Natural clays used in the manufacture of structural ceramics are weathered products from rocks that crop out at the earth's surface (Vieira & Sanchez Monteiro, Reference Vieira and Sanchez Monteiro2008; Daoudi et al., Reference Daoudi, Elboudour Elidrissi, Saadi, Albizane, Bennazha, Waquif, Elouahabi and Fagel2014). They may remain in situ as residual clays or may be transported and deposited in sedimentary basins as secondary clay deposits (Manning, Reference Manning1995; Christidis, Reference Christidis and Christidis2011) and valleys, resulting in alluvial clays (e.g. Ngon Ngon et al., Reference Ngon Ngon, Etame, Ntamak-Nida, Mbog, Maliengoue Mpondo, Yongue-Fouateu and Bilong2012; Fadil-Djenabou et al., Reference Fadil-Djenabou, Ndjigui and Mbey2014; Ndjigui et al., Reference Ndjigui, Mbey and Nzeukou2016). Optimum conditions necessary for the accumulation of thick, argillaceous sediments occur in warm, humid climates, where slowly rising shield areas bordering basins are drained by rivers at the maturity stage (Ridgeway, Reference Ridgeway1982). Some common clays (alluvial clays) provided the cohesion and workability needed for manufacturing whiteware and earthenware (Wilson, Reference Wilson1998; Reeves et al., Reference Reeves, Sims and Cripps2006). Their optimal exploitation and valorization for use in ceramics require detailed assessment, beginning with the determination of their mineralogical compositions and geochemical properties.
Due to its geographical location and geological context, Cameroon (Central Africa) has widespread clay deposits (Elimbi et al., Reference Elimbi, Yeugouo, Nenwa, Liboum and Njopwouo2003; Nkoumbou et al., Reference Nkoumbou, Villiéras, Barres, Bihannic, Pelletier, Razafitianamaharavo, Metang, Yonta Ngoune, Njopwouo and Yvon2008, Reference Nkoumbou, Njoya, Njoya, Grosbois, Njopwouo, Yvon and Martin2009; Ngon Ngon et al., Reference Ngon Ngon, Yongue-Fouateu, Bitom and Bilong2009; Ekosse, Reference Ekosse2010; Nzeukou et al., Reference Nzeukou, Fagel, Njoya, Beyala Kamgang, Eko Medjo and Chinje Melo2013; Fadil-Djenabou et al., Reference Fadil-Djenabou, Ndjigui and Mbey2014; Tassongwa et al., Reference Tassongwa, Nkoumbou, Njoya, Njoya, Tchop, Yvon and Njopwouo2014; Ndjigui et al., Reference Ndjigui, Mbey and Nzeukou2016). The potential uses of these clay materials include ceramic products such as bricks, tiles, refractories and fine porcelain (Elimbi et al., Reference Elimbi and Njopwouo2002; Kamseu et al., Reference Kamseu, Leonelli, Boccaccini, Veronesi, Miselli, Pellacani and Melo2007; Djangang et al., Reference Djangang, Elimbi, Melo, Nkoumbou, Lecomte, Yvon, Bonnet and Njopwouo2007, Reference Djangang, Elimbi, Lecomte, Nkoumbou, Soro, Blanchart, Bonnet and Njopwouo2008a,Reference Djangang, Elimbi, Lecomte, Soro, Nkoumbou, Yvon, Blanchart and Njopwouob; Pialy et al., Reference Pialy, Tessier Doyen, Njopwouo and Bonnet2009; Diko et al., Reference Diko, Ekosse, Ayonghe and Ntasin2011; Nzeukou et al., Reference Nzeukou, Traina, Medjo, Kamseu, Njoya, Melo, Kamgang, Cloots and Fagel2014; Ndjigui et al., Reference Ndjigui, Ebah Abeng, Ekomane, Nzeukou, Ngo Mandeng and Lindjeck2015). Nevertheless, there are few industrial units in the country; some local producers exist, but their handmade products are generally of poor quality. In fact, raw materials are not always used efficiently in ceramic production. For example, in Foumban (west Cameroon), clay materials were exploited for traditional production in small-scale ceramic factories (pottery, brickworks) and used to build the Foumban Royal Palace in the twentieth century. However, these traditional methods of ceramic production do not take into account the properties of the raw materials. This may explain why parts of the Foumban Palace became damaged and needed restoration. The purposes of this study are to locate common clay occurrences in the broader area of Foumban and to determine their mineralogical compositions and geochemical properties in relation to their suitability for ceramic products.
GEOLOGICAL SETTING
Foumban is part of the Bamoun plateau (average altitude: 1200 m) in western Cameroon, in the central area of the continental part of the Cameroon volcanic line (CVL), which includes plutonic and volcanic rocks (Njonfang et al., Reference Njonfang, Nono, Kamgang, Ngako, Tchoua, Becaluva, Bianchini and Wilson2011). The studied area is made up of a Precambrian–Panafrican basement underlying Eocene basalts (Weecksteen, Reference Weecksteen1957). The main volcanic rocks are fissure-erupted mafic rocks, among which transitional basalts constitute the main lava flows, with some samples dated at 51.8 m.y., the oldest volcanic manifestation found in the CVL (Moundi et al., Reference Moundi, Wandji, Ghogomu, Bardintzeff, Njilah, Foumboure and Ntieche2009). Various mantle sources participated in the formation of the Bamoun lavas (Moundi et al., Reference Moundi, Wandji, Bardinzeff, Menard, Okomo Atouba, Mouncherou, Reusser, Bellon and Tchoua2007), among which is a mantle source similar to that of Mt Cameroon (Okomo Atouba et al., Reference Okomo Atouba, Chazot, Moundi, Agranier, Bellon, Nonmotte, Nzenti and Kankeu2016). The Precambrian–Panafrican basement is made up of gneiss extruded by syntectonic plutonism and affected in the northern part by the Foumban shear zone (Njonfang et al., Reference Njonfang, Moreau and Tchoua1998). The Precambrian granite–gneissic bedrock mainly outcrops in the north of the Bamoun plateau. Transitional basalts outcrop in the western part, near Bangourain and Foumban, while alkaline basalts are observed in the south-eastern part, around Foumban, Koutaba and Foumbot (Wandji, Reference Wandji1995; Moundi et al., Reference Moundi, Ménard, Reusser, Tchoua and Dietrich1996, Reference Moundi, Wandji, Ghogomu, Bardintzeff, Njilah, Foumboure and Ntieche2009). A geological map (Weecksteen, Reference Weecksteen1957) illustrating the main rock types with locations of sampling points is shown in Fig. 1. The significant weathering of the bedrock has formed deep ferrallitic soils. The surface formations in the region are composed of red lateritic or ferrallitic soils in the uplands and hills and hydromorphic soils in the lowlands (Segalen, Reference Segalen1967).
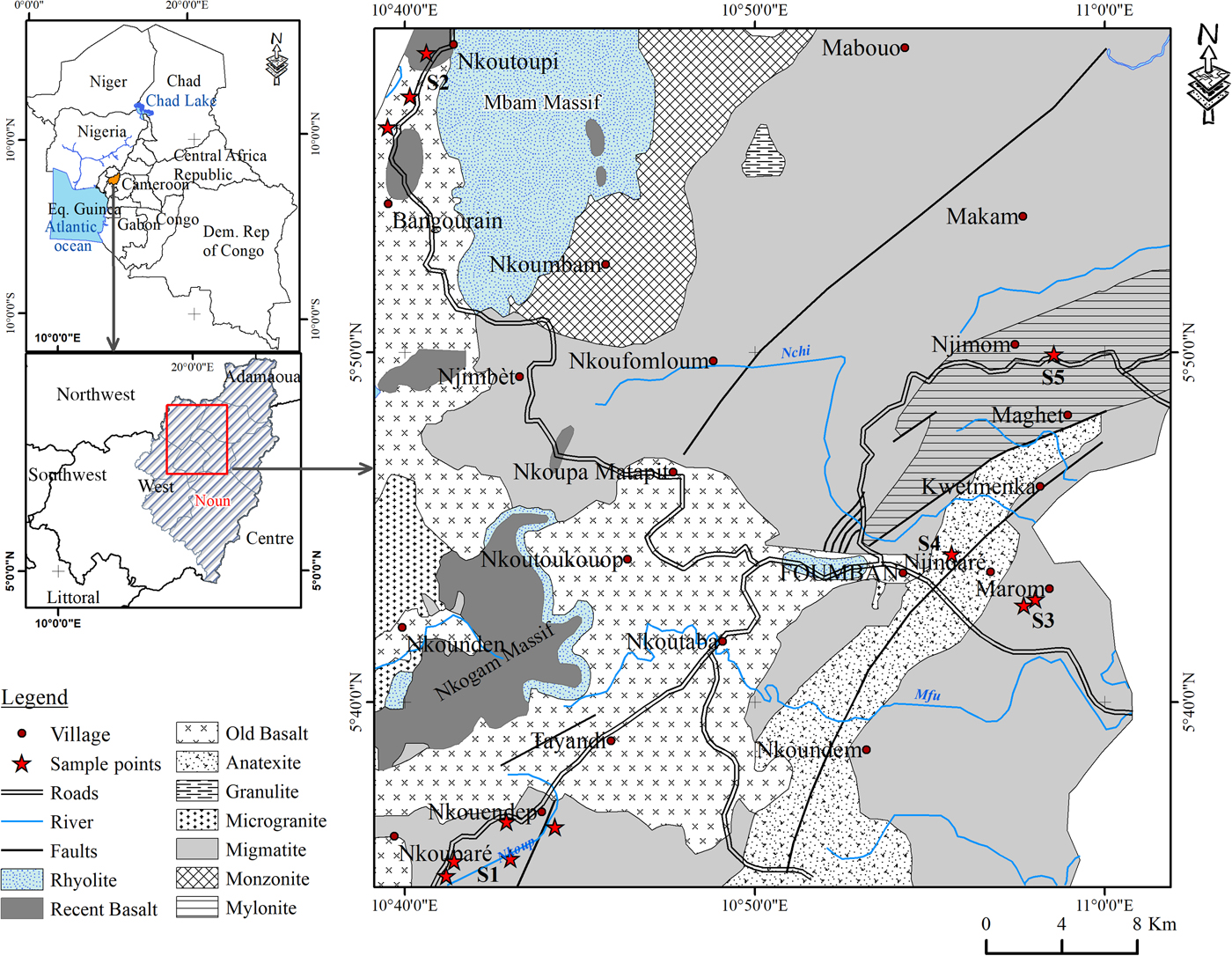
Fig. 1. Geological map of Foumban (after Weecksteen, Reference Weecksteen1957) with locations of sampling points.
MATERIALS AND METHODS
Field campaigns were conducted using classical methods of geological survey to locate and characterize clay materials found in valleys and/or foothills in Koutaba (S1), Bangourain (S2), Marom (S3), Njindare (S4) and Njimom (S5) (Fig. 1). Twenty-six samples of common clays (Table 1) from Koutaba (14), Marom (4), Bangourain (4), Njindare (2) and Njimom (2) were collected. The nature, size, thickness and distribution of the outcrops were determined. The colours of the various profile layers were determined with the Munsell code (Cailleux, Reference Cailleux1992) and the samples were selected according to clay facies variation (texture, colour).
Table 1. Location of sampling points and lithology.

Bulk dried samples (40°C for 24 h) were ground using an agate mortar and sieved to obtain a homogeneous, fine, <250 µm powder used for X-ray diffraction (XRD), X-ray fluorescence (XRF), thermal gravimetric analysis (TGA) and Fourier-transform infrared spectroscopy (FTIR) analyses. Mineralogical analysis was done in the AGES (Argiles, Géochimie et Environnements Sédimentaires) laboratory at the University of Liege in Belgium. X-ray diffraction patterns were determined with a Bruker Advance D8 diffractometer using Cu-Kα radiation, 40 kV and 30 mA. Bulk powders were analysed following the normal procedure as presented by Moore & Reynolds (Reference Moore and Reynolds1997). For clay fractions, oriented aggregates on glass slides were prepared from the <2 µm fraction obtained by suspensions of 1–2 g of the dried bulk sample in distilled water. Three patterns were recorded, under natural conditions (N), after saturation with ethylene glycol (EG) for 22–24 h and after heating (H) at 500°C for 4 h. Minerals were identified using the EVA software. The semi-quantitative mineralogical composition of bulk powder was estimated from the intensity of a diagnostic peak multiplied by a correction factor (Cook et al., Reference Cook, Johnson, Matti, Zemmels and Kaneps1975; Boski et al., Reference Boski, Pessoa, Pedro, Thorez, Dias and Hall1998). For the clay fractions, semi-quantitative estimation was done following the method described by Moore & Reynolds (Reference Moore and Reynolds1997).
Differential TGA was carried out with a SETARAM Cahn apparatus, operating under the following conditions: heating rate of 20°C/min, sample weight of 40 mg, in an air atmosphere from ambient temperature to 1200°C. Infrared spectra were collected on a Bruker FTIR Spectrometer between 4000 and 400 cm−1 to assess the structure and crystal order of the clay minerals. Geochemical analyses of major elements were carried out by XRF spectrometry with an ARL PERFORM-X 4200 XRF spectrometer with a standard error <1% on samples fired at 1000°C. The abundance of major elements was compared with the average compositions of the source rocks.
RESULTS AND DISCUSSION
Location and description of clay materials
At Njimom (18 km north of Foumban) and at Koutaba (27 km south of Foumban; Fig. 1), a thick-layered lateritic cover is observed on dissymmetric hills (Fig. 2a). A vertical cross-section from the top to bottom shows: (1) an organic brown layer; (2) a sandy, pale brown-reddish clayey layer; and (3) a reddish, homogeneous, clayey layer with locally greyish, brownish and purplish varieties; homogeneous, fine and sandy weathering horizon brownish with locally whitish and/or yellowish varieties (Fig. 2b). They are residual primary clays resulting from the weathering of the Panafrican granite–gneiss basement (Nkalih et al., Reference Nkalih Mefire, Njoya, Yongue Fouateu, Tapon Nsandamoun, Nzeukou Nzeugang, Mache, Siniapkine, Flament, Melo Chinje., Ngono and Fagel2015). Close to the study area, Njoya et al. (Reference Njoya, Nkoumbou, Grosbois, Njopwouo, Njoya, Courtin, Yvon and Martin2006) described a kaolin deposit overlain by rolling stones from the mylonitic cliff and by a <1 m thick grey, powdery soil, which is distinct from the red lateritic soil of the surrounding area.

Fig. 2. Description of the clay materials of Foumban. (a) Outcrop of lateritic cover on a hill at Njimom. (b) Vertical cross-section of the clayey laterite. (c) Alluvial clays from Nkoup swampy valley in Koutaba. (d) Vertical cross-section of the clayey hydromorphic materials.
In the lower part of the landscape, the clay materials occur in small and narrow valleys at Marom (5 km east of Foumban) and Njindare (2 km north of Foumban; Fig. 1). They are widespread in the swampy valleys along both margins of the Nkoup river at Koutaba (Fig. 2c), and along the Monoun river at Bangourain (~30 km northwest of Foumban; Fig. 1). The clay profiles are heterogeneous with several clayey and sandy layers of grey, grey-mottled brown and dark brown colours (Fig. 2d). This heterogeneity in colour, texture and thickness characterizes alluvial clays (Ngon Ngon et al., Reference Ngon Ngon, Yongue-Fouateu, Bitom and Bilong2009), with similar field features as the recent alluvial clastic sediments in the Sanaga Maritime region (southern Cameroon) studied by Ndjigui et al. (Reference Ndjigui, Ebah Abeng, Ekomane, Nzeukou, Ngo Mandeng and Lindjeck2015).
Mineralogical composition
Knowledge of the mineralogical and chemical properties of the clayey materials used is required to make ceramic products (Vieira & Sanchez Monteiro, Reference Vieira and Sanchez Monteiro2008; Dondi et al., Reference Daoudi, Elboudour Elidrissi, Saadi, Albizane, Bennazha, Waquif, Elouahabi and Fagel2014). Bulk powder XRD traces of the Foumban clays (Fig. 3a) reveal a mineral assemblage comprising kaolinite, quartz, K-feldspar, plagioclase, smectite, illite, gibbsite, goethite, rutile and hematite. The proportions of the various minerals vary among the study areas and also within the same area (Table 2). Quartz is the most common non-clay mineral with low (<10%) to moderate (21–44%) amounts at Njimom and Marom. K-feldspars and plagioclase are abundant at Marom, Njindare (NJAb) and in two samples from Koutaba (15–27% and 23–26%, respectively). Gibbsite, goethite, rutile and hematite are generally present in minor quantities (<5%). The oriented clay fractions consist of kaolinite, illite and smectite (Fig. 3b); the presence of smectite was verified from the shift of the d 001 spacing from 14.00 Å to 16.66 Å after EG treatment (KG3e).

Fig. 3. XRD patterns of Foumban clays. (a) Bulk powder (KG3e – Koutaba; BA2a – Bangourain; MA3b – Marom; NJAc – Njindare; NJR – Njimom). (b) Oriented clay fraction of the KG3e sample. N: untreated; EG: ethylene glycol solvated; H: heat-treated at 500°C; K: kaolinite; Q: quartz; Fp: K-feldspars; Pl: plagioclase; I: illite; S: smectite; G: goethite; Gi: gibbsite; H: hematite; R: rutile.
Table 2. Mineralogical composition (wt.%) of clay materials from Foumban.

The quantitative mineralogical analysis showed that kaolinite (25–84%) is ubiquitous and illite (0–18%) is present in most of the studied samples (Table 2). Samples from Marom and Njindare are richer in illite (8–18% and 11–17%, respectively). Smectite is mostly present in samples from Koutaba (3–31%) and as a trace phase in samples from Marom and Njimom (~5%). Chlorite appears scarcely in some alluvial clays from Koutaba and Bangourain (3–8%). The clay minerals are abundant in clays from Njimom (85–87%), Bangourain (59–76%), Njindare (63–80%) and Koutaba (35–95%) and low at Marom (22–50%).
The FTIR spectra (Fig. 4) are typical of kaolinite-rich clays. In samples from Koutaba (KG3e), Bangourain (BA2a) and Njimom (NJR), the bands at 3694, 3664, 3650, 3620 and 918 cm−1 are due to the presence of hydroxyl groups (Fialips et al., Reference Fialips, Petit and Decarreau1999). The absence of bands at 3667 and 3652 cm−1 in the KG3e and BA2a samples indicates disordered or poorly crystallized kaolinite; this is further confirmed by the absence of Al2OH bending bands at 938 cm−1 (Cases et al., Reference Cases, Lietard, Yvon and Delon1982). In addition, the FTIR analyses revealed the presence of impurities. The bands at ~2925 (2918) and 2855 (2851) cm−1 correspond to the C–H stretching vibrations related to the organic matter. The presence of quartz in all samples is confirmed by the Si–O symmetrical stretching vibration at ~795 cm−1. The wide band at ~1626 cm−1 is attributed to bending of adsorbed water molecules.

Fig. 4. FTIR spectra of Koutaba (KG3e), Bangourain (BA2a) and Njimom (NJR) samples: (a) complete spectrum; (b) 3800–3400 cm−1 region; (c) 500–1200 cm−1 region.
Two significant weight-loss events on TGA curves are characteristic of kaolinite-rich clays (Fig. 5). The first weight loss below 100°C (78°C, 1–3%) is due to the loss of adsorbed water. The second one between 486 and 500°C corresponds to kaolinite dehydroxylation (5–10%) and is usually indicative of the fraction of kaolinite reacting during firing (Christidis, Reference Christidis and Christidis2011). The minor weight loss (1–3%) between 226 and 266°C might be attributed to the presence of organic matter, goethite and/or gibbsite (Mackenzie, Reference Mackenzie1957), identified by XRD. These observations were confirmed in differential thermal analysis (DTA) curves with two main endothermic peaks at 104–115°C and 486–500°C, respectively. The first endothermic peak corresponds to the dehydration and the second to the dehydroxylation of kaolinite and its transformation into meta-kaolinite.

Fig. 5. TG/DTA curves of the clay samples from: (a) Koutaba and (b) Bangourain.
Geochemistry
Table 3 presents the major chemical composition of the clay samples. The most abundant oxides are SiO2 (45–71%), Al2O3 (14–31%) and Fe2O3 (1–12%), with high Fe contents (˃8%) in some samples from Bangourain. The TiO2, MnO, MgO, CaO, Na2O and P2O5 contents are generally low, with K2O (2–6%) being more abundant. The relatively high K2O content in samples from Marom and Njindare is due to the presence of K-feldspars and illite. Some samples have high Al2O3 content (>22%) in accordance with their high kaolinite content. The low SiO2/Al2O3 ratio (~2) in the Koutaba, Bangourain and Njimom clay samples is also compatible with the high kaolinite content in these samples. In contrast, the high SiO2/Al2O3 ratio (3–5) in the Marom and Njindare samples is controlled by the quartz content. The mixture of mainly kaolinite and quartz in the clays studied might be compared to that of the Romana kaolinitic materials (Ligas et al., Reference Ligas, Vras, Dondi and Marsigli1997). The loss of ignition (LOI) ranging from 3% to 9% in Marom and some Njindare clays and of >10% in other areas is associated with the loss of structural water in clay minerals, as was confirmed by thermal analysis and XRD. The relatively high LOI in some samples (Table 4) might also be attributed to the presence of volatile organic components or smectite (KG3e and KG3c).
Table 3. Chemical composition (wt.%) of clay materials from Foumban.

CIA = Chemical index of alteration; S/A = SiO2/Al2O3.
Table 4. Chemical composition ranges (%) of Foumban common clays compared with chemical compositions of other standard clays.

a Etruria Marl Staffordshire (Ridgeway, Reference Ridgeway1982).
b Ball clays (Group 4 Devon; Watts Blake Bearne technical literature).
c Composition range for the raw materials of fired bricks and tiles in France (Kormann & Ingénieurs du Centre Technique des Tuiles et Briques, Reference Kornmann2005).
d Composition range for the raw materials of fired bricks in Italy (Kormann & Ingénieurs du Centre Technique des Tuiles et Briques, Reference Kornmann2005).
The Chemical Index of Alteration (CIA; Nesbitt & Young, Reference Nesbitt and Young1984) ranges from 80 to 100 (Table 3), except for MA2d (63) and MA1e (70) in Marom clays. The high CIA values for Foumban clay deposits are due to their enrichment in kaolinite crystallized during intense chemical weathering (Goldberg & Humayun, Reference Goldberg and Humayun2010). The lower CIA for Marom clays and the high K2O contents are related to the occurrence of K-feldspars. The relatively low CIA values confirm moderate weathering of sediments (Ndjigui et al., Reference Ndjigui, Ebah Abeng, Ekomane, Nzeukou, Ngo Mandeng and Lindjeck2015).
Principal component analysis and genesis of clay materials
The chemistry and mineralogical composition of clays depend on the origin of clay (hydrothermal, residual or alluvial), the nature of the parent rocks, topography and climatic conditions. Figure 6 presents the results of a principal component analysis (PCA) for the major element data obtained from the analyses of clays from Foumban. The two factors F1 and F2 describe 47.6% and 19.6% of the total variance, respectively. High positive F1 correlation indicates high amounts of TiO2, Al2O3, Fe2O3, MnO, MgO and P2O5 and might be due to the high proportion of clay minerals, rutile and Fe and Mn oxides. The negative F1 scores of SiO2 and K2O may be due to the relatively abundant quartz, K-feldspar and plagioclase. The positive F2 correlation between MgO and CaO probably reflects the abundance of smectite. The projection diagram F1–F2 shows three separate fields corresponding to the three mentioned geochemical groups. Based on the PCA, two groups of common clays are identified. The first group, with SiO2, Na2O and K2O, derives from felsic minerals (quartz, feldspar) through the weathering of granite or rhyolite. All the Foumban common clays, except some from Njimom and Koutaba, are weathered products of granites or rhyolites. The kaolinite and quartz contents support their relationship with acidic rocks (Velde & Meunier, Reference Velde and Meunier2008). The relatively high Fe2O3 and MnO contents in some samples from Koutaba and Njimom might relate their source to a mafic rock, like basalt. The TiO2/Al2O3 binary diagram of Ekosse et al. (Reference Ekosse2001) was used to identify the source rocks of the clays (Fig. 7). The Foumban common clays dominantly indicate strong acidic affinities from rhyolitic or granitic source rocks with a minor basaltic component.

Fig. 6. Principal component analysis diagram of the geochemical data from the studied clays.

Fig. 7. Projection of studied samples on the TiO2/Al2O3 binary diagram (after Ekosse et al., Reference Ekosse2001).
The major-element contents of the common clays were compared with those of average composition in the regional potential source rocks (e.g. Mekwene-Njimafofire Panafrican granitoids: Nzina et al., Reference Nzina, Nzenti, Njiosseu Tanko, Ganno and Ngnotue2010; Mount Mbapit rhyolite: Wandji et al., Reference Wandji, Tchokona Seuwui, Bardintzeff, Bellon and Platevo2008; Bamoun plateau Eocene basalts: Moundi et al., Reference Moundi, Wandji, Bardinzeff, Menard, Okomo Atouba, Mouncherou, Reusser, Bellon and Tchoua2007; and Njimom mylonite: Njonfang et al., Reference Njonfang, Moreau and Tchoua1998). Granitic and basaltic rocks are easily and rapidly weathered to clay minerals and quartz with high rainfall and a warm climate on gentle slopes with a fluctuating water table and rapid water percolation (Murray & Keller, Reference Murray, Keller, Murray, Bundy and Harvey1993). This is characteristic of the formation of the ferrallitic soils that cover the entire humid tropical zone (Tardy & Roquin, Reference Tardy and Roquin1998). Kaolinite clays could have been chemically weathered from primary minerals, particularly feldspars. These weathered materials are further transported either to the river margins of Nkoup and Monoun for Koutaba and Bangourain, respectively, or to the bottom of the hill at Njindare and Marom, where significant amounts are accumulated. Smectite forms either from the chemical weathering of basalts or as a neoformation product in environments with limited drainage. Illite comes from the transformation by aggradation of mica (Reeves et al., Reference Reeves, Sims and Cripps2006). The relatively high proportions of K-feldspars and plagioclase of Marom and some Njindare and Koutaba clay materials might be due to the moderate weathering of the source rocks, as observed in immature recent sediments (Israde-Alcántara et al., Reference Israde-Alcántara, Robles-Camacho and Domínguez2008).
Suitability for ceramic products
The mineralogical association of Foumban clays, mainly represented by kaolinite, illite and quartz, is suitable for ceramic application. In ceramic building materials, kaolinite might provide sufficient strength, plasticity and refractoriness. Illite may promote the vitrification that is responsible for the densification of the final product, while quartz prevents cracking, shrinking and warping and also provides a uniform shape to the final product (Rajput, Reference Rajput2004). The presence of a relatively large smectite content (>5%) limits the application of some Koutaba clays for ceramic building materials.
Figure 8 suggests three main areas for the clay materials studied: (1) the quartz-rich field (sample MA1e with 71.07 wt.% SiO2); (2) the average quartz-rich field (Marom samples MA3c, MA3b and MA2d and sample KB3c from Koutaba); (3) and the clay-rich field (most samples from Koutaba, Bangourain, Njindare and Njimom). In view of the very high sand content of sample MA1e, sintering might occur in the temperature range of fired bricks and tiles (850–1050°C). Therefore, it might be preferable to use this sample as a degreaser for ceramic pastes. The remaining samples from Marom (MA3c, MA3b and MA2d) have relatively low contents of clay minerals, suggesting more porous matrices, and therefore they may not be suitable for tiles and fired bricks. Their iron oxides content (1.58–4.83%) and alkalis (3.94–5.01%) is an indication of their sintering temperature, which should be relatively low (cf. thermal analysis curves). Samples from Koutaba, Bangourain, Njindare and Njimom are very rich in clay minerals. Apart from samples KF5c, KF5d, KC4f, KC4d, KC4b, NJAc, NJJ and NJR, the iron oxides content and alkali elements is generally ≥10 wt.%, which guarantees a low vitrification temperature and a low porosity. Indeed, the presence of Fe in clay minerals is generally associated with octahedral and even tetrahedral substitution, which affects the structure and degree of crystal order, favouring a low sintering temperature. Those of the samples that have a percentage of Al2O3 ≥25 wt.% with a low Fe content and alkali elements might be suitable for refractories. This interpretation is in line with the mineralogy and the thermal properties of the clay materials studied.

Fig. 8. Ternary diagram: quartz/carbonates + Fe-oxides + accessories + feldspars/clay minerals for the Foumban common clays, after Fiori et al. (Reference Fiori, Fabbri, Donati and Venturi1989).
Table 4 shows the chemical composition of the studied samples in comparison with the composition range of clay materials used in fired bricks and tiles in France and Italy (Kornmann & Ingénieurs du Centre Technique des Tuiles et Briques, Reference Kornmann2005). The chemical compositions of the studied clays are within the range limits for French bricks and tiles. However, the proportions of SiO2 in some Marom and Koutaba clays are higher than those used for Italian bricks. The composition of Njimom clays and some Koutaba clays is close to that used for sanitary ware and Al–Si refractories. They might be used after removal of TiO2 and Fe2O3 (Murray & Keller, Reference Murray, Keller, Murray, Bundy and Harvey1993). The relatively high Fe content (>1%) in most of the studied clays suggests a light to red colour of fired products. The alkali and alkaline earth contents are responsible for the vitrification and therefore the ability to provide sufficient porosity, permeability and mechanical strength. Figure 9 compares the bulk major-element composition of Foumban common clays with those used for red stoneware in Italy and white stoneware in German, English and French industries, as given in the ternary diagram for SiO2–Al2O3–remaining oxides proposed by Fabbri & Fiori (Reference Fabbri and Fiori1985). In this diagram, all clays lie out of the red stoneware field and most studied clays plot in the white body field. Some Koutaba and Bangourain clays have very low SiO2 contents in comparison with the optimal value for any white body products (i.e. 72 wt.%; Fiori et al., Reference Fiori, Fabbri, Donati and Venturi1989). Those samples require addition of SiO2 for application in white bodies.

Fig. 9. Ternary diagram of Foumban clays: SiO2/Al2O3/other oxides. a = red stoneware (Italy); b, b′, b″ = white stoneware for German, English and French industries, respectively (data are from Fabbri & Fiori, Reference Fabbri and Fiori1985).
CONCLUSIONS
The Foumban area, Cameroon, was surveyed in order to locate, characterize and determine the genesis of clayey materials with the principal objective of evaluating their potential for use in ceramic industries. The Foumban common clays occur as grey, grey-brown, grey-greenish to dark grey, brownish, mottled clays dominated by the clay size fractions. Most of these materials are alluvial clays formed by weathering, transport and accumulation at the lower part of the landscape. They are composed of kaolinite, illite, smectite and chlorite, with minor quartz, K-feldspars, plagioclase, goethite and traces of rutile and hematite. Kaolinite dominates the <2 µm fractions. Geochemical data suggest felsic rocks as the main parent rocks for common clays from Foumban. Based on their mineralogical and geochemical characteristics, they might be suitable for ceramic products. The proportion of alkali and alkaline earth elements in Marom clays (6–9%) would enable lower firing temperatures. Some Bangourain clays with high Fe2O3 contents (>11 wt.%) might require processing prior to use. In addition, clays with >25 wt.% Al2O3 might be suitable as fireclays after beneficiation.
ACKNOWLEDGMENTS
This study was carried out within the framework of a research and development project funded by ARES-CCD/PRD ‘Caractérisation et valorisation des Matériaux Argileux de Foumban (Ouest-Cameroun) – MAFO’ of the Académie de Recherche et d'Enseignement Supérieur (ARES-CCD), Belgium. The authors are grateful to this institution for financial support.