Colorectal cancer is the third most common cancer among males and second among females worldwide, accounting for approximately two million new incidents in 2020 (Xi and Xu, Reference Xi and Xu2021). Known risk factors associated with colorectal cancer include genetic, lifestyle and environmental factors. Investigating the role of lifestyle factors such as physical inactivity, obesity, smoking and alcohol consumption is important due to the potential for improving our understanding of the disease (Aran et al., Reference Aran, Victorino, Thuler and Ferreira2016).
Recent epidemiological and experimental studies suggest that endogenous concentrations of sex hormones, such as estradiol, testosterone and sex hormone-binding globulin (SHBG), play a role in colorectal cancer risk. Lin et al. (Reference Lin, Zhang, Rexrode, Manson, Chan, Wu, Tworoger, Hankinson, Fuchs, Gaziano, Buring and Giovannucci2013) identified an association between estrone, estradiol, testosterone and SHBG and colorectal cancer risk. The authors measured the plasma level of sex hormones in 732 colorectal cancer patients (293 women and 439 men) and 1156 controls (437 women and 719 men). They applied the unconditional logistic regression method to calculate relative risk (RR) and confidence intervals (CIs) of two-sided tests. Both testosterone (RR = 0.62; 95% CI [0.40, 0.96]) and SHBG (RR = 0.65; 95% CI [0.42, 0.99]) showed an inverse association with colorectal cancer among men after adjusting for body mass index (BMI) and plasma levels of c-peptides. Among women, only the ratio between estradiol and testosterone (RR = 0.43; 95% CI [0.22, 0.84]) was inversely associated with colorectal cancer after adjusting for potential confounding.
A recent study by Yang et al. (Reference Yang, Giovannucci, Hankinson, Chan, Ma, Wu, Fuchs, Lee, Sesso, Lin and Zhang2020) focused on investigating the association between circulating sex hormones and the survival of colorectal cancer patients. Samples were collected from 609 cases (370 men and 239 postmenopausal women not taking hormone therapy at blood collection). Multivariate hazard ratios (HRs) and 95% CIs were estimated using the Cox proportional hazard regression. The authors showed that higher circulating levels of free testosterone were associated with a lower risk of overall and colorectal cancer-specific mortality among men. A positive correlation was found between estrone and both overall- and colorectal cancer-specific mortality among women. Leveraging data from the UK Biobank, Dimou et al. (Reference Dimou, Mori, Harlid, Harbs, Martin, Smith-Byrne, Papadimitriou, Bishop, Casey, Colorado-Yohar, Cotterchio, Cross, Marchand, Lin, Offit, Onland-Moret, Peters, Potter, Rohan and Murphy2021) conducted both observational and two-sample Mendelian randomization (MR) analyses to elucidate the role of circulating levels of testosterone and SHBG in colorectal cancer risk. The authors found no causal associations between circulating SHBG and free testosterone with colorectal cancer risk.
In addition to studying the association between sex hormones and colorectal cancer risk, recent studies have focused on the effect of sex steroids and colorectal cancer precursors, including conventional adenomas and serrated polyps. Hang et al. (Reference Hang, He, Kværner, Chan, Wu, Ogino, Hu, Shen, Giovannucci and Song2021) investigated the association between plasma sex hormones and early initiation of colorectal cancer among 5404 postmenopausal women from the Nurses’ Health Study I and II. Using the multivariate logistic regression approach, they observed that higher concentrations of SHBG were associated with a lower risk of conventional adenomas. A nominally significant association between SHBG and a lower risk of large-serrated polyps (≥10 mm) was found. In addition to SHBG, free estradiol and free testosterone were associated with a higher risk of conventional adenomas. A recent study investigated the serum hormone levels (testosterone, androstenedione, DHEAS [dehydroepiandrosterone sulfate] and SHBG) and the risk of colorectal precursors among men (Figueiredo et al., Reference Figueiredo, Gresham, Barry, Mott, Passarelli, Bradshaw, Anderson and Baron2022). Authors have found that levels of free testosterone, total testosterone, androstenedione, DHEAS or SHBG are not associated with either ‘low-risk’ (single tubular adenoma <1 cm) or ‘high-risk’ (advanced adenoma or sessile serrated adenoma or right-sided serrated polyps or >2 adenomas in any size) early precursor lesions in the colorectum.
Previous studies have investigated the causal effects of hormone-related traits such as age at menarche, age at menopause, using oral contraceptives and hormone replacement therapy (HRT) on colorectal cancer risk. Li et al. (Reference Li, Song, Wang, Meng, Guo, Liu, Lv and Wu2013) conducted a meta-analysis (11 case–control and 11 cohort studies) to assess the relationship between age at menarche and colorectal cancer. The random-effects pooled RR for the oldest versus the youngest age of menarche was 0.95 (95% CI [0.85, 1.06]) with significant heterogeneity (p < .001). Approximately similar RR values were obtained for separate case–control and cohort studies. Therefore, these RR results explained that age at menarche was not associated with colorectal cancer risk. Neumeyer et al. (Reference Neumeyer, Banbury, Arndt, Berndt, Bezieau, Bien, Buchanan, Butterbach, Caan, Campbell, Casey, Chan, Chanock, Dai, Gallinger, Giovannucci, Giles, Grady, Hampe and Chang-Claude2018) used an MR approach to test the causal effect of age at menarche and menopause on colorectal cancer risk. The authors found that age at menarche (OR = 0.98; 95% CI [0.95, 1.02]) and age at menopause (OR = 0.98; 95% CI [0.94, 1.01]) were not associated with colorectal cancer risk. Symer et al. (Reference Symer, Wong, Abelson, Milsom and Yeo2018) conducted a meta-analysis among women aged 55 to 74 years to evaluate the colorectal cancer incidence (disease-specific mortality and all-cause mortality) on an individual’s use of HRT at the time of randomization: never, current or former users. The authors found that adjusted colorectal cancer incidence in current users compared to never-users was lower (HR = 0.81; 95% CI [0.69. 0.94]; p = .005), as was death from colorectal cancer (HR = 0.63; 95% CI = [0.47, 0.85]; p = .002) and all-cause mortality (HR = 0.76; 95% CI [0.72, 0.80]; p < .001).
Despite the accumulating evidence for the role of sex hormones in colorectal cancer, the effect of ‘sex hormone precursors’ has not been studied thoroughly. DHEAS is the precursor of major sex hormones in our body, testosterone in males and estrogen in females (see Figure 1). The concept for Figure 1 was adopted from Allolio and Arlt (Reference Allolio and Arlt2002).
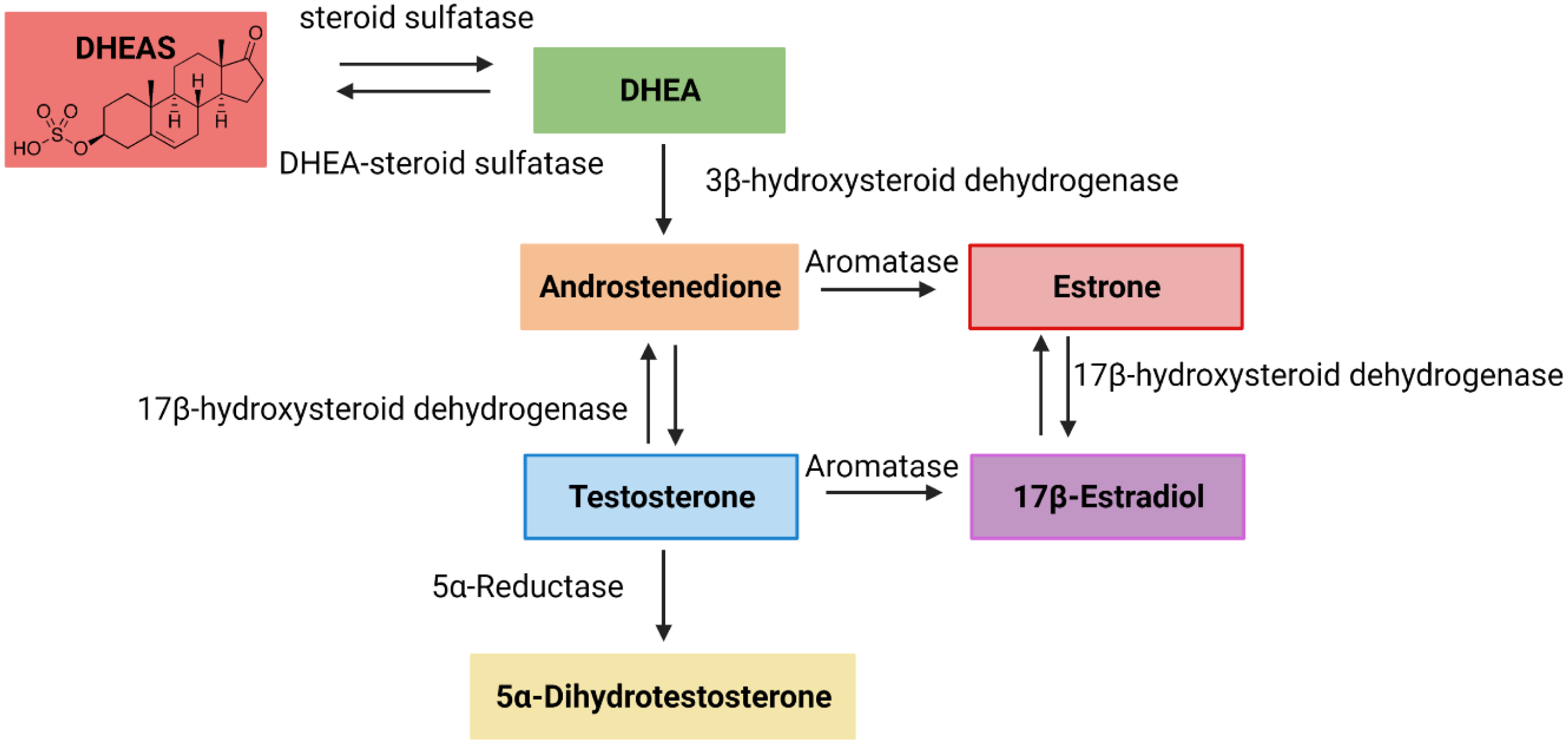
Fig. 1. Schematic representation of converting dehydroepiandrosterone sulfate (DHEAS) into testosterone and estrogen. The concept for the image was adopted from Allolio and Arlt (Reference Allolio and Arlt2002).
DHEAS, the sulfated derivative of DHEA, is a steroid hormone produced mainly by the adrenal cortex, the outer layer of the adrenal glands. Osawa et al. (Reference Osawa, Nakajima, Yoshida, Omura, Nagase, Ueno, Wada, Matsuhashi, Ochiai, Nakagama and Sekihara2002) have found that DHEA increases the number of aberrant crypt foci induced by azoxymethane in a murine model without progression to carcinogenesis, suggesting that DHEA may be a promising chemopreventive drug for colon cancer. DHEAS activates ERK1/2 in MCF-7 breast cancer cells (Upmanyu et al., Reference Upmanyu, Bulldan, Failing and Scheiner-Bobis2020). The ERK 1/2 cascade is a central signaling pathway that regulates various biological processes, including proliferation, differentiation, survival, apoptosis and stress response (Wortzel and Seger, Reference Wortzel and Seger2011).
In 2000, Alberg et al. (Reference Alberg, Gordon, Hoffman, Comstock and Helzlsouer2000) conducted a nested case–control analysis to evaluate whether serum DHEAS is associated with the likelihood of developing colon cancer. The results did not provide strong evidence for an association between circulating DHEAS and colon cancer risk. Nevertheless, DHEAS was associated with a decreased risk of colon cancer among men, and these results were not statistically significant under 95% CIs (p = .06). Therefore, further studies are required to support or refute the potential association between DHEAS and colorectal cancer.
MR is an epidemiological technique that leverages genetic data to provide evidence on causal relationships between pairs of complex traits. The variants must be robustly associated with the exposure as instrumental variables to predict the effect of the exposure (here DHEAS) on disease risk (colorectal cancer in this study). MR can be compared to a randomized controlled trial as it takes advantage of random allocation of genetic variants at conception (Larsson et al., Reference Larsson, Kar, Perry, Carter, Vithayathil, Mason, Easton and Burgess2022), while observational studies are susceptible to confounding. In this study, we have used a two-sample MR approach to assess the potential causal association of DHEAS with colorectal cancer. In two-sample MR, the associations of the instrumental variables with the exposure and outcome are derived from two independent (nonoverlapping) samples.
Materials and Methods
This study followed the STROBE-MR (strengthening the reporting of observational studies in epidemiology using MR) (Skrivankova et al., Reference Skrivankova, Richmond, Woolf, Davies, Swanson, VanderWeele, Timpson, Higgins, Dimou, Langenberg, Loder, Golub, Egger, Davey Smith and Richards2021) guidelines to report MR results.
Summary Data Resources
DHEAS
The genomewide association study (GWAS) summary statistics of DHEAS (adjusted for sex, age and log [BMI]) was obtained from Pott et al. (Reference Pott, Bae, Horn, Teren, Kühnapfel, Kirsten, Ceglarek, Loeffler, Thiery, Kratzsch and Scholz2019). In their study, GWAS analyses were performed using two cohorts of the Leipzig Research Centre for Civilization Diseases, Life-Adult (5758 samples: 2969 men and 2789 women) and Life-Heart (2070 samples: 1358 men and 712 women), after excluding all patients under treatment of sex hormones or glucocorticoids. Both Life-Adult and Life-Heart studies comprise randomly assigned participants from Leipzig, Germany. In LIFE-Heart and Life-Adult, the DHEAS levels were measured using liquid chromatography–tandem mass spectrometry and an electrochemiluminescence immunoassay. The unit of DHEAS measurement was μmol/L.
Colorectal cancer
The GWAS summary statistics of colorectal cancer were obtained from a recent study that included 4562 cases and 382,756 controls of white British ancestry (Zhou et al., Reference Zhou, Nielsen, Fritsche, Dey, Gabrielsen, Wolford, LeFaive, VandeHaar, Gagliano, Gifford, Bastarache, Wei, Denny, Lin, Hveem, Kang, Abecasis, Willer and Lee2018).
DHEAS and colorectal cancer GWAS summary statistics were obtained from two independent studies to avoid overlapping samples.
Instruments Selection
Summary statistics for 467 genetic variants (single nucleotide polymorphisms; SNPs) associated with DHEAS levels at genomewide significance (p < 5E-08 and MAF > 0.01) were extracted from the original DHEAS GWAS study (Pott et al., Reference Pott, Bae, Horn, Teren, Kühnapfel, Kirsten, Ceglarek, Loeffler, Thiery, Kratzsch and Scholz2019). To ensure instrumental variables were independent, strict clumping was performed with an r 2 threshold of .001 and a window size of 10,000 kb using the ‘ld_clump’ function in the TwoSampleMR package (Hemani et al., Reference Hemani, Zheng, Elsworth, Wade, Haberland, Baird, Laurin, Burgess, Bowden, Langdon, Tan, Yarmolinsky, Shihab, Timpson, Evans, Relton, Martin, Davey Smith, Gaunt and Haycock2018). Six instrumental variables (SNPs) were retrieved by the linkage disequilibrium pruning. We queried both PhenoScanner v2 (http://www.phenoscanner.medschl.cam.ac.uk/ accessed on February 8, 2022) and GWAS Catalog (https://www.ebi.ac.uk/gwas/home accessed on February 8, 2022) to investigate the potential confounding effect (p < 5E-08) among instrumental variables and their proxies (r 2 > .8). Particularly, we checked obesity-related traits (BMI index, waist circumference, hip circumference and weight; Doleman et al., Reference Doleman, Mills, Lim, Zelhart and Gagliardi2016), physical activity (Shaw et al., Reference Shaw, Farris, Stone, Derksen, Johnson, Hilsden, Friedenreich and Brenner2018), smoking (Botteri et al., Reference Botteri, Borroni, Sloan, Bagnardi, Bosetti, Peveri, Santucci, Specchia, van den Brandt, Gallus and Lugo2020), alcohol consumption (Y. Kim et al., Reference Kim, Je and Giovannucci2019), inflammatory bowel disease (Nørgaard et al., Reference Nørgaard, Farkas, Pedersen, Erichsen, de la Cour, Gregersen and Sørensen2011) and Crohn’s disease (Olén et al., Reference Olén, Erichsen, Sachs, Pedersen, Halfvarson, Askling, Ekbom, Sørensen and Ludvigsson2020), as reported in the literature. One SNP, rs117978821, was removed as it was associated with weight-related traits. These rigorously selected SNPs were used in the subsequent MR analyses.
MR Analysis
We used four complementary two-sample MR approaches, inverse-variance weighted (IVW), MR-Egger, weighted median and weighted mode, to estimate the causal effect of DHEAS on colorectal cancer. These methods have been derived under different assumptions of horizontal pleiotropy. The IVW method assumes no/balanced pleiotropy. The IVW estimate is calculated by meta-analyzing Wald ratio values of the individual instrumental variable. We used Cochran’s statistic (Q ∼
$\chi _{L - 1}^2$
, where L is the number of instrumental variables) to check the presence of heterogeneity, which can indicate pleiotropy under the null hypothesis of homogeneity.
MR-Egger regression is based on the INSIDE assumption (instrument strength independent of the direct effects). According to INSIDE, the instrumental variables’ pleiotropic effects should be independent instrumental variables’ association with the exposure. In addition to INSIDE, MR-Egger is also based on the no measurement error (NOME), where genetic associations with exposure of interest are measured without error. The regression dilution can evaluate the NOME assumption (
$I_{GX}^2)$
. When 0.6 <
$\;I_{GX}^2$
< 0.9, the simulation extrapolation (SIMEX) correlation analysis is used to estimate the causal effect. If
$I_{GX}^2$
< 0.6, MR-Egger and SIMEX are unreliable, so neither is performed (Vermeulen et al., Reference Vermeulen, Wootton, Treur, Sallis, Jones, Zammit, van den Brink, Goodwin, de Haan and Munafò2021). The intercept term of the Egger regression method is considered an estimate of the directional pleiotropic effect. The Rucker’s Q’ statistic (Q ∼
$\chi _{L - 2}^2$
, where L is the number of instrumental variables) was used to evaluate the heterogeneity in the MR-Egger method. In general, Rucker’s Q’ is less than or equal to Cochran’s Q. If the difference of Q statistics, Q-Q’, is sufficiently large with respect to a
$_{1\;}^2$
(3.84), we would infer that directional pleiotropy is an important factor and the MR-Egger model provides a better model than the IVW method.
Compared to IVW and MR-Egger, the weighted median method is more robust even when 50% of SNPs are invalid instruments. The weighted mode approach provides a consistent estimate when the largest number of similar instrumental variables come from valid instruments, even though the majority (>50%) are invalid. We used the MR pleiotropy residual sum and outlier (MR-PRESSO) global test to detect horizontal pleiotropy. Moreover, F statistics and the proportion of variance (R 2) were calculated to assess instrumental variables’ strength (Rees et al., Reference Rees, Wood and Burgess2017). Staiger and Stock (Reference Staiger and Stock1997) suggested that the F statistic of instrumental variables should be larger than 10 to ensure that the maximum bias in instrumental variable estimators is less than 10%. The TwoSampleMR R package (Hemani et al., Reference Hemani, Zheng, Elsworth, Wade, Haberland, Baird, Laurin, Burgess, Bowden, Langdon, Tan, Yarmolinsky, Shihab, Timpson, Evans, Relton, Martin, Davey Smith, Gaunt and Haycock2018) was utilized for all analyses explained in the Methods section except
$I_{GX}^2$
calculation, which was performed using the MendelianRandomization R package (Yavorska and Burgess, Reference Yavorska and Burgess2017).
Sensitivity Analysis
Leave-one-out sensitivity analyses were carried out with significant MR analysis results to check whether the causal association is driven by a single SNP.
Results
Two Sample MR Analysis Results
The proportion of variance (R 2), F statistics and Wald estimates were calculated for each instrumental variable (Table 1). The combined multi-allelic instrument has explained 2.97% of the variation in DHEAS levels.
Table 1. F statistic values and Wald ratio estimates for individual instrumental variables

Note: SE, standard error; CRC, colorectal cancer; DHEAS, dehydroepiandrosterone sulfate
As shown in Table 1, the F statistic is greater than 10 for all the instrumental variables. IVW estimate exhibits an inverse association between DHEAS level and colorectal cancer risk (OR = 0.70; 95% CI [0.51, 0.96]; p = .03). According to Cochran’s Q statistic (Q = 5.47; p = .24), homogeneity was observed among individual IVW causal estimates. We did not fit MR-Egger models as
$I_{GX}^2\;$
was close to zero. The Q’ (3.45; p = .33) and Q-Q’ (2.02; p value = .16) test statistics and respective p values indicated that the IVW method provides a better estimate. MR-PRESSO global test showed no horizontal pleiotropy among individual SNPs of DHEAS for colorectal cancer (p = .31), strengthening the selection of the IVW method to estimate the causal effect of DHEAS on colorectal cancer. Moreover, causal effects found from weighted median (OR = 0.70; 95% CI [0.49, 1.00]; p = .05) and weighted mode (OR = 0.69; 95% CI [0.41, 1.15]; p = .23) were consistent with IVW causal estimate (see Table 2).
Table 2. MR causal estimate of DHEAS on colorectal cancer

Note: DHEAS, dehydroepiandrosterone sulfate; SNP, single nucleotide polymorphism.
Results in Table 2 (OR = 0.70; 95% CI [0.51, 0.96]; p = .03) and Figure 2 (IVW regression line in light blue color) imply an inverse relationship between DHEAS level and colorectal cancer risk.

Fig. 2. A scatter plot representation relating the effect sizes of SNP (single nucleotide polymorphism)-DHEAS (dehydroepiandrosterone sulfate) association (x-axis, in standard deviation units) and SNP-colorectal cancer (y-axis) with standard error bars. The gradients of the lines correspond to causal estimates using each of the three different methods.
Sensitivity Analyses (Leave-One-Out Analysis)
We used the leave-one-out analysis to check whether the SNPs are inconsistent in their effect on the overall outcome or whether a single outlying SNP is driving the results. Figure 3 illustrates the results of MR analyses using the IVW method when leaving one SNP out each time. Figure 3 shows a consistent effect size at the exclusion of most SNPs (three out of five, rs28620926, rs29001546 and rs296360).

Fig. 3. Leave-one-out analysis using inverse-variance weighted (IVW) method. Each black point represents the IVW causal effect estimation of dehydroepiandrosterone sulfate (DHEAS) on colorectal cancer, excluding that particular variant (labeled on the y-axis) from the analysis. The black point with a red error bar depicts the IVW estimate using all single nucleotide polymorphisms (SNPs). Each point of the plot has been labeled with effect sizes and their respective p values (within brackets).
Discussion
Colorectal cancer is one of the most common cancers in Western society, with a poor prognosis for patients in the advanced stages. Emerging evidence shows that sex hormones such as estrogen and testosterone may play an important role in colorectal cancer risk. Nevertheless, the role of DHEAS, the precursor of estrogen and testosterone, was unclear. We conducted the first MR study that assesses the causal effect of endogenous DHEAS levels on colorectal cancer risk.
The MR analysis was conducted under three main assumptions. These assumptions should be satisfied to select genetic variants as instrumental variables: (1) the selected instrumental variables are robustly associated with the exposure; (2) the instrumental variables are not associated with confounding factors; and (3) the instrumental variables influence the outcome only through the exposure (no horizontal pleiotropy exists). Initially, we selected genetic variants at the genomewide significance level (5E-08) to reflect a strong association with the exposure, DHEAS. Furthermore, we calculated the F statistic for the selected SNPs. All instrumental variables have F statistic >10, satisfying the first assumption. We have minimized the violation of the second assumption by examining previously reported associations of instrumental variables. One genetic variant, rs117978821, was removed as it was associated with weight-related traits (BMI is considered a potential risk factor in colorectal cancer). These findings may be limited as there can be undiscovered risk factors of colorectal cancer. Herein, we did not perform MR-Egger (intercept analysis) as
$I_{GX}^2\;$
was too low. Nevertheless, weighted median, Cochran’s Q, the difference of Q (Q-Q’) and MR-PRESSO test statistics detected the no/balanced horizontal pleiotropy satisfying the third assumption. Therefore, the IVW method was selected to estimate the causal effect.
In the present study, IVW estimates exhibit a significant association between DHEAS levels and decreased colorectal cancer risk. Lin et al. (Reference Lin, Zhang, Rexrode, Manson, Chan, Wu, Tworoger, Hankinson, Fuchs, Gaziano, Buring and Giovannucci2013) reported that testosterone levels and the ratio of estradiol to testosterone were associated with decreased colorectal cancer risk among men and women, respectively. Their results suggest that men with lower androgenicity due to reduced androgen receptor activity or lower circulating DHEAS are at higher risk of colorectal cancer. Yang et al. (Reference Yang, Giovannucci, Hankinson, Chan, Ma, Wu, Fuchs, Lee, Sesso, Lin and Zhang2020) have found that higher circulating testosterone levels are associated with lower colorectal cancer risk among men, but higher estrone levels show increased colorectal risk among women. Previous studies have suggested an inverse relationship between testosterone and colorectal cancer risk among men, but the association between estrogen and colorectal cancer was not consistent among women. Our study was conducted using DHEAS GWAS summary statistics adjusted for the sex variable, and an inverse association was found between DHEAS and colorectal cancer. Moreover, Alberg et al. (Reference Alberg, Gordon, Hoffman, Comstock and Helzlsouer2000) have mentioned that DHEAS was associated with a decreased risk of colon cancer among men, and these results were within the bounds of chance. Our outcome strengthens Alberg et al.’s (Reference Alberg, Gordon, Hoffman, Comstock and Helzlsouer2000) results through the negative causal effect of DHEAS on colorectal cancer.
In addition to colorectal cancer, DHEA (desulfated version of DHEAS)/DHEAS shows an association with other hormonal cancers such as prostate (Kim et al., Reference Kim, Ryan, Zhang, Youngren, Wilton, Alumkal, Beer, Baertsch, Stuart, Chi, Gleave, Rettig, Reiter, Lara, Evans and Small2016), breast (Hankinson and Eliassen, Reference Hankinson and Eliassen2010; Tworoger et al., Reference Tworoger, Missmer, Eliassen, Spiegelman, Folkerd, Dowsett, Barbieri and Hankinson2006), ovarian (Mizushima and Miyamoto, Reference Mizushima and Miyamoto2019) and endometrial (Michels et al., Reference Michels, Brinton, Wentzensen, Pan, Chen, Anderson, Pfeiffer, Xu, Rohan and Trabert2019) cancers. Kim et al. (Reference Kim, Ryan, Zhang, Youngren, Wilton, Alumkal, Beer, Baertsch, Stuart, Chi, Gleave, Rettig, Reiter, Lara, Evans and Small2016) have explained DHEA as a potential biomarker in metastatic castration-resistant prostate cancer. Nevertheless, case–control, cohort or MR studies were not found that investigated the DHEAS-prostate cancer association. Tworoger et al. (Reference Tworoger, Missmer, Eliassen, Spiegelman, Folkerd, Dowsett, Barbieri and Hankinson2006) described that DHEAS was associated with increased estrogen receptor/progesterone receptor-positive breast cancer risk in predominantly postmenopausal women. Later on, Hankinson and Eliassen (Reference Hankinson and Eliassen2010) explained that DHEA/S was associated with breast cancer risk among postmenopausal women and no significant association among premenopausal women. Similarly, Michels et al. (Reference Michels, Brinton, Wentzensen, Pan, Chen, Anderson, Pfeiffer, Xu, Rohan and Trabert2019) have found that compared to the lowest level, the highest DHEA level (5th vs. 1st quintile: OR = 1.85; 95% CI [1.06, 3.25]) was associated with increased endometrial cancer risk among postmenopausal women. In early studies, Cuzick et al. (Reference Cuzick, Bulstrode, Stratton, Thomas, Bulbrook and Hayward1983; p = .007) and Helzlsouer et al. (Reference Helzlsouer, Alberg, Gordon, Longcope, Bush, Hoffman and Comstock1995; p = .02) showed that DHEA levels were significantly higher in ovarian cancer patients than in control subjects. In contrast, later studies did not find a significant difference in blood levels of DHEA or DHEAS between ovarian cancer patients and matched controls (Lukanova et al., Reference Lukanova, Lundin, Akhmedkhanov, Micheli, Rinaldi, Zeleniuch-Jacquotte, Lenner, Muti, Biessy, Krogh, Berrino, Hallmans, Riboli, Kaaks and Toniolo2003; Ose et al., Reference Ose, Fortner, Rinaldi, Schock, Overvad, Tjonneland, Hansen, Dossus, Fournier, Baglietto, Romieu, Kuhn, Boeing, Trichopoulou, Lagiou, Trichopoulos, Palli, Masala, Sieri and Kaaks2015; Rinaldi et al., Reference Rinaldi, Dossus, Lukanova, Peeters, Allen, Key, Khaw, Trichopoulos, Trichopoulou, Oikonomou, Pera, Larrañaga, Martinez-Garcia, Ardanaz, Quirós, Tormo, Tjønneland, Olsen, Overvad, Chang-Claude and Kaaks2007; Tworoger et al., Reference Tworoger, Lee, Buring and Hankinson2008). These studies imply that DHEA/DHEAS plays an important role in multiple hormonal cancers.
There are several limitations of the current study that should be acknowledged. The present study used GWAS summary statistics from the cohorts that dominated European ancestry; hence, results may not be generalized to other populations. Moreover, MR captures lifelong exposure to DHEAS; the MR effect may not be similar to the short-term effect of randomized clinical trials. Therefore, the effect of DHEA/DHEAS on colorectal cancer still needs to be studied in intervention studies. Herein, we conducted MR analysis for sex-adjusted DHEAS GWAS summary statistics as a sufficient number of SNPs were not retained by men- or women-specific DHEAS GWAS. Though DHEAS GWAS summary data are adjusted for sex groups, the potential residual effect of this covariate cannot be excluded, which may violate the first assumption that instrumental variables are robustly related to the exposure. Moreover, owing to the lack of data, the original DHEAS GWAS study has not been controlled for the menstrual cycle and using birth control methods. In leave-one-out sensitivity analyses, the exclusion of only one SNP was statistically significant under the 5% significance level (all five were significant at the 10% threshold), showing that results are influenced by the small number of SNPs used in the analysis. A lower number of SNPs can also affect the utility of other MR methods as testing heterogeneity is robust with higher SNPs.
Besides these limitations, this study provides a new direction for hormone-colorectal cancer risk association studies. DHEAS acts as the precursor of estrogen and testosterone. Unless DHEAS has a more significant effect than estrogen/testosterone on colorectal cancer or DHEAS is a more straightforward supplementation than estrogen or testosterone, DHEAS/estrogen/testosterone will still be valuable interventions.
Conclusions
Using publicly available GWAS summary statistics, we conducted two-sample MR analyses to investigate the causal effect of DHEAS on colorectal cancer. We identified an inverse relationship between DHEAS (adjusted for sex variable) and colorectal cancer risk. Nevertheless, MR explains lifelong exposure to DHEAS, which may not be analogous to the short-term effect of randomized clinical trials. Moreover, this study is based on data retrieved from the European ancestry-based databases and may not be extrapolated to other ancestries or populations. Apart from these limitations, this study provides a direction for future hormone-cancer risk association studies. Future MR analyses should focus on gender-based DHEAS-colorectal cancer association among different ancestries/populations.
Data Availability Statement
Publicly available DHEAS GWAS summary statistics were obtained from the Leipzig Health Atlas (https://zenodo.org/record/5702746#.YaYG0boxnmE). The colorectal cancer GWAS summary statistics were downloaded from the GWAS Catalog (https://www.ebi.ac.uk/gwas/). PhenoScanner v2 (http://www.phenoscanner.medschl.cam.ac.uk/) and GWAS Catalog (https://www.ebi.ac.uk/gwas/home) were used to identify previously reported traits/diseases for instrumental variables in interest. All statistical analyses and graph preparations were performed using the TwoSampleMR R package, and installation instructions and links are freely available at https://github.com/MRCIEU/TwoSampleMR.
Acknowledgments
The computational resources and services used in this work were provided by the eResearch Office, Queensland University of Technology, Brisbane, Australia.
Author Contributions
Conceptualization: D.K.J, N.S.G., M.E.R., and P.F.K.; methodology: D.K.J. and P.F.K.; formal analysis: D.K.J., writing-original draft: D.K.J.; writing-review and editing: D.K.J., N.S.G., M.E.R., P.F.K. and J.B.; supervision: N.S.G., M.E.R., and J.B. All authors read and approved the final manuscript.
Financial Support
This research was supported by QUT postgraduate research allowance (QUTPRA), QUT HDR tuition fee sponsorship, Advance Queensland Industry Research Fellowship; the NHMRC Career Development Fellowship; and the Cancer Council Queensland grant.
Conflict of Interest
None.
Ethical standards
This study was performed in line with the principles of the Declaration of Helsinki. Approval was granted by the Ethics Committees of Queensland University of Technology (December 19, 2019/1900001147) and QIMR Berghofer Medical Research Institute (August 23, 2019/P1051).