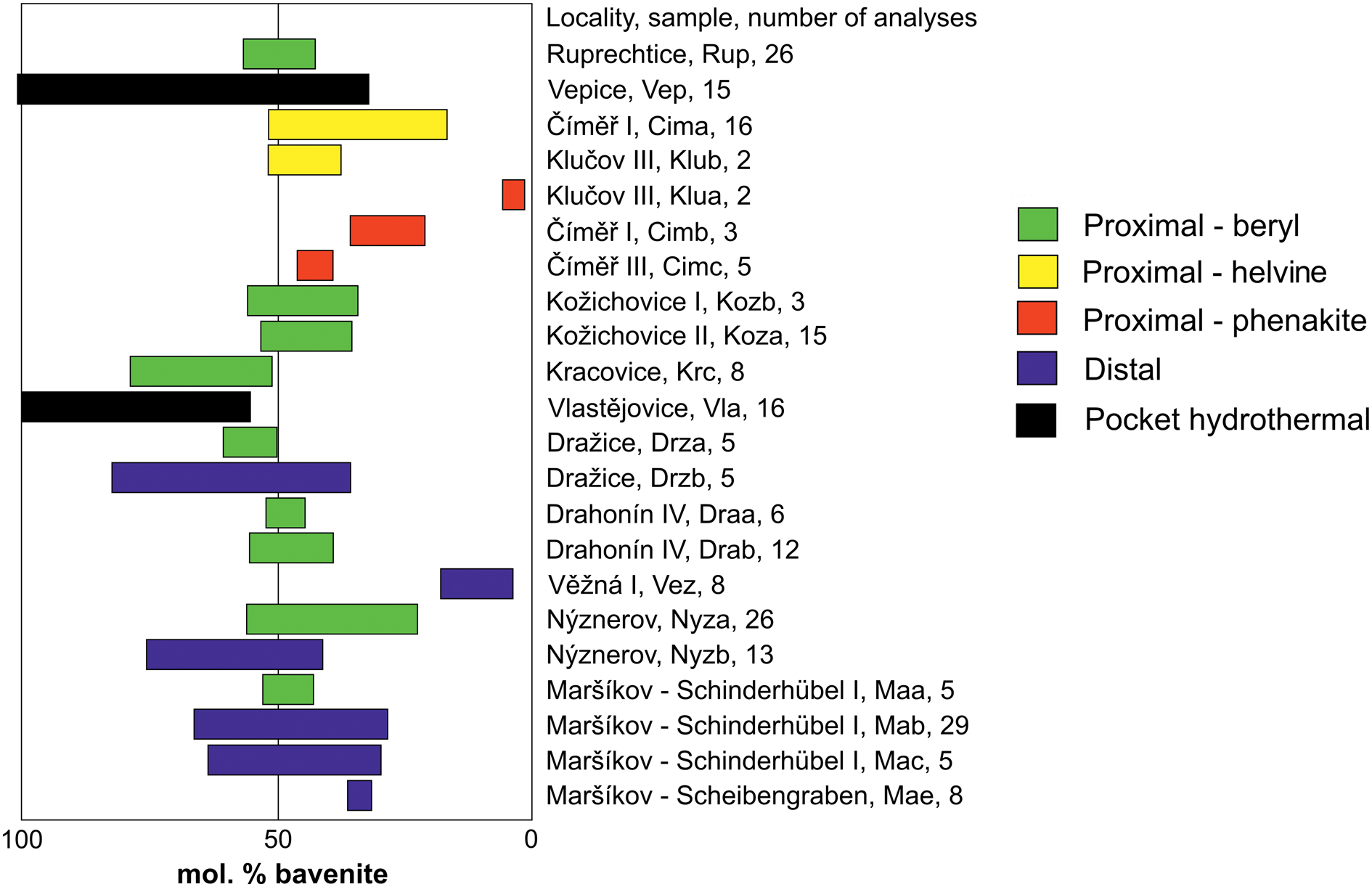
Figure 4. Ranges of bavenite bohseite compositions in individual samples.
Table 5. Representative compositions of bavenite–bohseite.

Sample types: Brl, Hlv, Phk – proximal in pseudomorphs after beryl, helvine and phenakite, respectively; D – distal; PH – pocket hydrothermal.
b.d.l. – below detection limit, * – calculated from stoichiometry.
Two samples of type 1, hydrothermal bavenite and bavenite–bohseite from miarolitic pockets, yielded the highest Al concentrations (all data in this paragraph given in apfu) – Vlastějovice (2.00–1.12) and Vepice IV (2.00–0.67) (Fig. 4). Type 2 proximal bavenite–bohseite is more variable in Al concentrations (1.56–0.05). Solid solutions with dominant bohseite having very low to moderate Al (1.02–0.05) are typical in the pseudomorphs after Al-free precursors phenakite and helvine–danalite whereas in the beryl pseudomorphs bavenite–bohseite has higher Al (1.56–0.69). Type 3, distal bavenite–bohseite, yielded high variability in Al contents (1.63–0.09). Elevated Al (1.63–0.58) is typical for the Schinderhübel I pegmatite associated with muscovite and for bavenite–bohseite from Dražice (1.63–0.96) whereas bohseite from Věžná I is Al-poor (0.34–0.09).
We also focused our study on minor to trace elements, which have not been reported in the majority of the literature cited. Low concentrations of Na (~0.2 wt.% Na2O) are typical in most data (Table 5); however, higher concentrations were found in the distal bohseite from Věžná I (≤0.71 wt.% Na2O; ≤0.20 apfu Na). Proximal bavenite–bohseite from the Třebíč pegmatites also contains minor concentrations of Fe (≤0.59 wt.% FeO, ≤0.07 apfu Fe) in bavenite–bohseite after beryl (Kožichovice I) and in bohseite after helvine–danalite from Číměř I (≤0.74 wt.% FeO, ≤0.10 apfu Fe) and Mn (≤0.81 wt.% MnO, ≤0.10 apfu Mn) in the latter. Concentrations of P close to the detection limit were obtained in the Věžná I (≤0.10 apfu P) and Maršíkov Schinderhübel I (≤0.13 apfu P) pegmatites. Concentrations of F are mostly low (≤0.2 wt.% F); the highest concentration (≤0.74 wt.% F) was found in the proximal bavenite–bohseite from the Nýznerov pegmatite.
The zoned composition of a single crystal within a radial aggregate was examined at several localities where samples suitable for such study were available. Zoning was found in the hydrothermal miarolitic bavenite from Vlastějovice; from 1.30 apfu Al in the base to 1.96 apfu Al in the top of a single platelet. In contrast, at the Ruprechtice and Nýznerov pegmatites, single platelets are almost homogeneous, and no apparent compositional evolution was observed. Hence, the individual crystals of bavenite–bohseite vary from almost homogeneous to highly heterogeneous. The highest variability within the examined sample (several fragments were studied) was observed for the hydrothermal bavenite–bohseite from the miarolitic pocket at Vepice IV (2.00–0.67 Al apfu). Evident zoning is developed in the Alpine-type vein from Schinderhübel I (Fig. 3e) where mono-mineral aggregates of bohseite in quartz are Al-poor (≥0.58 apfu) whereas bavenite–bohseite associated with epidote and pumpellyite in central part of the veinlet is Al enriched (≤1.25 apfu). Also, distal bavenite–bohseite associated with muscovite from the Maršíkov Schinderhübel I pegmatite is heterogeneous (1.31–0.58 Al apfu) as well as distal bavenite–bohseite from Dražice (1.69–0.96 Al apfu).
Raman spectroscopy
Bavenite–bohseite, and some associated primary and secondary minerals, were identified using the Raman spectroscopy (unoriented). Secondary phenakite was not observed in the assemblages of secondary minerals for any of the samples investigated whereas bertrandite was identified in several samples (see Table 4). Here, we present a newly-acquired spectrum of bohseite. Raman spectroscopy was also used to identify minerals of bavenite–bohseite series and to identify eventual structure variations based on compositional changes. For this investigation, three representative samples were selected: a bavenite-rich sample from Vlastějovice; bavenite/bohseite from Ruprechtice; and a bohseite-rich sample from Věžná I (Fig. 4). All samples have similar bavenite spectra (Lafuente et al., Reference Lafuente, Downs, Yang, Stone, Armbruster and Danisi2015) with slight peak shifts and differences; only the bohseite spectrum is contaminated with some kind of fluorescence.
Discussion
Composition of bavenite–bohseite
We focused primarily on the variations in Al content on the basis of a large set of EPMA data (Table 5, Fig 4). The individual paragenetic types have been discussed separately to reveal differences between them and to demonstrate the role of a Be precursor combined with variations in composition of hydrothermal fluids that facilitated crystallisation of bavenite–bohseite with a diverse origin (proximal versus distal). We have also tried to compare the composition of the individual paragenetic types of bavenite–bohseite with the published data. However, this task was very difficult due to frequent absence of information regarding textural and paragenetic positions of the bavenite–bohseite samples analysed with respect to potential or evident primary Be mineral precursors (see De Michele, Reference De Michele1967 and references therein). Furthermore, significant differences in the compositions among bavenite–bohseite samples from the same locality presented by the individual authors (e.g. Fleischer and Switzer, Reference Fleischer and Switzer1953; Lussier and Hawthorne, Reference Lussier and Hawthorne2011) as well as the absence of analysed minor elements (Fe, Mn, P, Na and F) in most published papers partly obscure such discussion.
The samples of hydrothermal bavenite and bavenite–bohseite from miarolitic pockets are typically rich in Al (2.00–0.67 apfu) and very heterogeneous (Fig. 4, Table 5). This paragenetic type differs significantly from the other recognised types of bavenite–bohseite in having high Al contents, however only two examined samples are not sufficient for a comprehensive discussion. Similar miarolitic bavenite to bavenite–bohseite was studied at several localities, e.g. Baveno, Italy (Lussier and Hawthorne, Reference Lussier and Hawthorne2011), Cape Granite Suite, South Africa (Scheepers et al., Reference Scheepers, O'Brien and Schoch2017) and Bustarviejo, Madrid, Spain (Garcia-Guinea et al., Reference Garcia-Guinea, Correcher, Quejido, LaIglesia and Can2005). Their compositions yielded high Al in the Cape Granite (1.82–2.01 apfu) but high variability in Baveno (0.62 and 1.41 apfu; Fleischer and Switzer, Reference Fleischer and Switzer1953; Lussier and Hawthorne, Reference Lussier and Hawthorne2011). In the alkaline pegmatite from Mont Saint-Hilaire, Canada miarolitic bohseite is Al-poor (0.53 apfu Al; Lussier and Hawthorne, Reference Lussier and Hawthorne2011).
Proximal and distal bavenite–bohseite yielded almost the same variations in the concentrations of Al (1.56–0.05 apfu and 1.69–0.09 apfu, respectively; Fig. 4). Bohseite with very low to moderate Al is typical in pseudomorphs after Al-free Be-precursors (phenakite and helvine–danalite), whereas bavenite–bohseite after beryl in LCT pegmatites has higher Al (Fig. 4). The proximal bavenite–bohseite after beryl from the NYF pegmatites Kožichovice I and II with moderate Al (Fig. 4) reflects lower content of Al in primary Mg,Fe-enriched beryl (Table 2) compared to the LCT pegmatites with very low Mg and Fe given above. At the Dražice and Schinderhübel I pegmatites, distal bavenite exhibits higher variation (1.69–0.84 apfu and 1.25–0.66 apfu, respectively) than the proximal one (1.47–1.10 apfu and 1.04–0.94 apfu, respectively). Accordingly, proximal bavenite–bohseite reflects mainly the composition of the precursor whereas compositions of distal bavenite–bohseite are more heterogeneous and could mirror variability in activities of Al and Be in fluids. In addition, associated secondary minerals (e.g. bertrandite) in proximal bavenite–bohseite or Be-free Al-bearing phases (K-feldspar, muscovite, epidote, pumpellyite and zeolites) in both distal and proximal bavenite–bohseite may have controlled concentration of Al in bavenite–bohseite.
Moderate concentrations of Na were found in the distal bohseite from Věžná I (≤0.71 Na2O; ≤0.20 apfu Na) and they are similar to other bavenite–bohseite (Lussier and Hawthorne, Reference Lussier and Hawthorne2011; Szełęg et al., Reference Szełęg, Zuzens, Hawthorne, Pieczka, Szuszkiewicz, Turniak, Nejbert, Ilnicki, Friis, Makovicky, Weller and Lemée-Cailleau2017). Elevated concentrations of Fe and Mn in proximal bavenite–bohseite from the Číměř I pegmatite (Table 5) reflect the composition of the precursor – helvine–danalite (Fig. 3), whereas elevated Fe but no Mn in the proximal bavenite–bohseite after beryl from Kožichovice I mirrors elevated Fe in primary Mn-free beryl (Table 2). Moderate contents of Mg in primary beryl and its absence in the relevant secondary proximal and distal bavenite–bohseite (Fig. 5) as well as in the distal bavenite–bohseite associated with epidote + pumpellyite at the Schinderhübel I pegmatite imply that Mg is very probably not compatible with the crystal structures of bavenite and bohseite. This is also supported by the concentrations of Mg below the detection limit (~400 ppm) in all analysed samples.
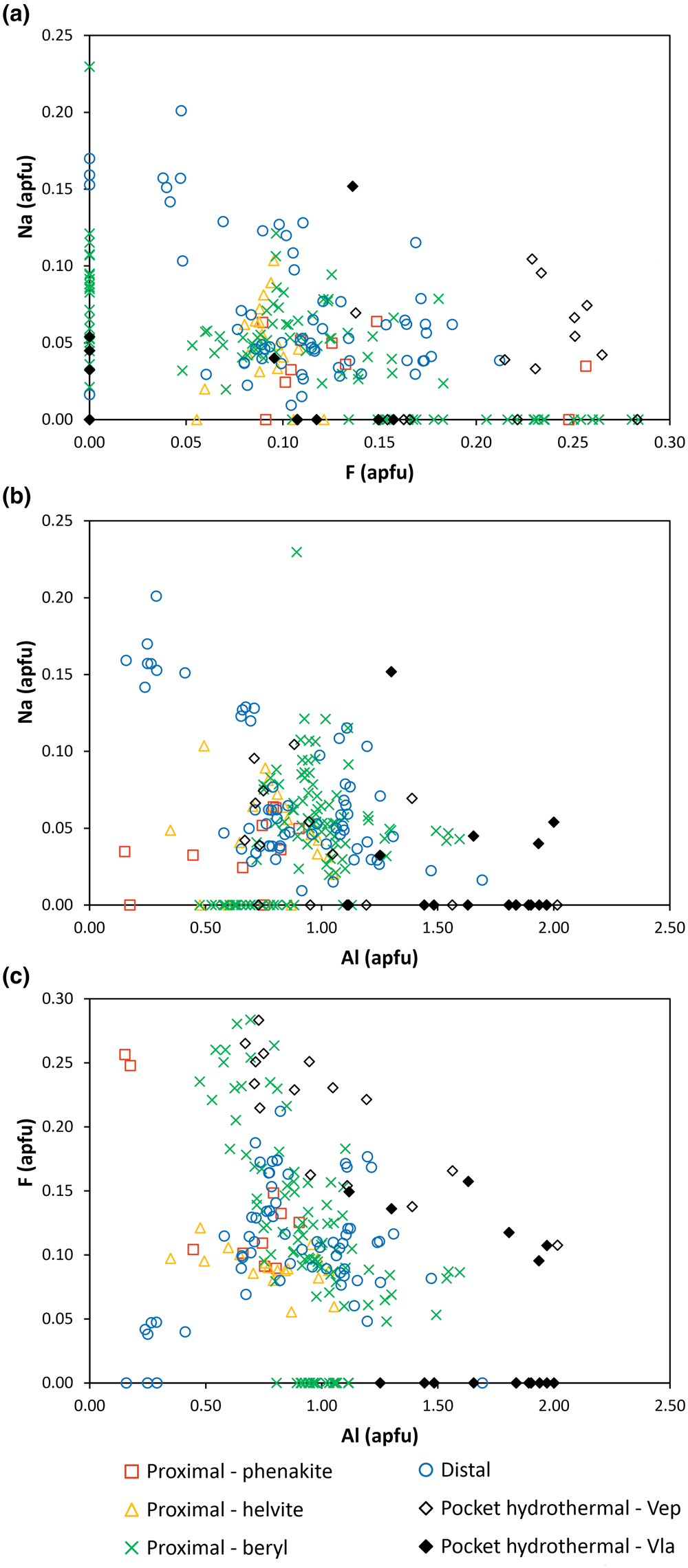
Figure 5. Compositional variation diagrams of bavenite–bohseite. (a) F/Na; (b) Na/Al; and (c) F/Al.
The variability of two most common minor elements Na and F in the individual types of bavenite–bohseite are shown in Fig. 5. Both samples of primary hydrothermal miarolitic bavenite–bohseite differ; bavenite–bohseite from Vepice IV is enriched in F (Fig. 5a,b) whereas bavenite from Vlastějovice is F-poor. The distal type typically contains slightly higher concentrations of Na compared to the proximal type, whereas concentrations of F are similar. The distal type also yields a positive correlation for F/Al whereas primary bavenite–bohseite from Vepice IV has a negative F/Al correlation (Fig. 5c). In summary, differences in the concentrations of Na and F among the individual types of bavenite–bohseite are minor. Because almost all published data included no determination of Fe and Mn and in most cases no F and Na, except for Lussier and Hawthorne (Reference Lussier and Hawthorne2011) and Szełęg et al. (Reference Szełęg, Zuzens, Hawthorne, Pieczka, Szuszkiewicz, Turniak, Nejbert, Ilnicki, Friis, Makovicky, Weller and Lemée-Cailleau2017), a comprehensive comparison with the other analytical data published previously is not desirable.
Raman spectroscopy of bavenite–bohseite
Assignment of bands in the Raman spectra is theoretically possible. Tentative assignments of Raman bands was done based on similarities with different silicate minerals or minerals with beryllate tetrahedra. Raman spectra were compared with cyclosilicates, both single chain and multiple chain inosilicates and framework silicates. A certain resemblance with the spectra of framework silicates was observed, and our results for the bavenite–bohseite series seem to be in agreement with work by Cannillo et al. (Reference Cannillo, Coda and Fagani1966) and later Lussier and Hawthorne (Reference Lussier and Hawthorne2011) who list the minerals of this series as framework silicates.
Spectra in the range of 100–300 cm−1 could be related to lattice vibrations and the area including Raman bands from 300 cm−1 to 600 cm−1 presumably belongs to SiO4 modes combined with Ca–O bonds – their shifts being due to OH− vibration and translation (Fig. 6a). Part of the spectrum from 650 cm−1 to 760 cm−1 probably involves the contribution of the relative motion of the tetrahedrally coordinated Be atoms in adjacent chain links BeO4–SiO4–BeO4 (Prencipe et al., Reference Prencipe, Noel, Civalleri, Roetti and Dovesi2006; Szełęg et al., Reference Szełęg, Zuzens, Hawthorne, Pieczka, Szuszkiewicz, Turniak, Nejbert, Ilnicki, Friis, Makovicky, Weller and Lemée-Cailleau2017).
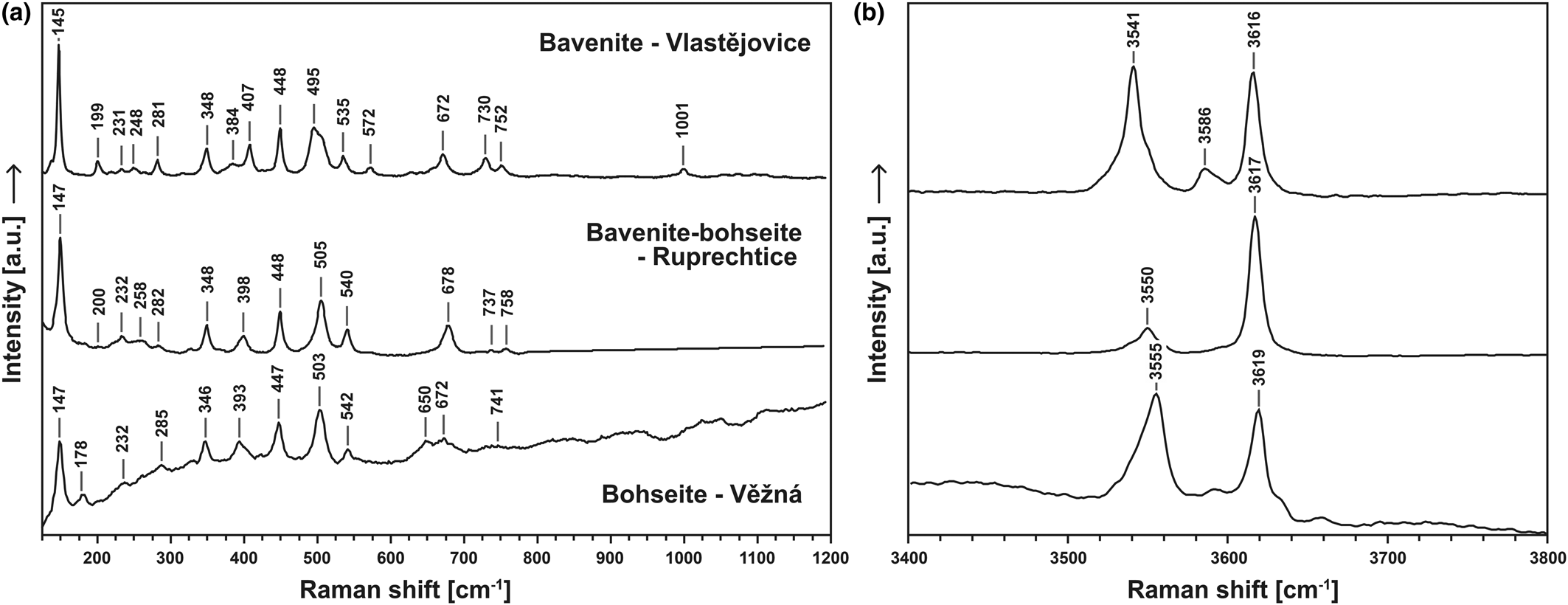
Figure 6. Raman spectra of bavenite, 1:1 bavenite–bohseite and bohseite. (a) spectral range 100–1200 cm–1 and (b) spectral range 3400–3800 cm–1.
Comparison of the Raman spectra in the OH− region with their calculated contents unambiguously shows that the overall composition has a strong influence on the mutual arrangement of these OH− vibrational modes (similar correlation has been observed in tourmalines – see Huy et al., Reference Huy, Nguyen, Chen, Ming and Yang2011). The Raman spectra obtained in the range of 3400–3800 cm−1 is given in Fig. 6b. The vibration at ~3617 cm−1 is relatively consistent, whereas the modes lower than 3555 cm−1 vary in accord with the relative proportions of the bavenite and bohseite components. On the basis of this observation, we can assume that with increasing bohseite component, the distance between the OH− vibrational modes decreases. This result can then be linked to the observations from the crystallographic study of bohseite by Szełęg et al. (Reference Szełęg, Zuzens, Hawthorne, Pieczka, Szuszkiewicz, Turniak, Nejbert, Ilnicki, Friis, Makovicky, Weller and Lemée-Cailleau2017).
Mineral assemblages of bavenite–bohseite
(1) Hydrothermal bavenite and bavenite–bohseite from miarolitic pockets occur in the Vlastějovice and Vepice IV pegmatites where a potential primary Be-precursor is absent (Table 4). The absence of a primary Be mineral in these pegmatites implies that the concentration of Be in melt was not sufficiently high to precipitate beryl (>60 ppm Be in low-T granitic melt: London and Evensen, Reference London, Evensen and Grew2002) or other primary Be mineral. Residual fluids enriched in Be facilitated the origin of bavenite–bohseite as a primary hydrothermal Be mineral in a miarolitic pocket; elevated concentration of Ca in these residual fluids is crucial. The investigated mineral assemblages of hydrothermal bavenite–bohseite are rather similar to other occurrences in miarolitic pockets from intragranitic pegmatites (e.g. Baveno, Italy – Fleischer and Switzer, Reference Fleischer and Switzer1953; Strzegom, Poland – Janeczek, Reference Janeczek1986; Cape Granite Suite, South Africa – Scheepers et al., Reference Scheepers, O'Brien and Schoch2017; Bustarviejo, Madrid, Spain – Garcia-Guinea et al., Reference Garcia-Guinea, Correcher, Quejido, LaIglesia and Can2005), where additional Ca-rich minerals (epidote, fluorite, calcite, prehnite, stilbite, laumontite and axinite) and chlorite are typically associated; however, epidote, fluorite, prehnite, chlorite and zeolites are absent at the examined localities of miarolitic hydrothermal bavenite–bohseite. Only the Vlastějovice pegmatite is evidently LCT affiliated (Novák et al., Reference Novák, Kadlec and Gadas2013) whereas all other pegmatites with miarolitic hydrothermal bavenite–bohseite discussed above are typically NYF affiliated. High activity of Ca in the fluids essential for the origin of hydrothermal bavenite–bohseite has two distinct sources in the examined pegmatites: residual fluids derived from pegmatite in intragranitic NYF pegmatites (e.g. Třebíč Pluton) and pre-emplacement contamination of pegmatite melt from host Fe-skarn at the LCT elbaite pegmatite Vlastějovice (Novák et al., Reference Novák, Kadlec and Gadas2013).
(2) The proximal assemblages after primary Be minerals (Table 4) include several distinct paragenetic and textural types. Total to almost complete bavenite–bohseite pseudomorphs with rare relics of primary Be precursor (beryl) are common at most localities (Ruprechtice, Kracovice, Dražice, Drahonín IV and Schinderhübel I); bavenite–bohseite is commonly associated with bertrandite and locally with K-feldspar, muscovite-illite, gismondine-Ca, analcime, laumontite and/or scolecite (Table 4, Fig. 2,3). Veinlets with bavenite–bohseite as the sole product of replacement of primary phenakite and helvine–danalite are typical in pegmatites of the Třebíč Pluton (Zachař et al., Reference Zachař, Novák and Škoda2020). However, more complex secondary assemblages after beryl were also found in this region: bavenite–bohseite + beryl + bazzite + smectite (Kožichovice II), bohseite + milarite (Číměř I, Kožichovice II), bohseite + bazzite (Kožichovice II), bavenite + clinozoisite (Kožichovice II, Číměř I), bohseite + axinite (Číměř I; Novák and Filip, Reference Novák and Filip2010; Zachař et al., Reference Zachař, Novák and Škoda2020). Bohseite dominates in pseudomorphs after Al-free minerals phenakite and helvine–danalite, and its textures are similar to the proximal bavenite–bohseite after beryl (Fig. 3d). In the Třebíč Pluton, secondary Be minerals occur almost exclusively within pseudomorphs, except for rare bavenite–bohseite on the surface of beryl crystals (Fig. 2d). The total absence of typical secondary distal Be minerals on brittle tectonic fractures and fissures is a distinctive feature of the Třebíč pegmatite region.
(3) The distal bavenite–bohseite is variable in morphology and mineral assemblages. Most brittle fractures and fissures continue to their host rock and are evidently related to tectonism. Externally derived fluids running through fractures were responsible for the alteration of beryl (or other primary Be mineral) and facilitated formation of distal bavenite–bohseite. Bavenite–bohseite in radial aggregates or as fine-grained chalk-like coatings apparently not associated with further secondary minerals is the most common paragenetic type (Table 4). At the Dražice pegmatite, abundant distal bavenite–bohseite typically occurs with K-feldspar. At the Schinderhübel I pegmatite, further minerals occur in a close association with distal bavenite–bohseite: muscovite + albite + quartz or epidote + pumpellyite + quartz (Table 4, Fig. 3e). At the Dražice and Schinderhübel I pegmatites, where both proximal and distal bavenite were identified, proximal bavenite–bohseite has a simpler mineral assemblage compared to the distal bavenite–bohseite which also has more variable composition (Fig. 4). Moreover, distal bavenite–bohseite is not associated with bertrandite even at the localities where abundant bertrandite occurs with the proximal bavenite–bohseite (Table 4).
It is difficult to compare the types of bavenite–bohseite occurrences described in the present study with published reports because the information provided on paragenetic and textural relationships of bavenite–bohseite in these reports was not sufficient to distinguish primary hydrothermal miarolitic, or secondary proximal and distal types (e.g. Fleischer and Switzer, Reference Fleischer and Switzer1953; Janeczek, Reference Janeczek1986; Lussier and Hawthorne, Reference Lussier and Hawthorne2011). Nevertheless, the mineral assemblages observed of proximal and distal bavenite–bohseite are rather different from those described by Černý (Reference Černý1968, Reference Černý and Grew2002). In particular, the widespread proximal assemblage bavenite + bertrandite at almost all localities of LCT pegmatites (Table 4) is remarkable and does not fit with the published observations. According to Černý (Reference Černý and Grew2002), bertrandite is typically associated with muscovite and less commonly with K-feldspar; phenakite and euclase are the only associated secondary Be minerals with bavenite–bohseite. Abundance of K-feldspar and higher variability of the mineral assemblages in distal bavenite–bohseite from the examined pegmatites suggest that bavenite–bohseite and/or bertrandite may be stable over a wider range of P–T–X conditions (see the Discussion below).
Mineral reactions involving bavenite–bohseite
Actual mineral reactions generating bavenite–bohseite from the primary Be-rich precursors (beryl, phenakite and helvine–danalite) are difficult to formulate due to variable compositions of the primary beryl and mainly secondary bavenite–bohseite, in addition to locally complex textural relations in the secondary mineral assemblages. Several idealised reactions involving beryl as a primary Be precursor are presented in Table 6 (#1–5), using the ideal compositions of all involved Be minerals and/or the composition bavenite–bohseite = 1:1.
Table 6. Idealised reactions (1–5) involving beryl as a primary Be precursor using the ideal compositions of all involved Be minerals and/or the composition bavenite–bohseite = 1:1; and reactions (6–8) involving Al-free primary Be minerals – phenakite and helvine–danalite created with bohseite s.s. and bavenite/bohseite = 1/1 as alteration products.

Reactions 1 and 2 illustrate two theoretical settings where Si + Al or only Si are entirely fixed in secondary minerals. Alteration following reaction 1 was observed at the Dražice pegmatite, where released Be and Al facilitated the development of a wide halo of abundant distal bavenite–bohseite on fractures around the altered beryl crystals; the potential excess of Al was incorporated in K-feldspar commonly associated with bavenite–bohseite (Table 4). The assemblage bavenite + bertrandite in pseudomorphs after beryl at Kracovice (Fig. 3f) shows that the modified reaction 3 is close to the real situation at this locality where distal secondary Be minerals are probably absent or very rare (Němec, Reference Němec1990). The modified reactions 3 and 4 illustrate that the proportions of bavenite or bohseite components in the mineral may vary significantly. The more Al is contained in bavenite–bohseite the more modal bertrandite may be present in the assemblage if we assume that no Be was released from the system. At the localities with the assemblage proximal bavenite–bohseite + bertrandite after beryl, higher contents of Al in bavenite–bohseite are typical (e.g. Kracovice; Fig. 2f). More complex proximal assemblages observed in the Třebíč pegmatites including also secondary beryl and bazzite do not change the reaction 4 significantly because beryl is at both sides of the reaction. Scandium in secondary bazzite was supplied by primary Sc-enriched beryl (Novák and Filip, Reference Novák and Filip2010). Secondary milarite Ca2K(Be2Al)Si12O30(H2O) requires elevated K, which is commonly present in fluids manifested at other localities by abundant K-feldspar, muscovite, and/or muscovite–illite (Table 4).
Reactions 6–8 in Table 6, involving Al-free primary Be minerals – phenakite and helvine–danalite were created with bohseite s.s. and bavenite/bohseite = 1/1 as alteration products.
The mineral assemblages observed are mostly simple and contain only bohseite after phenakite with no Be being released during the reaction 6. This is a good fit with the pseudomorphs after phenakite in pegmatites of the Třebíč Pluton where bohseite is a sole proximal secondary Be mineral and distal secondary Be minerals are absent (Table 4, Fig. 2b). In the helvine–danalite pseudomorphs, bohseite is associated locally with rare secondary danalite II (Table 4); however, it modifies reaction 8 only slightly (helvine–danalite is on both sides of the reaction) and low $f_{{\rm O}_2}$ is necessary for the formation of secondary danalite II (Barton and Young, Reference Barton, Young and Grew2002). However, the occurrence of jarosite in pseudomorphs after phenakite described by Zachař (Reference Zachař2021) demonstrates that the released S and M2+ cations facilitated origin of a secondary sulfate mineral at high $f_{{\rm O}_2}$
. These contrasting assemblages (helvine–danalite versus jarosite) manifest high variability of $f_{{\rm O}_2}$
in the fluids which facilitated origin of secondary proximal bohseite at the Třebíč pegmatites.
Significant input of Ca and H2O are necessary in all the reactions and, in addition, input of Si and locally Al is required for some reactions. The beryl-, phenakite- and helvine–danalite-involving reactions differ in the abundance of Al which facilitates origin of Al-rich bavenite and further distal Al-bearing secondary minerals (K-feldspar and muscovite). They are common mostly in distal secondary assemblages after beryl in the LCT pegmatites (Dražice, Schinderhübel I; Table 3), however are mostly absent or rare in the proximal assemblages.
P–T–X conditions of bavenite–bohseite origin
Because Ca is an essential constituent of bavenite and bohseite, these minerals have not been considered in experimental and thermodynamic studies performed in the BASH and Na–BASH systems (e.g. Barton, Reference Barton1986; Barton and Young, Reference Barton, Young and Grew2002; Markl, Reference Markl2001; Wang and Li, Reference Wang and Li2020). Consequently, the P–T–X conditions may be estimated only from the textural relations and associated minerals as was done by Scheepers et al. (Reference Scheepers, O'Brien and Schoch2017) for a miarolitic pocket at the Cape Granite, South Africa. The P–T stability of the associated minerals (stilbite-Ca), fluid-inclusion study, and mainly the chlorite geothermometry suggest T = 210–320°C and P < 2 kbar. At the Vlastějovice pegmatite, the absence of Mg but elevated contents of Sn in coeval axinite-(Mn) indicate that the miarolitic pocket system was closed to the host rock (Fe-skarn) during crystallisation of pocket minerals. The crystallisation of hydrothermal bavenite at rather high T ≈ 300–400°C (London, Reference London2008) is reasonable and it fits very well with the crystallisation T of axinite-(Mn) in miarolitic pockets in the pegmatitic gem-tourmaline deposit, Malkhan, Transbaikalia at 285–415°C (Zagorsky et al., Reference Zagorsky, Peretyazhko and Dmitrieva2016).
Together with bavenite–bohseite as the dominant proximal mineral, bertrandite is widespread in the investigated pseudomorphs after beryl (Table 4). It is stable at T <240°C, P = 100 MPa and low activity of Al (Fig. 7; Barton, Reference Barton1986; Barton and Young, Reference Barton, Young and Grew2002). Formation of bavenite–bohseite at T ≈ 200–300°C is indicated by the presence of coeval bertrandite and zeolites in the proximal bavenite–bohseite assemblages. On the one hand, these zeolites could have crystallised at temperatures as high as 310°C: analcime at T < 300°C (Neuhoff et al., Reference Neuhoff, Hovis, Balassone and Stebbins2004), scolecite at T < 310°C (e.g. Weisenberger, Reference Weisenberger2009) and gismondine-Ca at T = 110–250°C (Ghobarkar and Schäf, Reference Ghobarkar and Schäf1999; Chung et al., Reference Chung, Chen, Lin and Li2002). On the other hand, these zeolites may have crystallised at a much lower temperature, T ≈ 100–150°C (e.g. Weisenberger and Bucher, Reference Weisenberger and Bucher2010). Nevertheless, the presence of epidote + pumpellyite in the assemblage with distal coeval bavenite–bohseite (Fig. 4e) suggests crystallisation at T > ~200–250°C (Frey and Robinson, Reference Frey and Robinson2009; Liou et al., Reference Liou, Kim and Maruyama1983, Reference Liou, Maruyama and Cho1985). Accordingly, the temperature of crystallisation of bavenite–bohseite associated with bertrandite + K-feldspar and with epidote + pumpellyite was estimated at T ≈ 200–300°C and these values are similar to the data of Scheepers et al. (Reference Scheepers, O'Brien and Schoch2017). In summary, bavenite–bohseite crystallised over a temperature range of 400 to ~100–150°C estimated from its coeval crystallisation with zeolites (Weisenberger, Reference Weisenberger2009) and other minerals (Frey and Robinson, Reference Frey and Robinson2009; Liou et al., Reference Liou, Kim and Maruyama1983, Reference Liou, Maruyama and Cho1985).

Figure 7. Beryllium minerals stability as a function of temperature and the activity of alumina (modified from Barton and Young, Reference Barton, Young and Grew2002). Note the small stability field of the assemblage bertrandite + K-feldspar.
The pressure of bavenite–bohseite crystallisation may be estimated from its geological position and mineral assemblages. Hydrothermal bavenite–bohseite from miarolitic pockets is a primary mineral which crystallised in the very early stage of the hydrothermal process of pegmatite consolidation. At the Vlastějovice pegmatite, the pressure of crystallisation in the miarolitic pocket was similar to the pressure during emplacement of rare-element pegmatite at lithostatic P <~ 200–300 MPa (Ackerman et al., Reference Ackerman, Zachariáš and Pudilová2007). Relatively high pressure is indicated for the distal bavenite–bohseite coeval with the assemblage epidote + pumpellyite in the Alpine-type veinlet cutting the quartz core of the Maršíkov Schinderhübel I pegmatite (Table 4, Fig. 3e) where P >~150–200 MPa is a reasonable estimate based on its association with pumpellyite (Frey and Robinson Reference Frey and Robinson2009). In contrast, at some localities secondary proximal bavenite–bohseite is associated with zeolites (Table 4) which typically crystallised at low P <~50–100 MPa or even lower P <~15–20 MPa (Chipera and Apps, Reference Chipera, Apps, Bish and Ming2001; Weisenberger, Reference Weisenberger2009; Weisenberger and Bucher, Reference Weisenberger and Bucher2010) as is indicated by the occurrence of scolecite with the proximal bavenite–bohseite at the Drahonín IV pegmatite. Hydrostatic pressure (see Palinkaš et al., Reference Palinkaš, Wegner, Čobić, Palinkaš, Barreto, Váczi and Bermanec2014) at P < 50 MPa is assumed for fissure-filling secondary distal, and probable for part of secondary proximal, bavenite–bohseite, respectively.
Betrandite is considered to form in acidic to neutral conditions by Černý (Reference Černý and Grew2002); however, associated smectite, adularia, axinite and zeolites (analcime, scolecite, laumontite and gismondine-Ca), are common in the proximal bavenite–bohseite assemblages (Table 4), and indicate instead alkaline to neutral conditions (Hedenquist et al., Reference Hedenquist, Arribas and Gonzalez-Urien2000; Weisenberger and Bucher, Reference Weisenberger and Bucher2010). Moreover, the secondary assemblages epididymite + bertrandite + K-feldspar + muscovite (Novák et al., Reference Novák, Korbel and Odehnal1991) and bertrandite + K-feldspar + magnesio-arfvedsonite (Čopjaková et al., Reference Čopjaková, Prokop, Novák, Losos and Gadas2021), both after beryl from the Věžná I pegmatite, are additional evidence that the stability of bertrandite might be considered as being of moderately alkaline conditions. The results of our study suggest that the stability fields of the relevant Be minerals in P–T–X diagrams require further experimental study (e.g. Wang and Li, Reference Wang and Li2020) as well as thorough study of their mineral assemblages.
Geochemical signature of fluids producing bavenite–bohseite
Secondary minerals generated by hydrothermal alteration of primary minerals are indicators of the composition of hydrothermal fluids in distinct stages of subsolidus evolution of granitic pegmatites. Together with typical cations and anions (see e.g. London, Reference London2008) Ca and Mg also were commonly detected in subsolidus alteration products. Magnesium evidently has an external origin in most cases (e.g. Novák et al., Reference Novák, Selway, Černý, Hawthorne and Ottolini1999, Reference Novák, Škoda, Gadas, Krmíček and Černý2012, Reference Novák, Prokop, Losos and Macek2017b; Čopjaková et al., Reference Čopjaková, Prokop, Novák, Losos and Gadas2021) but an external or internal source of Ca is a matter of discussion (e.g. Teertstra et al., Reference Teertstra, Černý and Ottolini1999; Martin and de Vito, Reference Martin and De Vito2014; Novák et al., Reference Novák, Kadlec and Gadas2013, Reference Novák, Prokop, Losos and Macek2017b; Pieczka et al., Reference Pieczka, Szuskiewicz, Szełeg and Nejbert2019; Zachař et al., Reference Zachař, Novák and Škoda2020; Gadas et al., Reference Gadas, Novák, Vašinová Galiová and Pezzotta2022), and obviously might differ in the individual pegmatites.
The mineral assemblages of hydrothermal bavenite and bavenite–bohseite from miarolitic pockets suggest elevated activities of the same cations (Be, Ca, Si, Al, Mn and Fe) but distinct differences in volatiles in residual fluids (H2O + B) and (H2O + CO2 + S) at the Vlastějovice and Vepice IV pegmatites, respectively, as indicated by their mineral assemblages. Moreover, the occurrence of Sn-enriched axinite-(Mn) from Vlastějovice suggests a more complex composition of residual fluids manifested in a higher degree of fractionation of this Li-bearing pegmatite (Novák et al., Reference Novák, Kadlec and Gadas2013). The mineral assemblages as well as composition of proximal bavenite–bohseite (Table 4) demonstrates high activity of Ca in fluids but locally also elevated activities of K (K-feldspar; Kracovice), Na (analcime; Drahonín IV), and Fe + Mn + B + S (axinite and danalite; Číměř I). In contrast, Si, Fe, Mn and S were derived from a primary Be-precursor (helvine–danalite) in the latter case. The assemblages of distal bavenite–bohseite illustrate the dominance of Ca in fluids together with locally elevated activities of K (K-feldspar, muscovite, muscovite–illite), Na (albite), Mg + Fe (epidote, pumpellyite), and Si (recrystallisation of quartz). Participation of external fluids as sources for Ca, Mg and other cations, derived from the host amphibole gneiss is evident in the distal assemblage bavenite–bohseite + epidote + pumpellyite + quartz from the Maršíkov Schinderhübel I pegmatite. Close spatial relations of bavenite to epidote veins cutting the host pegmatite has also been described from Cava Grignaschi, Italy (De Michele, Reference De Michele1967). The albitisation of early-formed plagioclase from host amphibole gneiss is very likely to be the source of Ca (cf. Martin and de Vito Reference Martin and De Vito2014, Weisenberger and Bucher, Reference Weisenberger and Bucher2011) and is supported by elevated Sr contents of epidote from the Maršíkov, Schinderhübel I pegmatite that was probably derived during albitisation of rock-forming plagioclase from the host metabasite. At the Věžná I pegmatite (Table 1), H2O- and Ca-rich, CO2-poor serpentinite-derived fluids are an important source of Ca (e.g. Palandri and Reed, Reference Palandri and Reed2004; Chavagnac et al., Reference Chavagnac, Monnin, Ceuleneer, Boulart and Hoareau2013; Evans et al., Reference Evans, Hattori and Barronet2013; Čopjaková et al., Reference Čopjaková, Prokop, Novák, Losos and Gadas2021) together with Mg. At NYF pegmatites of the Třebíč Pluton, residual fluids are the most probable source of Ca, manifested not only by abundant proximal secondary bavenite–bohseite but also other late proximal secondary Ca-rich minerals including abundant titanite after ilmenite (Škoda et al., Reference Škoda, Novák and Houzar2006; Zachař and Novák, Reference Zachař and Novák2013), microlite + fersmite + kristiansenite after columbite (Výravský et al., Reference Výravský, Škoda and Novák2019), and high Ca contents in both solidus and subsolidus tourmalines (Novák et al., Reference Novák, Škoda, Filip, Macek and Vaculovič2011).
A high activity of Ca in residual fluids is essential for the origin of hydrothermal bavenite–bohseite in miarolitic pockets. This might be explained by the total absence of apatite in the pegmatite which is a typical sink for Ca in distinct stages of pegmatite evolution (London, Reference London2008; Martin and de Vito, Reference Martin and De Vito2014). All examined localities, except for the Nýznerov, Věžná I and Scheibengraben pegmatites, contain very rare apatite or it is absent (Table 4). Bavenite–bohseite occurs in a variety of NYF and LCT granitic pegmatites (Table 1); however, it is absent at some LCT pegmatites from the region of the Bohemian Massif where rich assemblages of secondary Be minerals were observed. The beryl–columbite pegmatites from the Písek region, Moldanubicum (e.g, Černý et al., Reference Černý, Novák, Chapman and Ferreira2007; Škoda et al., Reference Škoda, Novák and Cícha2011; Švecová et al., Reference Švecová, Čopjaková, Losos, Škoda, Nasdala and Cícha2016) are typical examples with very abundant late fluorapatite in miarolitic pockets, and abundant secondary bertrandite, typically proximal in pockets after dissolved beryl. This is commonly associated with secondary muscovite together with rather rare proximal milarite, secondary beryl, phenakite and danalite (Novák and Cempírek, Reference Novák and Cempírek2010). Notwithstanding the detailed scientific study of these pegmatites, including Be minerals (Vrba, Reference Vrba1895; Sejkora et al., Reference Sejkora, Litochleb, Exnar, Černý and Čech1998; Novák and Cempírek, Reference Novák and Cempírek2010) bavenite–bohseite has not been identified to date (pers. comm. J. Cicha). An absence of Ca-enriched external fluids together with residual fluids depleted in Ca due to sequestration of abundant late fluorapatite at these pegmatites did not allow formation of bavenite–bohseite and demonstrates the importance of apatite abundance in the pegmatite for the availability of Ca in both residual and external fluids.
Conclusions and summary
Bavenite–bohseite was recognised as the most abundant secondary Be mineral in the NYF, mixed and LCT pegmatites of the Bohemian Massif. Three distinct textural and paragenetic types were recognised. (1) Rare primary hydrothermal bavenite–bohseite from miarolitic pockets; (2) proximal secondary bavenite–bohseite in pseudomorphs after primary Be minerals (beryl, helvine–danalite and phenakite); and (3) distal secondary bavenite–bohseite mostly in brittle tectonic fractures and fissures within the host pegmatite. Hydrothermal bavenite–bohseite from miarolitic pockets is typically the only Be mineral in the pegmatite. In contrast, secondary proximal bavenite-bohseite is commonly associated with other secondary Be minerals after beryl – bertrandite, milarite, beryl, danalite and bazzite (see Table 4). We recommend using the terms primary hydrothermal, proximal or distal secondary mineral in the descriptions of bavenite–bohseite and other secondary Be minerals together with a detailed study of actual paragenetic position of secondary Be minerals and their relations to their potential primary Be precursors.
The individual types of bavenite–bohseite differ in their Al contents (Fig. 4). Hydrothermal bavenite to bavenite–bohseite from miarolitic pockets is Al-rich (2.00–0.67 Al apfu); proximal bavenite–bohseite gave variable Al with low contents (1.02–0.05 Al apfu) in pseudomorphs after phenakite and helvine–danalite, and higher contents (1.56–0.69 Al apfu) in pseudomorphs after beryl; distal bavenite–bohseite is the most variable (1.63–0.09 Al apfu) (Table 5, Fig. 4). Proximal bavenite–bohseite reflects mainly the composition of the Be mineral precursor whereas compositions of distal bavenite–bohseite are more heterogeneous and mirror the variability of hydrothermal fluids. The petrogenetic family of the host pegmatite (LCT, NYF) is important only for the composition of primary hydrothermal bavenite–bohseite in pockets. Thus, bavenite is typical for LCT and bohseite for NYF pegmatites, respectively.
The bavenite–bohseite solid solutions range in compositions from Bvn100Bhs0 to Bvn2Bhs98; with compositions close to Bvn50Bhs50 being the most common (Fig. 4). Only traces of Na, F, Fe, Mn and P were detected. Raman spectra in the range of 3400–3800 cm−1 vary chiefly in the vibrational modes lower than 3555 cm−1 in accord with the relative proportions of the bavenite and bohseite components (Fig. 6b). With these modes, the composition of the samples examined can be recognised quite reliably.
Calcium is an essential element together with Be, Si and Al, which were mostly released from primary Be mineral precursors. Presuming an external source for Ca is reasonable in the case of the pegmatite contaminated from a serpentinite host, which supplied Mg and Ca. Similarly, the metamorphic overprint of the pegmatites demonstrated by Alpine-type hydrothermal fissure fillings with epidote ± pumpellyite, albite, and bavenite–bohseite supplied external Ca. For intragranitic NYF pegmatites, residual fluids are the most probable source of Ca as well as in the pegmatite cutting an Fe skarn where external Ca contamination of pegmatite melt proceeded during pre-emplacement and/or post-emplacement stages (Novák, Reference Novák2013; Novák et al., Reference Novák, Kadlec and Gadas2013) before the crystallisation of bavenite and bavenite–bohseite in pockets.
Primary hydrothermal bavenite from miarolitic pockets crystallised at rather high T ≈ 300–400°C, whereas distal Al-poor bohseite is a very late fracture-hosted mineral formed at rather low T ≈ 100–150°C. The PT stability fields of the associated minerals allow us to propose that bavenite–bohseite is stable over a range of pressures from ~15 to ~150 MPa. The mineral assemblages and position of bavenite–bohseite in pegmatite evolution (miarolitic, hydrothermal proximal and distal) indicate that bavenite-dominant compositions may have crystallised at higher temperatures relative to bohseite-dominant ones. However, this must be confirmed by further studies.
Acknowledgements
The authors thank the Principal Editor Roger Mitchell, and reviewers E. Szełęg and P. Uher for constructive criticism that significantly improved the manuscript. This research was supported by OP RDE [grant number CZ.02.1.01/0.0/0.0/16_026/0008459 (Geobarr) from the ERDF] for MN and RŠ. The research was financially supported by the Ministry of Culture of the Czech Republic (long-term project DKRVO 2019-2023/1.II.e; National Museum, 00023272; to ZD and LV).
Competing interests
The authors declare none.