Introduction
Cystic echinococcosis (CE) is a parasitic zoonosis caused by the Echinococcus granulosus cestode with intermediate (sheep and other domestic livestock, omnivores and lagomorphs) and final (carnivores, usually dogs) hosts. The parasite eggs are released into the environment in the faeces of the definitive host and contaminate the soil and water. Consequently, the environment represents a potential source of infection for humans (Shaikenov et al., Reference Shaikenov2004; Umhang et al., Reference Umhang2017).
This zoonosis is endemic in many South American countries (Cucher et al., Reference Cucher2016), and it is a major public health and economic problem in the Patagonian region of Argentina (Pierangeli et al., Reference Pierangeli2007).
To date, most studies modelling Echinococcus spp. transmission have focused on determining the influence of environmental parameters on the risk of infection with E. multilocularis, causal agent of alveolar echinococcosis (AE) (Veit et al., Reference Veit1995; Giraudoux et al., Reference Giraudoux2003, Reference Giraudoux2013; Danson et al., Reference Danson2004; Guislain et al., Reference Guislain2007, Reference Guislain2008; Burlet et al., Reference Burlet, Deplazes and Hegglin2011). Based on these studies, Atkinson et al. (Reference Atkinson2013) proposed that the transmission of echinococcosis could be influenced by climate change and anthropogenic factors through changes in animal population dynamics, spatial overlap of competent hosts, and the creation of favourable weather conditions for egg survival and dispersion.
In terms of the definitive host, an association of CE risk infection with free-roaming dog movements has been suggested by some authors (Moro et al., Reference Moro2004; Van Kesteren et al., Reference Van Kesteren2013). In addition to this, other authors, including Vaniscotte et al. (Reference Vaniscotte2011), have proposed a relationship between the behavioural pattern of foxes and the risk of E. multilocularis. For instance, AE might have been introduced to Hokkaido from Kunashiri Island, which is located 15 km off the north-eastern coast of Hokkaido, through migration of infected foxes during the winter (Satoh et al., Reference Satoh2005). Other than the above-mentioned studies, there has been virtually no research on the influence of definitive host behaviour on the dispersion of Echinococcus eggs.
Furthermore, to the best of our knowledge, over the last 27 years only two studies have provided direct evidence of interaction between bioclimatic conditions and E. granulosus egg distribution (Wachira et al., Reference Wachira, Macpherson and Gathuma1991; Chaâbane-Banaoues et al., Reference Chaâbane-Banaoues2015). This lack of information becomes relevant when we consider the heterogeneous distribution of the disease in the world. Besides, the latest reviews on this subject have recognized that numerous studies have been performed on drivers of environmental distribution of E. multilocularis eggs, yet they found an absence of empirical evidence on the same matter in E. granulosus eggs (Burlet et al., Reference Burlet, Deplazes and Hegglin2011; Atkinson et al., Reference Atkinson2012, Reference Atkinson2013). This gap encouraged us to publish our findings, and our study suggests an exploratory working model that could be a starting point for further research on spatial investigations in CE. We carried out this study to add evidence about E. granulosus egg occurrence under Patagonian environmental conditions with the aim of testing the hypothesis that in this region the persistence and dispersion of the parasite eggs can be explained by parameters such as landscape attributes, meteorological conditions and dog infection behaviour, and worm burden status.
Materials and methods
Setting and background
The investigation was carried out at the Hydatidosis Control Program HQ located in the village of Sarmiento (Province of Chubut, Argentina, 45°36′S, 69°5′W, 350 m above sea level). For the sake of background, the first trial to test the EG95 vaccine's effectiveness in Argentina (Lightowlers et al., Reference Lightowlers1999) was carried out here. For the purpose of this study, four dogs were dewormed with Praziquantel (5 mg/kg) and Albendazol (50 mg/kg). The dogs were kept in individual kennels in a restricted-access enclosure of 10,000 m2 under stringent conditions, and fed with balanced food and potable water. One month after deworming and following a fast of 24 hours, two of the dogs were infected with approximately 80,000 protoscoleces recovered from lung and liver hydatid cysts obtained from slaughtered sheep in the Sarmiento abattoir (Chubut, Argentina), following García Llamazares et al. (Reference García Llamazares1997). The viability of protoscoleces was assessed by their flame cell activity, motility and ability to exclude 0.32% of Tripan Blue. Six months later, in order to determine the parasitic burden, dogs were dosed with 4 mg/kg of arecoline hydrobromide according to standard procedures (Schantz, Reference Schantz1973). The infected dogs harboured a parasitic burden ranging from 100 to 1000 mature adults of E. granulosus, as indicated by counts and optical microscopy, whereas the uninfected dogs yielded negative to parasites. The viability of the parasite eggs and the genotype of E. granulosus G1 sl has been described elsewhere (Sánchez Thevenet et al., Reference Sánchez Thevenet2005). The present longitudinal study started just after the four dogs—two of them infected and two uninfected—were removed from the enclosures. We then carried out our observational follow-up research over five subsequent years. As the study site was a restricted-access area, there were no other free-roaming dogs or animals within the enclosures.
Landscape characteristic and dispersion studies
The study site covers 10,000 m2. The vegetation is sparse and includes low bushes and natural pastures. A low-depth natural pond c. 10 m2 in size is located in the south-east sector. For the purpose of the model, the area was divided into subareas, depending on dog occupation. These subareas were identified as follows:
P (n = 4): plots occupied by every dog
A (n = 4): adjacent sectors to P, with a width of 10 m
M (n = 2): middle-distance sectors located 10–100 m from P plots
D (n = 4): distant sectors located > 100 m from P plots
Dogs were not allowed in subareas A, M and D.
Faecal samples
The original canine faeces remained outdoors, deposited onto the surface stratum of soil and exposed to natural environmental conditions over 70 months in the study site. Dog faeces were screened for E. granulosus eggs and for Echinococcus spp. coproantigen at 0 (T0), 41 (T41), 70 (T70) and 84 (T84) months of exposure under natural environmental conditions. Each faecal sample was composed of five individual scats collected from P plots occupied by infected or uninfected dogs. Each composite sample was divided into two aliquots, one of which was preserved at 4°C in 10% formaldehyde for coprological studies, and the other frozen at −70°C for 72 h to render E. granulosus eggs non-infective for coproantigen studies. Egg examination was carried out by standard ether-formalin-sedimentation and by centrifugal flotation techniques using a sucrose solution with a specific gravity of 1.27. Each sample was examined microscopically at 10× in triplicate and at 40× magnification under a Leica DM500 microscope. Echinococcus spp. coproantigen detection was carried out by means of a sandwich enzyme-linked inmunosorbent analysis (ELISA) as developed by Allan et al. (Reference Allan1992). Samples were first inactivated at −70°C for 72 h for coproantigen detection, as mentioned above, and then left to hydrate for 24 h after the addition of an equal volume of 0.3% (v/v) Tween in phosphate-buffered saline. After a final 30-minute sedimentation at 5135 × g, we stored 2.0 ml aliquants of the resulting supernatant at −20°C until further processing. The cut-off value for the ELISA capture test was 0.215OD ± 3 SD.
Environmental samples
Samples of topsoil, sludge sediment and water from the natural pond were analysed at each above-mentioned T0 to T84 month follow-up time.
Topsoil specimens were collected by means of random conglomerate sampling methods on the basis of the quadrant division scheme shown in fig. 1. From each quadrant we transferred five sub-fractions of approximately 50 g of surface soil within a 10 cm2 area 5 cm deep to a resealable polyethylene bag and kept the samples at 4°C until processing. Thus, a mean sample (from five component samples taken from each quadrant) was designed for parasitological examinations. A mean sample of sludge sediment was also gathered from the bottom of a natural pond by dragging from five different sectors. Following Sánchez Thevenet et al. (Reference Sánchez Thevenet2004) and as a pretreatment, the mean topsoil and sludge sediment samples were ground and homogenized and a 2 g aliquant was sieved and dried in an oven at 25°C for 24 h. The moisture-free material was then processed using the methods of Dada and Lindquist (Reference Dada and Lindquist1979). Each sample was examined microscopically at 10× in triplicate and at 40× magnification under a Leica DM500 microscope. Aliquots of sieved material were preserved at −20°C and the genus-specific Echinococcus spp. antigen was detected by Western Blot following Guarnera et al. (Reference Guarnera2000).

Fig. 1. Schematic representation of the sampling design. Filled grey rectangles indicate plots occupied by dogs for six months. The diagram is not to scale. Numbers 1 to 50: quadrants of topsoil sampling. Quadrants 1–12: plots occupied by uninfected dogs. Quadrants 13–18 and 19–24: plots occupied by infected dogs with a worm burden of approximately of 1000 and 100 adults of E. granulosus, respectively. Quadrants 25–43: adjacent zone (A). Quadrants 44 and 45: medium zone (M). Quadrants 47–50: distant zone (D). 46: natural pond; w: predominant wind direction.
The water sample was collected from the pond surface in 8 l sterile polyethylene containers and stored, protected from light, at room temperature until processing. The water was centrifuged for 10 minutes at 1500 rpm to obtain a concentrate from a water sample of relevant size. The concentrate obtained was then processed by Willis flotation and Telemann sedimentation methods and the procedure monitored concurrently in triplicate by light microscopy at 10× and at 40× magnification using the Leica DM500 microscope.
Meteorological conditions
Throughout the period studied the climate corresponded to the inferior arid category, which is characterized by great thermal amplitude, with warm summers and cold winters with frequent frosts. The weather data were obtained from the Instituto Nacional de Tecnología Agropecuaria local meteorological station at Sarmiento-EEA (Chubut Province, Argentina). The temperature range over the period studied was −3 to + 37°C, with a mean annual temperature of + 10°C. There were also average winds and a low level of precipitation, under 300 mm/year.
Data analysis, biosafety regulations and ethical aspects
Double blind methods were applied to prevent biases during the sample examinations. Frequency distribution and summary measures were also used. Biosecurity measures were applied for studies of Echinococcus spp. eggs in accordance with Eckert et al. (Reference Eckert2001) in all instances of the study. The study was accepted and approved by the Postgraduate Committee of the Faculty of Natural Sciences of the National University of the Patagonia, San Juan Bosco (Argentina).
Results
A total of 224 composite samples were analysed during the whole period studied: 16 dog faeces, 200 topsoil, four sediments and four water samples.
In faeces from infected dogs, E. granulosus eggs were observed from T0 up to T41, whereas the coproantigen test remained positive up to T70. Table 1 summarizes the parasitological and coproantigen results in the faecal samples.
Table 1. Echinococcus granulosus eggs and Echinococcus coproantigen presence in dog faecal samples exposed at different times under natural environmental conditions in Sarmiento (Chubut province, Patagonia, Argentina), by dog infection status.
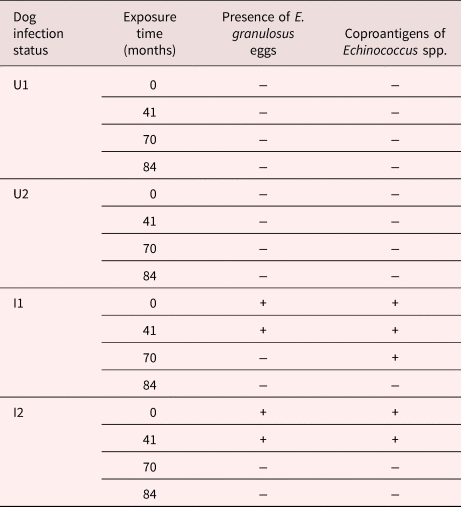
UI and U2: uninfected dogs (controls)
I1: infected dog with a worm burden of approximately 1000 adults of E. granulosus
I2: infected dog with a worm burden of approximately 100 adults of E. granulosus
In total, 20/200 topsoil samples resulted positive for taeniid-E. granulosus-like eggs during the whole study period (table 2): 11/50 at T0, 9/50 at T41, 0/50 at T70 and 0/50 at T84. The P sector occupied by a dog with a burden of approximately 1000 adult E. granulosus yielded 100% (6/6) and 83.3% (5/6) of the soil quadrants positive for eggs of the parasite at T0 and T41, respectively. The P sector occupied by a dog that had a parasite burden of 100 adults yielded 83.3% (5/6) and 50% (3/6) of the quadrants positive for eggs at T0 and T41, respectively. The other positive sample at T41 corresponded to quadrant number 38, located at the adjacent sector (A) in the direction of prevailing winds (see fig. 1). The coproantigen test was positive in 9/12 of topsoil samples at T0, and 2/12 at T41; in both cases the samples were from P quadrants occupied by infected dogs. As an additional qualitative observation, it is worth noting that dogs used to defaecate at one end of their plot, and therefore most of their faeces were accumulated in that sector. Coincidentally, the soil quadrants that were positive for parasite eggs and coproantigen test were those close to this sector.
Table 2. Presence of taeniid-E. granulosus-like eggs in topsoil samples collected at different times from field quadrants in Sarmiento (Chubut province, Patagonia, Argentina).

PU1 and PU2: plots occupied by uninfected dog
PI1: plot occupied by infected dog with a parasitic burden of approximately 1000 adults of E. granulosus
PI2: plots occupied by infected dog with a parasitic burden of approximately 100 adults of E. granulosus
A: adjacent zones to dog plots (P), with a width of 10 m
M: middle-distance sectors located 10–100 m from P plots
D: distant areas located > 100 m from dog plots
Sludge sediment samples were negative at T0 and positive for taeniid-E. granulosus-like eggs at T41. None of the water samples were positive for eggs at any time. Other elements, such as diatoms and cyanobacteria, were detected in these water samples.
Discussion
This study provides insights into the dispersion of E. granulosus eggs under natural field conditions in Patagonia, showing that parasite eggs were found within a maximum distance of 115 m from the deposition site over the course of 41 months. However, 95% of the soil samples positive for parasite eggs came from the sectors occupied by dogs or where they usually relieved themselves.
Up to now the models used to determine the dispersion of Taeniidae eggs in the environment have mainly used Taenia hydatigena, T. saginata, T. pisiformis and E. multilocularis (Atkinson et al., Reference Atkinson2013). Taeniid eggs can disperse widely across the environment. Previous studies in New Zealand showed that T. hydatigena eggs were dispersed up to 80 m over 10 days (Roberts et al., Reference Roberts, Lawson and Gemmell1987), and although the majority of the eggs remained within a radius of 180 m from the deposition site, they could still be dispersed up to a distance of 10 km or over 30,000 ha via biological agents, such as insects (Gemmell, Reference Gemmell, Andersen, Ouhelli and Kachami1997). It has also been shown that T. hydatigena eggs could disperse over 60 km as a consequence of the transfer of viable taeniid eggs by birds (Torgerson et al., Reference Torgerson1995). In fact, viable eggs of T. saginata and T. pisiformis were recovered from bird faeces, as pointed out by Torgerson (Reference Torgerson2016). Recently, Lass et al. (Reference Lass2015) demonstrated for the first time the contamination of fresh fruit, vegetables and mushrooms with E. multilocularis eggs. As sampling sites were located away from homesteads, at distances ranging from a few metres to several kilometres, it seems logical to think that some kind of parasite egg dispersion mechanism from dog faeces to the environment was taking place. In our model a sample from pond sediment as well as some topsoil samples from quadrants located in the direction of the prevailing winds (NE direction) were positive for taeniid-E. granulosus-like eggs after 41 months. This observation is in agreement with the proposal of Bourée (Reference Bourée2001) that wind and water influence the dispersion of E. granulosus eggs into the environment.
For E. granulosus, environmental factors that promote the creation of improved conditions for egg survival, such as cool temperatures, humidity and shade, can result in their presence in any given area (Wachira et al., Reference Wachira, Macpherson and Gathuma1991; Van Kesteren et al., Reference Van Kesteren2013; Cadavid Restrepo et al., Reference Cadavid Restrepo2018). Thus, we argue that the sparse vegetation of low bushes and the mean cool environmental temperatures in the study area can act as protection against excessive exposure to sunlight and desiccation, which increases the probability of worm egg survival. Similar results and conclusions were reached for the viability characterization of the E. granulosus egg samples generated in the present survey, as described elsewhere (Sánchez Thevenet et al., Reference Sánchez Thevenet2003, Reference Sánchez Thevenet2005).
With regards to dog parasite burden and the presence of eggs in soil, in plots occupied by infected dogs, eggs were found in most of the topsoil samples studied after 41 months. Moreover, the greater number of positive soil samples came from plots occupied by the dog with the highest worm burden. Recently, we proved that T. hydatigena eggs are clumped when they are outside the proglottids originally containing them, suggesting that clumping could be a survival strategy for these eggs in the face of adverse environmental conditions (Sánchez Thevenet et al., Reference Sánchez Thevenet, Alvarez and Basualdo2017). In addition to this, we demonstrated that triacylglycerols (TAG) play a key role in the maintenance of embryonic viability in T. hydatigena eggs, a survival strategy in the environment outside the host (Sánchez Thevenet et al., Reference Sánchez Thevenet, Basualdo and Alvarez2010). Generalizing these observations to E. granulosus, it may be possible that the greater the dog parasite burden the greater the possibility of generating clumps of eggs that then assure their persistence and dispersion in the environment. Thus, our findings support the notion that dogs’ infection burden is indeed a key determinant of the permanence and dispersion of E. granulosus eggs in the environment, and also that the TAG depots could liberate eggs from dependence on external energy sources, thus providing an endogenous reserve that could be utilized when external nutrients are unavailable.
The persistence of the E. granulosus life cycle is linked to the long-lasting viability of parasite eggs in the environment. In a previous study, we demonstrated that once released in the environment, parasite eggs may remain viable and at the semi-senescent stage for at least 41 months under Patagonian climate conditions, thus keeping their capacity to generate infection in the intermediary ovine host (Sánchez Thevenet et al., Reference Sánchez Thevenet2005). In this subsequent study, we did not find parasite eggs in either dog faeces or topsoil samples after 70 months of permanence of the contaminated dog scats. However, we corroborated that the coproantigens of E. granulosus remain positive after 70 months in faeces. These findings have enormous practical implications, as this new laboratory-based tool is currently being implemented for surveillance in disease control programmes (Allan and Craig, Reference Allan and Craig2006; Pierangeli et al., Reference Pierangeli2010).
Long-distance dispersion of eggs is important for CE transmission dynamics at larger spatial scales, but our understanding of this phenomenon is mostly based on mathematical modelling rather than field data. It is noteworthy that when field data are included in the aforementioned models, most of them constitute data on the prevalence of the disease in host species, rather than information on the decay of the quantity and viability of parasite eggs in the environment, and their distribution as a consequence of the burden of infection or the behaviour of the dog. This paper attempts to enhance the information on this question, providing evidence from a real environmental scenario. The present prototype is open to comments, suggestions and contributions. It is important to take into account that the main limitations of the study include the low sensitivity of the technique used to screen eggs in water samples and the limited accuracy of the Western Blot method applied in soil samples to confirm the identity of Echinococcus eggs. We thus consider it necessary to optimize technical options applied in future investigation. As has been pointed out by Umhang et al. (Reference Umhang2017), there is a need to improve the standardization of technical options to study samples such as soil, water or sediments. Very few data are currently available on Echinococcus species in soil and other environmental matrices (Shaikenov et al., Reference Shaikenov2004; Szostakowska et al., Reference Szostakowska2014; Lass et al., Reference Lass2015; Umhang et al., Reference Umhang2017). One technical option proposed to confirm them as E. granulosus eggs is polymerase chain reaction (PCR). As Alvarez Rojas et al. (Reference Alvarez Rojas, Mathis and Deplazes2018) point out in their latest review on assessing the contamination of food and the environment with taeniid eggs (including Echinococcus eggs), the isolation and molecular identification of the eggs is technically challenging and little standardized. Unfortunately, we have not had the opportunity to perform PCR studies on our soil samples. That is why we use the expression taeniid-E. granulosus-like eggs. Furthermore, we have not studied the possibility of egg transport either by birds or by arthropods, an unexplored area in the echinococcosis research world.
Finally, it is worth highlighting the suggestion in Chaâbane-Banaoues et al. (Reference Chaâbane-Banaoues2015, Reference Chaâbane-Banaoues2016) and Souto et al. (Reference Souto, Sánchez Thevenet and Basualdo Farjat2016) in relation to the fact that environmental parameters determine the potential occurrence of parasite eggs, while other parameters relating to human behaviour will interact to influence prevalence of disease in the population. Our results validate this hypothesis and show that the dispersion of E. granulosus eggs in the Patagonian region can be explained by factors such as landscape attributes, meteorological conditions and final host infection burden and behaviour. The development of spatio-temporal models including real data on dispersion drivers to determine risk of CE is essential to design more cost-effective control strategies across diverse settings. Since the studies by Gemmell et al. in the 1970s and 1980s there have been almost no reports on the dispersion of Echinococcus granulosus eggs from infected dogs, perhaps due to the difficulty in undertaking biohazardous and long-term investigations with infected dogs. Therefore, we think that the value of the present study is to contribute significantly to the knowledge we have about natural dissemination of E. granulosus eggs from dogs and the contamination profile of local environments.
Author ORCIDs
P. Sánchez Thevenet, 0000-0001-7018-6128
Acknowledgements
We would like to thank the Sanitary Technician Carlos Terán, from the Zoonoses Department of Chubut Province, Argentina, for his support in sample collection.
Financial support
This study was partially supported by Universidad Nacional de la Patagonia San Juan Bosco (ResCS126/06).
Conflict of interest
None.
Ethical standards
See Materials and methods section.