Long-chain n-3 PUFA, such as EPA (20 : 5n-3) and DHA (22 : 6n-3), have a cardioprotective effect( Reference Alexander, Miller and Van Elswyk 1 ). n-3 PUFA can be obtained from fish and from the conversion of α-linolenic acid (ALA, 18 : 3n-3), which is found in flaxseed and perilla oil( Reference Barcelo-Coblijn and Murphy 2 ). Isotope tracer studies have suggested that the conversion of ALA to DHA is higher in rats (approximately 70 %)( Reference Lin and Salem 3 ) than in humans (0·01–9 %)( Reference Baker, Miles and Burdge 4 ) due to the constraint at fatty acid desaturase (FADS) 1, elongase of very long fatty acid (ELOVL) 2 or translocation of twenty-four carbon intermediates( Reference Burdge 5 ). In addition, the conversion rate of ALA to DHA is lower in men (0·01–4 %) than in women (approximately 9 %)( Reference Baker, Miles and Burdge 4 ) due to the oestrogen-mediated upregulation of the conversion( Reference Burdge 6 ).
Oestrogen( Reference Kitson, Stroud and Stark 7 – Reference McNamara, Able and Jandacek 9 ), dietary content of ALA( Reference Brenna, Salem and Sinclair 10 , Reference Valenzuela, Barrera and Gonzalez-Astorga 11 ), fatty acid composition of the diet( Reference Blanchard, Pedrono and Boulier-Monthean 12 ) and dietary content of linoleic acid (LA, 18 : 2n-6)( Reference Valenzuela, Barrera and Espinosa 13 , Reference Wang, Botolin and Xu 14 ) have been shown to affect the conversion of ALA into DHA. Previous studies have reported that oestrogen increases the conversion of ALA into DHA in rat( Reference Kitson, Marks and Shaw 8 , Reference McNamara, Able and Jandacek 9 ), given that oestrogen increases the hepatic expression of FADS2 through modulation of PPAR-α expression( Reference Kitson, Stroud and Stark 7 ). Increased intake of ALA also enhances the conversion of ALA into EPA and DHA in rats and humans( Reference Brenna, Salem and Sinclair 10 , Reference Valenzuela, Barrera and Gonzalez-Astorga 11 ). It has been shown that ALA increases hepatic expressions of FADS2, FADS1, ELOVL5, ELOVL2 and peroxisomal acyl-coenzyme A oxidase 1 (ACOX1) through regulating PPAR-α ( Reference Gonzalez-Manan, Tapia and Gormaz 15 , Reference Jump 16 ). In addition, an increased LA:ALA ratio decreases the conversion of ALA into EPA and DHA in rat( Reference Blanchard, Pedrono and Boulier-Monthean 12 ), because LA competes with ALA for conversion processes including desaturation, elongation and peroxisomal β-oxidation( Reference Schmitz and Ecker 17 ). The conversion enzymes are known to be regulated by transcription factors such as PPAR-α and sterol regulatory element binding protein 1 (SREBP1)( Reference Jump 16 ).
Previous studies reported that high-LA diets( Reference Raatz, Bibus and Thomas 18 – Reference Goyens, Spilker and Zock 20 ) and a high-SFA diet( Reference Gonzalez-Manan, D’Espessailles and Dossi 21 ) decreased plasma phospholipid levels of EPA and DHA as compared with a low-LA diet and a low-SFA diet, respectively. A high-LA diet and a high-SFA diet decreased the expressions of the FADS( Reference Rodriguez-Cruz, Tovar and Palacios-Gonzalez 22 , Reference Picklo and Murphy 23 ) and ELOVL( Reference Wang, Botolin and Xu 14 , Reference Song, Qu and Zhang 24 , Reference Tripathy, Torres-Gonzalez and Jump 25 ) in female or male rodents. A high-SFA diet consistently decreased the expression of PPAR-α ( Reference Gonzalez-Manan, D’Espessailles and Dossi 21 ) but increased SREBP1( Reference Dossi, Tapia and Espinosa 26 ) in male rodents. However, the effect of a high-LA diet on the transcription factors was controversial. In male rats, diets containing 9·7–16·6 % LA from total energy intake (with 0·2–6·0 % ALA) decreased hepatic expressions of PPAR-α and increased SREBP1 when compared with diets containing 4·6–9·7 % LA (with 6·0–12·6 % ALA)( Reference Gonzalez-Manan, D’Espessailles and Dossi 21 , Reference Rincon-Cervera, Valenzuela and Hernandez-Rodas 27 ). On the other hand, Tu et al. ( Reference Tu, Cook-Johnson and James 28 ) reported no significant differences between the diets containing 6 % LA from total energy intake (with 0·6 % ALA) and 0·4 % LA (with 0·03 % ALA). In the previous studies, dietary intake of LA and ALA were changed, suggesting that the effect on the expression of conversion enzymes and transcription factors was due to either LA or ALA. Thus, the purpose of this study was to investigate the hypothesis that a high-LA diet decreases the conversion of ALA to EPA and DHA by reducing the expression of the conversion enzymes by modulating the expression of transcription factors, while the ALA content is the same in low- and high-LA diets. In addition, we compared the synergic effect of oestrogen and a low-LA diet on the increased conversion of ALA into EPA and DHA in ovariectomised rats.
Methods
Animals and diets
The experimental protocol was approved by the Institutional Animal Care and Use Committee of Hanyang University (HY-IACUC-16-0027). In all, 3-week-old female Wistar rats (Jung-Ang Lab. Animal Inc., Seoul, Korea) were housed in individually ventilated cages in an air-conditioned room maintained at 22 ± 1°C and 40–50 % humidity, with a 12 h light–12 h dark cycle. During a week of acclimation, rats (n 42) were provided a standard chow diet and fresh tap water ad libitum. After acclimation, rats were provided experimental diets and weighed weekly, and food intake was measured daily. The experimental diets were modified American Institute of Nutrition-93G diets containing 15·8 % fat with 10 % LA and 0 % ALA (control diet) or with 9·1 % LA and 1 % ALA (low-LA diet) or a high-fat diet containing 40 % fat with 24·5 % LA and 1 % ALA (high-LA diet expressed as percentage total energy) (Table 1). Grape seed oil (Sajo Haepyo) was used instead of soyabean oil to limit the level of ALA and provide LA, which is the main competitor of ALA, in the process of conversion to long chain PUFA, and flaxseed oil (Amaflax) was used as the source of ALA.
Table 1 Composition of experimental diets
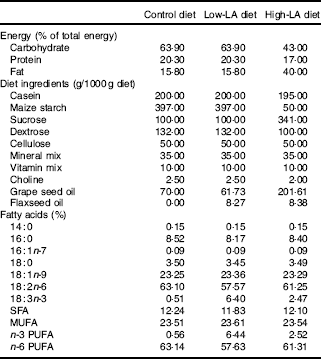
Control diet, 0 % n-3 PUFA diet; LA, linoleic acid; ALA, α-linolenic acid; low-LA diet, 1 % ALA (18 : 3n-3) in 9·1 % LA (percentage of total energy) diet; high-LA diet, 1 % ALA in 24·5 % LA diet.
Experimental design
During the 12 weeks of the experiment, rats were assigned to one of the three diets: low-LA diet containing 0 % n-3 PUFA from energy (n 18), low-LA diet containing 1 % ALA from energy (n 12) and high-LA diet containing 1 % ALA from energy (n 12). At week 8, rats were ovariectomised or underwent a sham surgery under anaesthesia using a combination of zoletil (25 mg/kg) and rompun (10 mg/kg). From 1 week after surgery, rats were subcutaneously injected with 1 ml of maize oil vehicle (Sigma-Aldrich) with or without 10 µg of 17β-oestradiol-3-benzoate (E) to mimic the rat’s oestrus cycle, every 4 d during the last 3 weeks of the experiment. Thus, rats were divided into seven groups (n 6/group): 0 % n-3 PUFA diet, without E after sham surgery; 0 % n-3 PUFA diet, without E and with E after ovariectomy; ALA in low-LA diet (9·1 % LA of total energy), without E (low-LA) and with E after ovariectomy (low-LA-E); and ALA in high-LA diet (24·5 % LA of total energy), without E (high-LA) and with E after ovariectomy (high-LA-E).
Tissue and blood collection
After 12 weeks of the experiment, rats were fasted overnight and euthanised using zoletil (25 mg/kg) and rompun (10 mg/kg). Blood and organs were obtained and were stored at –80°C for further analysis.
GC
Liver tissue (100 mg) was homogenised using 5 ml of chloroform–methanol–distilled water (2:2:1, by vol.)( Reference Zhang and Hong 29 ). The homogenates were centrifuged at 4000 rpm (Hanil Science Inc.) for 10 min, and the solvents of bottom layer were redissolved in n-hexane and separated by thin-layer chromatography (SIL G-25; Macherey-Nagel GmbH & Co.) in n-hexane–diethyl ether–acetic acid (40:10:1, by vol.). After developing the TLC plate, phospholipids were scraped off. Liver phospholipids and serum without lipid extraction were directly methylated by adding boron trifluoride methanol (B1252; Sigma-Aldrich) and heated at 100°C for 10 and 45 min, respectively. Fatty acid methyl esters of liver phospholipid and serum were analysed using GC (Shimadzu 2010AF; Shimadzu Scientific Instrument) with a 100 m × 0·25 mm inner diameter with 0·20 µm film capillary column (SP2560; Supelco). H2 was used as the carrier gas at a flow rate of 40 ml/min. The injection and detection temperatures were 230 and 240°C, respectively. The run temperature began at 180°C and then was increased at 5°C/min increase up to 200°C and then increased by 10°C/min to 240°C. The split ratio was 10:1. GC was calibrated with an external standard composed of defined fatty acids (GLC-727; Nu-Check Prep). Qualified GC evaluation was carried out by measuring CV of sum of EPA and DHA composition in quality control material( Reference Domenichiello, Kitson and Chen 30 ), and the CV was 2·4 %.
Western blot analysis
Hepatic tissues were homogenised in ice-cold lysis buffer (20 mm HEPES, 0·25 m sucrose, 0·5 mm EDTA, 2 mm dithiothreitol, 1 mm phenylmethylsulfonyl fluoride, 10 µg/ml leupeptin, 10 µg/ml aprotinin, and 1 mm sodium orthovanadate; pH 7·5) including protease inhibitor cocktail tablets and phosphatase inhibitor cocktails (EDTA-free protease inhibitor and PhosSTOP; Roche Diagnostics GmbH) using a Tissuemiser (Fisher Scientific). The homogenates were centrifuged at 20 000 g (Eppendorf) for 60 min at 4°C. The protein concentration of supernatants was determined by the Bradford method using bovine serum albumin (Bio-Rad) as the standard. Protein (30 µg) was separated on 8 or 10 % SDS-PAGE, transferred to a polyvinylidene fluoride membrane (0·45 µm; Merck Millipore) and blocked for 1 h at room temperature with 5 % skimmed milk in TRIS-buffered saline containing Tween-20 (TBST). The membranes were incubated overnight with a primary antibody oestrogen receptor (ER)-α (1:1000), ER-β (1:1000), PPAR-α (1:1000), SREBP1 (1:500), FADS2 (1:400), FADS1 (1:500), ELOVL5 (1:200), ELOVL2 (1:10 000) or ACOX1 (1:2000) with 5 % skimmed milk or 5 % bovine serum albumin in TBST at 4°C. The antibodies of ER-α, ER-β, PPAR-α, SREBP1, FADS2, FADS1, ELOVL2 and ACOX1 were purchased from Abcam; ELOVL5, Santa Cruz Biotechnology. After washing in TBST, membranes were incubated with horseradish peroxidise (HRP)-conjugated goat anti-rabbit (Cell Signaling Technology), anti-mouse IgG (Enzo Life Science) or donkey anti-goat IgG-HRP (Santa Cruz Biotechnology) with 5 % skimmed milk in TBST for 1 h at room temperature. Immunoreactive bands were visualised under the UV setting of the ChemiDoc MP Imaging System (Bio-Rad) to estimate the total protein per lanes, and β-actin (1:1000; BD Transduction Laboratories) was used for normalisation. Each Western blot was repeated three times.
Statistical analysis
The sample size was calculated using PASS version 14 (NCSS LLC) based on the previously described effect of high-LA diet consumption on the hepatic level of DHA( Reference Clarke, Jump and Berdanier 31 ). Concerning the hepatic level of DHA, a sample size of six animals per group would provide the appropriate power (1–β–0·95) to identify significant differences (α=0·05) in the variables analysed with an effect size d=2·59, two-sided test and a sample size ratio=1. Values are expressed as the mean with their standard errors, and differences were considered significant at P <0·05. Data were analysed using a two-way ANOVA with factors of ALA and E or LA and E, followed by Duncan’s post hoc test. Analysis was performed using SPSS for Windows, version 21.0 (SPSS Inc.).
Results
Dietary intake, body weight and organ weights
Regardless of the diet, rats with E by injection or sham surgery consumed significantly less food and had lower final body weights but heavier liver, uterus and kidney weights than rats without E (Table 2). Regardless of E, rats fed the high-LA diet consumed less food than those fed the low-LA diet; however, their body and organ weights were not significantly different.
Table 2 Dietary intake, organ weights and body weight (Mean values with their standard errors, n 6 per group)

E, 17β-oestradiol-3-benzoate; Sham, 0 % n-3 PUFA diet without E after sham surgery; OVX and OVX-E, 0 % n-3 PUFA diet without E and with E after ovariectomy; LA, linoleic acid; ALA, α-linolenic acid; low-LA and low-LA-E, 1 % ALA (18 : 3n-3) in 9·1 % LA diet without E and with E after ovariectomy; high-LA and high-LA-E, 1 % ALA in 24·5 % LA diet without E and with E after ovariectomy.
* Mean values were significantly different between maize oil and E by injection or sham surgery within the same diet (P < 0·05).
† Mean values were significantly different among low-LA and high-LA with maize oil or E injection, respectively (P < 0·05).
Fatty acid composition of serum and liver phospholipid
Regardless of the diet, rats with E by injection or sham surgery had lower serum and liver phospholipid levels of 14 : 0, 16 : 0, 16 : 1n-7, 18 : 1n-9 and MUFA, but higher 18 : 0, 22 : 5n-6, 22 : 6n-3 and n-3 PUFA than rats without E (Tables 3 and 4). However, E had no effect on the serum and liver levels of 20 : 5n-3. Regardless of E, rats fed the high-LA diet had lower serum and liver phospholipid levels of 14 : 0, 16 : 0, 16 : 1n-7, 18 : 1n-9, 18 : 3n-3, 20 : 5n-3, 22 : 5n-3, 22 : 6n-3, SFA, MUFA and n-3 PUFA but higher 18 : 2n-6, 20 : 4n-6, 22 : 4n-6, 22 : 5n-6 and n-6 PUFA than rats fed the low-LA diet. Regardless of E and amount of dietary LA, rats fed ALA had lower serum and liver levels of 22 : 4n-6, 22 : 5n-6 and n-6 PUFA but higher 18 : 3n-3, 20 : 5n-3, 22 : 5n-3, 22 : 6n-3 and n-3 PUFA than rats fed the 0 % n-3 PUFA diet. There was a synergic effect of low-LA and E on the increased serum and liver phospholipid levels of 22 : 6n-3 (P=0·001 and P <0·001).
Table 3 Fatty acid (FA) composition of serum (Mean values with their standard errors, n 6 per group)

E, 17β-oestradiol-3-benzoate; Sham, 0 % n-3 PUFA diet without E after sham surgery; OVX and OVX-E, 0 % n-3 PUFA diet without E and with E after ovariectomy; LA, linoleic acid; ALA, α-linolenic acid; low-LA and low-LA-E, 1 % ALA (18 : 3n-3) in 9·1 % LA diet without E and with E after ovariectomy; high-LA and high-LA-E, 1 % ALA in 24·5 % LA diet without E and with E after ovariectomy.
* Mean values were significantly different between maize oil and E by injection or sham surgery within the same diet (P < 0·05).
† Mean values were significantly different among low-LA and high-LA with maize oil or E injection, respectively (P < 0·05).
‡ Mean values were significantly different among OVX and low-LA with maize oil or E injection, respectively (P < 0·05).
Table 4 Fatty acid (FA) composition of liver phospholipids (Mean values with their standard errors, n 6 per group)

E, 17β-oestradiol-3-benzoate; Sham, 0 % n-3 PUFA diet without E after sham surgery; OVX and OVX-E, 0 % n-3 PUFA diet without E and with E after ovariectomy; LA, linoleic acid; ALA, α-linolenic acid; low-LA and low-LA-E, 1 % α-linolenic acid (18 : 3n-3) in 9·1 % LA diet without E and with E after ovariectomy; high-LA and high-LA-E, 1 % ALA in 24·5 % LA diet without E and with E after ovariectomy.
* Mean values were significantly different between maize oil and E by injection or sham surgery within the same diet (P < 0·05).
† Mean values were significantly different among low-LA and high-LA with maize oil or E injection, respectively (P < 0·05.
‡ Mean values were significantly different among OVX and low-LA with maize oil or E injection, respectively (P < 0·05).
Hepatic expressions of enzymes involved in conversion of α-linolenic acid into EPA and DHA
Regardless of the diet, rats with E by injection or sham surgery had higher hepatic expression of FADS2, ELOVL5, ELOVL2, PPAR-α, ER-α and ER-β but lower SREBP1 than rats without E (Fig. 1–3). Regardless of E, rats fed the high-LA diet had higher hepatic expression of SREBP1 but lower FADS2, FADS1, ELOVL5, ELOVL2 and PPAR-α than rats fed the low-LA diet. Regardless of E and amount of dietary LA, rats fed ALA had higher hepatic expression of FADS2, FADS1, ELOVL5, ELOVL2 and PPAR-α but lower SREBP1 than rats fed the 0 % n-3 PUFA diet. Hepatic expression of ER-α and ER-β was not affected by the amount of dietary LA or ALA intake. Hepatic expression of ACOX1 was not affected by E, LA or ALA. There was a synergic effect of low-LA and E on the increased hepatic expression of PPAR-α (P <0·05).
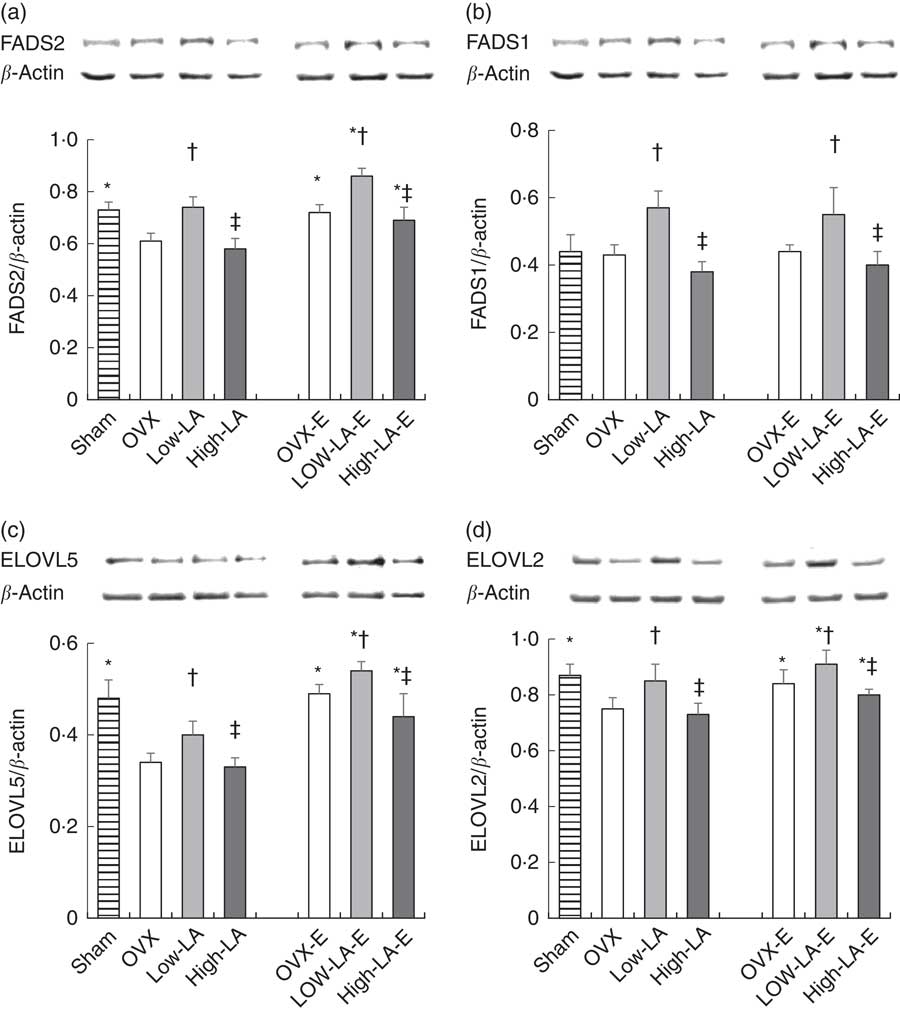
Fig. 1 Effects of 17β-oestradiol-3-benzoate (E), α-linolenic acid (ALA) and linoleic acid (LA) on the hepatic expressions of (a) fatty acid desaturase (FADS)2, (b) FADS1, (c) elongase of very long fatty acid (ELOVL)5 and (d) ELOVL2. Values are means (n 6), with their standard errors represented by vertical bars. * Mean values were significantly different between maize oil and E injection or sham surgery within the same diet (P <0·05). † Mean values were significantly different among OVX and low-LA with maize oil or E injection, respectively (P <0·05). ‡ Mean values were significantly different among low-LA and high-LA with maize oil or E injection, respectively (P <0·05). OVX and OVX-E, 0 % n-3 PUFA diet without E and with E after ovariectomy; low-LA and low-LA-E, 1 % α-linolenic acid (18 : 3n-3) in 9·1 % LA diet without E and with E after ovariectomy; high-LA and high-LA-E, 1 % ALA in 24·5 % LA diet without E and with E after ovariectomy.
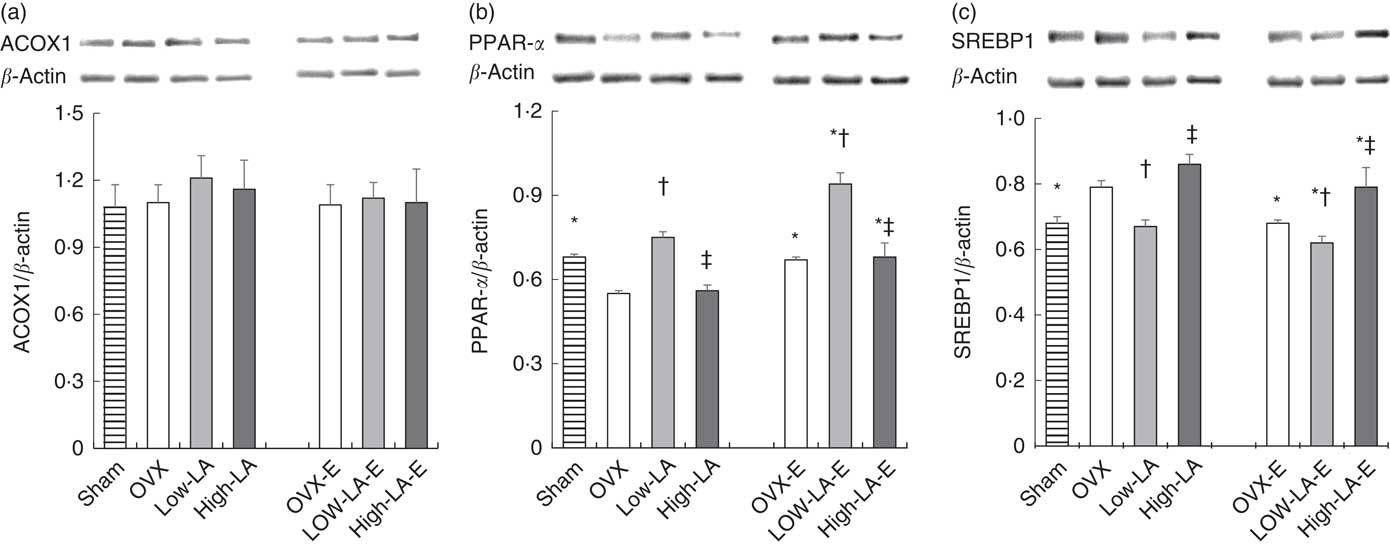
Fig. 2 Effects of 17β-oestradiol-3-benzoate (E), α-linolenic acid (ALA) and linoleic acid (LA) on the hepatic expressions of (a) acyl-coenzyme A oxidase 1 (ACOX1), (b) PPAR-α and (c) sterol regulatory element binding protein 1 (SREBP1). Values are means (n 6), with their standard errors represented by vertical bars. * Mean values were significantly different between maize oil and E injection or sham surgery within the same diet (P <0·05). † Mean values were significantly different among OVX and low-LA with maize oil or E injection, respectively (P <0·05). ‡ Mean values were significantly different among low-LA and high-LA with maize oil or E injection, respectively (P <0·05). OVX and OVX-E, 0 % n-3 PUFA diet without E and with E after ovariectomy; low-LA and low-LA-E, 1 % α-linolenic acid (18 : 3n-3) in 9·1 % LA diet without E and with E after ovariectomy; high-LA and high-LA-E, 1 % ALA in 24·5 % LA diet without E and with E after ovariectomy.

Fig. 3 Effects of 17β-oestradiol-3-benzoate (E), α-linolenic acid (ALA) and linoleic acid (LA) on the hepatic expressions of (a) oestrogen (ER)-α and (b) ER-β. Values are means (n 6), with their standard errors represented by vertical bars. * Mean values were significantly different between maize oil and E injection or sham surgery within the same diet (P <0·05). OVX and OVX-E, 0 % n-3 PUFA diet without E and with E after ovariectomy; low-LA and low-LA-E, 1 % α-linolenic acid (18 : 3n-3) in 9·1 % LA diet without E and with E after ovariectomy; high-LA and high-LA-E, 1 % ALA in 24·5 % LA diet without E and with E after ovariectomy.
Discussion
This study demonstrated that a high-LA diet reduced protein expression of FADS2, FADS1, ELOVL5 and ELOVL2 through increased expression of PPAR-α and decreased expression of SREBP1 in ovariectomised rats, suggesting that a high-LA diet decreased the conversion of ALA into EPA and DHA.
Previous studies consistently reported that high-LA diets decrease the expressions of FADS2 and FADS1( Reference Rodriguez-Cruz, Tovar and Palacios-Gonzalez 22 ) in female rats and ELOVL5( Reference Wang, Botolin and Xu 14 , Reference Tripathy, Torres-Gonzalez and Jump 25 ) in male rats. No previous study has reported the effect of a high-LA diet on ELOVL2. Valenzuela et al. ( Reference Valenzuela, Barrera and Espinosa 13 ) reported that a high-LA diet decreased the activity of FADS2 and FADS1 calculated by the ratios of 18 : 3n-6:LA and 20 : 4n-6:20 : 3n-6, respectively. Although the activity or expression of desaturase was not measured directly, the previous study reported that a high-LA diet decreased the conversion of ALA into EPA and DHA by reduced activity of FADS( Reference Valenzuela, Barrera and Espinosa 13 ). In addition, a high-SFA diet decreased the hepatic expressions of ELOVL5( Reference Wang, Botolin and Xu 14 ), FADS2, FADS1( Reference Rodriguez-Cruz, Tovar and Palacios-Gonzalez 22 ) and ELOVL2, suggesting that high fat with either LA or SFA could decrease all elongases and desaturases.
Two previous studies suggested that diets containing 9·7–16·6 % LA from total energy intake (with 0·2–6·0 % ALA) decreased hepatic expression of PPAR-α but increased SREBP1 as compared with diets containing 4·6–9·7 % LA (with 6·0–12·6 % ALA)( Reference Gonzalez-Manan, Tapia and Gormaz 15 , Reference Rincon-Cervera, Valenzuela and Hernandez-Rodas 27 ), consistent with the present study. In addition, Tu et al. ( Reference Tu, Cook-Johnson and James 28 ) reported no significant differences between the diets containing 6 % LA and 0·4 % LA on the expressions of PPAR-α and SREBP1. This inconsistency could be due to dietary contents of total PUFA, LA and ALA. The high-LA diet containing 6·6 % PUFA had no effect on transcription factors, but the high-LA diet containing approximately 17 % PUFA, which contained a similar amount of PUFA as used presently (25 %), modified the transcription factors. Clarke et al. ( Reference Guillou, Martin and Pineau 32 ) also reported that a diet with 20 % PUFA (from total energy intake) regulated the expression of SREBP1, but a diet with 5 % PUFA did not, suggesting that the amount of PUFA could be important for modification of transcription factors. As mentioned above, PUFA increase the expression of PPAR-α and decreased SREBP1( Reference Devarshi, Jangale and Ghule 33 ), but n-3 PUFA were reportedly more potent modulator of PPAR-α and SREBP1 than n-6 PUFA( Reference Jump 16 , Reference Schmitz and Ecker 17 ). In addition, a diet with LA:ALA=0·5:1 increased the expression of PPAR-α and decreased SREBP1 as compared with a diet with LA:ALA=10:1 in rats( Reference Sheaff, Su and Keswick 34 ). Previous studies modulated dietary content of LA and ALA, since high-LA diets contained low ALA and low-LA diets contained high ALA. However, in the present study, the high- and low-LA diets contained the same amount of ALA.
A few studies showed that hepatic and blood levels of EPA and DHA were lower with a high-LA diet (approximately 26 % LA from the total energy intake and 0·4–1 % ALA from the total energy intake) than a low-LA diet (0·4–3·5 % LA from the total energy intake and 0·03–0·4 % ALA from the total energy intake) in male rats( Reference Valenzuela, Barrera and Gonzalez-Astorga 11 , Reference Tu, Cook-Johnson and James 28 , Reference Wu, Su and Cui 35 ), which is consistent with the present study. Wu et al. ( Reference Cormier, Rudkowska and Lemieux 36 ) reported that enhanced conversion from ALA to DHA traced by isotope occurred through the increased activity of FADS1 in HepG2 cell. In addition, blood levels of EPA and DHA were increased with the increased expression of conversion enzymes, such as FADS and ELOVL, in rats and humans( Reference Valenzuela, Barrera and Espinosa 13 , Reference Childs, Romeu-Nadal and Burdge 37 ). An isotope tracer study conducted in humans reported that a high-LA diet reduced the conversion of ALA to EPA as compared with a low-LA diet. A clinical trial also reported that a high-LA diet significantly decreased the plasma phospholipid level of EPA and DHA, although the high-LA diet contained a high level of ALA compared with a low-LA diet( Reference Tapia, Valenzuela and Espinosa 38 ). Thus, the results of the present study suggested that a high-LA diet reduced the conversion of ALA into EPA and DHA by decreasing the hepatic expression of both desaturases and elongases.
Contrary to our hypothesis, the high-LA diet had no effect on ACOX1 expression in the present study. Previously, there were inconsistent results regarding the effect of high-LA diets on ACOX1, a peroxisomal β-oxidation enzyme. Consistent with the present study, high-LA diets, containing 3·7 times more LA (12·6 % from energy), without n-3 PUFA supplementation, have been shown to decrease the hepatic expression of ACOX1 but high-LA diets with n-3 PUFA supplementation did not( Reference Ide, Kobayashi and Ashakumary 39 ). Previous studies reported that n-3 PUFA increase ACOX1 expression with diets containing LA:ALA ratios of 0·2–0·3, but not LA:ALA ratios of 1·3–387( Reference Gonzalez-Manan, Tapia and Gormaz 15 , Reference Mason, Kharotia and Wiggins 40 ), suggesting that LA:ALA ratios higher than 1·3 had no effect on ACOX1 expression. In the present study, LA:ALA ratios were 9–124, and ACOX1 expression was not changed. Although ALA had no effect on ACOX1 expression in the present study, previous studies showed that n-3 PUFA could allow the recovery of PPAR-α signalling after reduction due to high-LA diets and, consequently, trigger the recovery of ACOX1 expression( Reference Ide, Kobayashi and Ashakumary 39 ).
Consistent with our results, previous studies reported that oestrogen increases hepatic and blood levels of DHA but not EPA( Reference Kitson, Marks and Shaw 8 , Reference Kitson, Smith and Marks 41 ). Oestrogen also increases the hepatic expressions of FADS2( Reference Kitson, Marks and Shaw 8 ), ELOVL2( Reference Zhang, Li and Li 42 ) and ELOVL5( Reference Kim, Jin and Park 43 ), which are modulated by increased PPAR-α ( Reference Kitson, Stroud and Stark 7 ) and decreased SREBP1( Reference Nakamura and Nara 44 ). However, Kitson et al. ( Reference Kitson, Marks and Shaw 8 ) reported that oestrogen has no effect on the hepatic expression of FADS1, converting 20 : 4n-3 to EPA, which is consistent with the present study. Although FADS1 expression was not affected, oestrogen increased the conversion of ALA into DHA, because it increased the expression of FADS2 – the rate-limiting enzyme in the conversion of ALA into DHA( Reference Oh, Jin and Park 45 ).
In the present study, oestrogen and the low-LA diet have synergic effects on serum and liver phospholipid levels of DHA and on the hepatic expression of PPAR-α. Consistent with the present study, it has been well known that oestrogen increases the hepatic expressions of ER-α and ER-β ( Reference Nakamura and Nara 44 ,46). The binding of oestrogen to membrane ER directly phosphorylates PPAR-α and increases the intracellular concentration of DHA, which indirectly activates PPAR-α via elevated Ca phospholipase A2 ( Reference Kitson, Stroud and Stark 7 ). The present study and previous studies( Reference Gonzalez-Manan, D’Espessailles and Dossi 21 , Reference Ide, Kobayashi and Ashakumary 39 ) consistently showed that low-LA diets increase PPAR-α expression. Therefore, the combination of oestrogen and a low-LA diet have an added effect on PPAR-α expression. Regarding the synergic effect on DHA level, the low-LA diet increased the conversion of ALA into EPA and DHA, but oestrogen only increased the conversion of ALA into DHA. Thus, the synergic effect of oestrogen and low-LA diets was observed on the hepatic level of DHA, but not EPA, in the present study.
The present study had a few limitations. First, the high-LA diet had a higher content of sugar compared with the low-LA diet. Thus, these components could influence the low conversion of ALA into DHA in the high-LA diet. Second, the conversion of ALA to DHA was estimated by fatty acid composition rather than using the stable isotope tracer technique. Third, mRNA levels of transcription factors and conversion enzymes were not measured to confirm our findings at the gene level.
Conclusion
The high-LA diet decreased the conversion of ALA into EPA and DHA by reducing the expression of FADS and elongases through modification of transcription factors such as PPAR-α and SREBP1. In addition, oestrogen enhanced the conversion of ALA into DHA by modifying conversion enzymes and transcription factors, particularly PPAR-α, synergically with the low-LA diet.
Acknowledgements
The authors are grateful to Youri Jin for technical assistance.
This research was supported by the Basic Science Research Program through the National Research Foundation of Korea (NRF) funded by the Korean government (grant no. NRF-2015R1D1A1A09060823).
The authors’ contributions are as follows: D. K. carried out the experimental work and prepared the manuscript; J.-E. C. conducted the statistical analyses; Y. P. contributed to the interpretation of the results, editing of the manuscript, and to the final text. All authors read and approved the final manuscript.
None of the authors has any conflicts of interest to declare.