INTRODUCTION
For the conversion of radiocarbon (14C) ages of last glacial-to-early-deglacial times into calendar age equivalents we are faced with the problem of finding two differently focused, in part intimately interrelated empirical data sets that compare 14C years with paired calendar years as outlined further below.
The IntCal20 calibration curve with its 1σ standard deviation predictive envelope provides the best-possible conversion of atmosphere-related 14C ages to calendar age equivalents back to 55 cal. ka BP that is presently available. Back to ∼14 or ∼15 cal ka BP, it relies on the tree ring-based “master records” of atmospheric 14C concentration (Reimer et al. Reference Reimer, Austin, Bard, Bayliss, Blackwell, Ramsey, Butzin, Cheng, Edwards and Friedrich2020). Prior to ∼15 cal ka BP and the continuous tree-ring record, IntCal20 uses a Bayesian spline to integrate evidence from a broad mixture of different 14C records (Reimer et al. Reference Reimer, Austin, Bard, Bayliss, Blackwell, Ramsey, Butzin, Cheng, Edwards and Friedrich2020). On the one hand, the IntCal20 (international consensus) calibration curve thus incorporates atmospheric 14C ages of (short) floating tree-ring records and lacustrine sediment records based on carefully picked plant macrofossils for the record of Lake Suigetsu (Bronk Ramsey et al. Reference Bronk Ramsey, Heaton, Schlolaut, Staff, Bryant, Lamb, Marshall and Nakagawa2020).
On the other hand, IntCal20 is strongly controlled by many 14C records of marine sediment piles (hardly laminated), corals, and speleothem sections. Several carbonate-based records have the great advantage of paired U/Th age control (e.g., those from Hulu Cave; Cheng et al. Reference Cheng, Edwards, Southon, Matsumoto, Feinberg, Sinha, Zhou, Li, Li and Xu2018), but widely suffer from, in part little known, variable short-term 14C offsets either from the coeval atmospheric concentration due to variable marine reservoir ages or from changes in the soil reservoir effect of cave deposits. Those variables are only rarely constrained by multiple precise data series (Wang et al. Reference Wang Zh and Cao2022). The carbonate-based indirect atmospheric 14C records show less fine structure than the Suigetsu record.
Problems to refine and correlate the outlined different time series were overcome by new statistical analyses, using a Bayesian spline procedure that also incorporates calendar age uncertainty and an additive error term on the 14C determinations to provide a statistically robust calibration curve (with uncertainties no greater than on previous iterations). As a result, most fine-scale fluctuations in the relationship between 14C concentration and cal. age estimates are smoothed out and/or treated as “noise” in IntCal20 beyond the range of tree-ring calibration (Reimer et al. Reference Reimer, Austin, Bard, Bayliss, Blackwell, Ramsey, Butzin, Cheng, Edwards and Friedrich2020).
Prior to ∼15 cal ka BP, the Suigetsu 14C record, although a component of IntCal20, forms the only direct and quasi-continuous record of atmospheric 14C concentrations free of any potential inherent variations of reservoir and dead-carbon age back to >35 cal ka BP (a limit chosen for practical use in this paper). Originally optical varve counts of Suigetsu sediment sections had been considered as a valuable tool to generate an independent line of evidence of absolute age control, a great perspective (Schlolaut et al. Reference Schlolaut, Staff, Marshall, Brauer, Bronk Ramsey, Lamb and Nakagawa2018). This approach was proven false by further independent age information outlined below.
Sections between 17.285 and 40.408 m core depth with weak-and-thin or no lamination resulted in different calendar ages for varve counts by microscopy and μXRF (micro-X-Ray fluorescence) and necessitated a varve interpolation program to derive a time scale down to 40 m (Schlolaut et al. Reference Schlolaut, Staff, Marshall, Brauer, Bronk Ramsey, Lamb and Nakagawa2018). Sarnthein et al. (Reference Sarnthein, Küssner, Grootes, Ausin, Eglinton, Muglia, Muscheler and Schlolaut2020) tested the two varve timescales using as test case the age of the lower end of 14C plateau no. 2b, the oldest 14C structure and tie point that can be constrained by μXRF-based varve counts (Marshall et al. Reference Marshall, Schlolaut, Nakagawa, Lamb, Brauer, Staff, Bronk Ramsey, Tarasov, Gotanda, Haraguchi, Yokohama, Yonenobu and Tada2012). In contrast to an age of 16.4 cal ka BP proposed by optical varve counts (Schlolaut et al. Reference Schlolaut, Staff, Marshall, Brauer, Bronk Ramsey, Lamb and Nakagawa2018), the μXRF-based counts reveal an age of ∼16.9 cal ka BP. This value matches directly a third, independent age estimate, the U/Th model-based Suigetsu timescale with an age estimate of 16.94 cal ka BP (Bronk Ramsey et al. Reference Bronk Ramsey, Heaton, Schlolaut, Staff, Bryant, Lamb, Marshall and Nakagawa2020). This point-match and the increasing undercount of optical varves with age (Bronk Ramsey et al. Reference Bronk Ramsey, Heaton, Schlolaut, Staff, Bryant, Lamb, Marshall and Nakagawa2020; Sarnthein et al. Reference Sarnthein, Küssner, Grootes, Ausin, Eglinton, Muglia, Muscheler and Schlolaut2020; Figure 3) clearly support the use of the latter ages for the Suigetsu record as “best possible” approximation.
Box 1 Definition of atmospheric 14C plateaus derived from tree rings and the Lake Suigetsu sediment section (following Sarnthein et al. Reference Sarnthein, Grootes, Kennett and Nadeau2007, Reference Sarnthein, Balmer, Grootes and Mudelsee2015, Reference Sarnthein, Küssner, Grootes, Ausin, Eglinton, Muglia, Muscheler and Schlolaut2020).
-
(1) The term “14C plateau” is assigned to a set of largely constant atmospheric 14C ages over a range extending over 250/400 to 1200 consecutive cal yr. This leads to a horizontal, in part slightly oblique scatter band of atmospheric 14C ages (Figure 1), in the Lake Suigetsu section measured with a time resolution of 8–20, rarely up to 35 14C data per 1000 yr.
-
(2) 14C age is assumed constant over the full period of the plateau (“horizontal” plateau) because data resolution is insufficient to define a statistically significant true 14C slope over the plateau period.
-
(3) The upper and lower margin of the plateau (Y-axis) are chosen such that 90% of the 14C ages are within these boundaries (1.6 σ criterion; in Figure 1 delineated by horizonal lines).
-
(4) From 10 to 21 cal. ka, the typical scatter/uncertainty range of an average plateau 14C age amounts to ±100–250 14C yr, at cal ages of >21 cal ka BP, especially >23 cal. ka, the uncertainty range is rising up to ±500 yr due to an increased analytical error.
-
(5) Most lower and upper cal age boundaries (X-axis) of an atmospheric 14C plateau are identified visually by 14C age jumps, that form tipping points of the 14C curve, where the 14C curve is crossing the horizontal border lines assigned to the upper and lower margins of the plateau’s uncertainty range, hence is deviating beyond the scatter band of a 14C plateau.
-
(6) The rapid change in 14C age over cal. age at plateau boundaries is reflected by a distinct maximum of the 1st-derivative of the curve for the 14C age vs. cal age record. The 1st-derivative thus provides a second, statistical plateau identification.
-
(7) The cal. age of most atmospheric plateau boundaries, assigned halfway between the two nearest 14C ages, can be constrained with a precision of ±50 to ±70 yr on the basis of Suigetsu 14C ages calibrated by U/Th model ages of Bronk Ramsey (Reference Bronk Ramsey, Heaton, Schlolaut, Staff, Bryant, Lamb, Marshall and Nakagawa2020).
In summary, we followed Bronk Ramsey et al. (Reference Bronk Ramsey, Heaton, Schlolaut, Staff, Bryant, Lamb, Marshall and Nakagawa2020) and finally replaced the ages based on optical varve counts by the updated U/Th-based model ages deduced from a complex correlation scheme to U/Th-based ages of the Hulu speleothem record (Bronk Ramsey et al. Reference Bronk Ramsey, Heaton, Schlolaut, Staff, Bryant, Lamb, Marshall and Nakagawa2020; ages updated by dataset of Cheng et al. Reference Cheng, Edwards, Southon, Matsumoto, Feinberg, Sinha, Zhou, Li, Li and Xu2018).
Different from the widely smoothed IntCal20 curve, the Suigetsu record of atmospheric 14C concentrations ∼10 to ∼35 cal ka BP appears to display a whole suite of fairly distinct centennial-to-millennial-scale fluctuations. Their quality, authenticity, and origin are the topic of this study that mainly re-evaluates data sets recently published (Bronk Ramsey et al. Reference Bronk Ramsey, Heaton, Schlolaut, Staff, Bryant, Lamb, Marshall and Nakagawa2020). If confirmed, these fluctuations, at least the 14C plateau boundaries, will provide a suite of atmospheric, thus global stratigraphic tie points to correlate glacial-to-deglacial records of changes in climate and ocean. In particular, the age tie points provided by these 14C structures will reduce the problem of properly assigning cal. age estimates by interpolation over long 14C plateau periods with varying climate and sedimentation conditions down to the scale of a 14C plateau. During LGM and early deglacial times, however, long, largely horizontal scatter bands of approximately constant 14C ages may span up to 1200 yr. Also, the suite of structures per se may provide tie points for initial age correlation (Sarnthein et al. Reference Sarnthein, Grootes, Kennett and Nadeau2007, Reference Sarnthein, Küssner, Grootes, Ausin, Eglinton, Muglia, Muscheler and Schlolaut2020).
THE SUIGETSU ATMOSPHERIC 14C RECORD—DATA BASE
The noisy Suigetsu 14C record is based on an average macrofossil sample density of ∼8 to ∼20, rarely 35 14C dates per thousand years (kyr), as compared to a modeled time resolution of 200 14C ages/cal. kyr for IntCal20 (Reimer et al. Reference Reimer, Austin, Bard, Bayliss, Blackwell, Ramsey, Butzin, Cheng, Edwards and Friedrich2020). The glacial-to-deglacial Suigetsu record of 14C ages between 14 and 28 cal ka BP (Figure 1) is not a straight slope line but shows a suite of sections with no or little slope, other sections with a rapid decrease in 14C age with decreasing calendar age, labeled “plateaus” and “jump” structures. Comparison with 14C structures displayed by the tree-ring record 10–15 cal ka BP (Figure 3) shows close similarity making the probability that the Suigetsu 14C structures might result from chance deposition low. The significance of these Suigetsu centennial to millennial-scale features beyond the tree ring record and 15 cal ka BP is under debate since their signal-to-noise ratio in the record is low and, in some cases, does not allow rejection of the null hypothesis assuming a straight line for the correlation of 14C and cal age data (Bard and Heaton Reference Bard and Heaton2021).
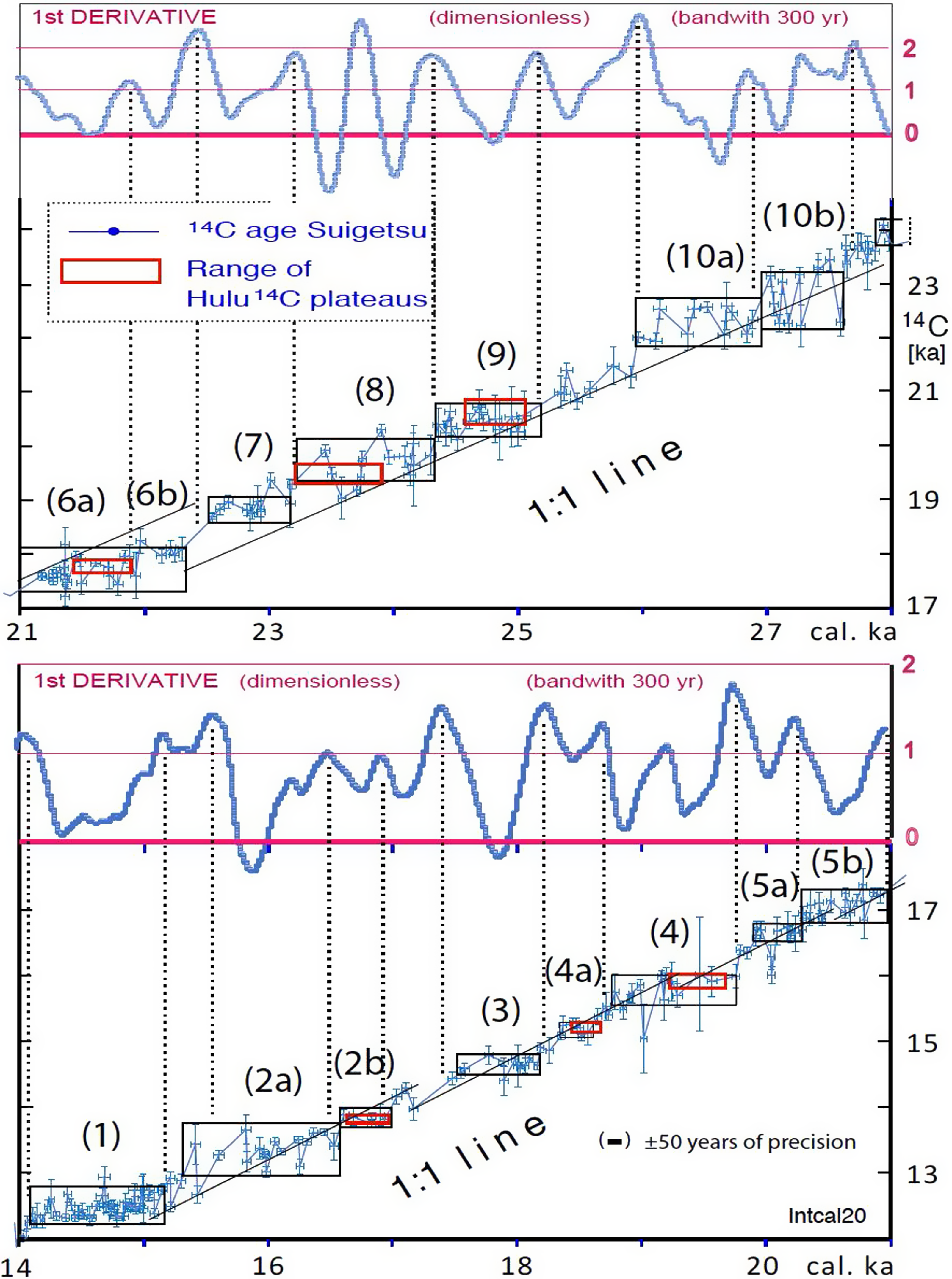
Figure 1 Atmospheric 14C ages and error bars of Lake Suigetsu plant macrofossils vs. U/Th-based model age of 15–21 (bottom) and 21–27 (top) cal ka BP (blue dots; Sarnthein et al. Reference Sarnthein, Küssner, Grootes, Ausin, Eglinton, Muglia, Muscheler and Schlolaut2020, modified, using age data of Bronk Ramsey et al. Reference Bronk Ramsey, Heaton, Schlolaut, Staff, Bryant, Lamb, Marshall and Nakagawa2020). 14C plateaus longer than 400 yr (in case of plateau 4a: 250 yr) are outlined by a suite of labeled horizontal boxes that envelop scatter bands of largely constant or slightly rising 14C ages, separated by “14C jumps,” statistically best defined by maximum spikes in the 1st derivative plot and 1σ uncertainty range. For rules of plateau definition see Text Box 1.
Plateaus extend over ∼400 to ∼1200 yr each, some being less, some more distinct (Sarnthein et al. Reference Sarnthein, Küssner, Grootes, Ausin, Eglinton, Muglia, Muscheler and Schlolaut2020), where the lower value of (300 to) 400 yr has been dictated by the 14C sample density of hemipelagic marine sediment sections from all over the ocean (in contrast to the much higher sample resolution of the Suigetsu lacustrine record discussed here), moreover by the 14C measuring uncertainty in this age range. The question is in which way this apparent centennial-to-millennial-scale structure of the Suigetsu 14C record can be identified objectively and reproducibly. In the 14C age domain the suite of 14C age fluctuations has been defined by visual inspection of “age plateaus” (rules of definition described in the Text Box 1; Sarnthein et al. Reference Sarnthein, Grootes, Kennett and Nadeau2007) giving a result fully congruent with structures based on the first derivative of the 14C age-to-calendar age relationship and its 1σ uncertainty range, obtained by using a running kernel window (Sarnthein et al. Reference Sarnthein, Balmer, Grootes and Mudelsee2015).
An independent approach plots the Suigetsu atmospheric 14C record for the period 14–30 cal ka BP as Δ14C deviations from the standard atmosphere vs. IntCal20 cal age (Figure 2). The curve is here defined by a Bayesian spline (Bronk Ramsey et al. Reference Bronk Ramsey, Heaton, Schlolaut, Staff, Bryant, Lamb, Marshall and Nakagawa2020; Bard and Heaton Reference Bard and Heaton2021). All in all, periods of gradually decreasing Δ14C values are reflecting 15 atmospheric 14C age plateaus (blue numbers) and their 1σ-uncertainty range. In between, (rapid) rises in Δ14C are directly compared with 14C age “jump”’ between plateaus. In Figure 2 these inflections in the Bayesian spline directly face the Suigetsu-based plateau and jump structures as derived by visual inspection and by the first derivative of 14C ages in the time domain, here plotted as dark pink line segments in the Δ14C domain (Supplementary Table 1S). In general, the two presentations agree well especially considering the exaggerated jumps in atmospheric 14C concentration induced by the “simplifying” assumption of an on-average constant Suigetsu 14C age over a “horizontal” 14C scatter band of a plateau-episode. This assumption is needed where insufficient data was available to say, whether very short-term 14C changes within the plateau may be real.

Figure 2 Raw 14C data of Lake Suigetsu (blue dots), converted into the deviation of atmospheric 14C concentration from the (modern) standard atmosphere (in ‰ Δ14C units), plotted vs. cal. ages of Bronk Ramsey et al. (Reference Bronk Ramsey, Heaton, Schlolaut, Staff, Bryant, Lamb, Marshall and Nakagawa2020). A Bayesian spline named “Suigetsu only curve” (pink band) (Bard and Heaton Reference Bard and Heaton2021, Figure 3a modified) shows periods of gradually decreasing atmospheric Δ14C values, reflecting 15 atmospheric 14C age plateaus and their uncertainty range as defined and listed by Sarnthein et al. (Reference Sarnthein, Küssner, Grootes, Ausin, Eglinton, Muglia, Muscheler and Schlolaut2020; Table 1). In between, rapidly increasing atmospheric Δ14C values reflect short gaps and/or 14C age “jumps” between plateaus. Periods where gradually decreasing Δ14C values balance out the rate of 14C decay provide the horizontal age plateaus, as outlined in the Discussion section. Superimposed are straight line segments in green (Bard and Heaton Reference Bard and Heaton2021, called “B&H 2021”) and dark pink (modified from Sarnthein et al. Reference Sarnthein, Küssner, Grootes, Ausin, Eglinton, Muglia, Muscheler and Schlolaut2020; using the revised ages of Bronk Ramsey et al. Reference Bronk Ramsey, Heaton, Schlolaut, Staff, Bryant, Lamb, Marshall and Nakagawa2020) that display the atmospheric Δ14C structures as defined both by visual inspection of raw 14C ages and the 1st derivative technique (Figure 1; Sarnthein et al. Reference Sarnthein, Balmer, Grootes and Mudelsee2015). Based on a careful check of the position of plateau boundaries (rules of definition in Text Box 1), we slightly revised three of them previously published (Sarnthein et al. Reference Sarnthein, Küssner, Grootes, Ausin, Eglinton, Muglia, Muscheler and Schlolaut2020): (i) a slight upward shift of the base of plateau 7, (ii) omission of the boundary between plateaus 6b and 6a, and (iii) a minimal backward shift of the 5a-b plateau boundary by a single age date.

Figure 3 High-resolution record of atmospheric 14C age jumps and plateaus (i.e., a suite of labeled horizontal boxes that envelop scatter bands of largely constant 14C ages extending over >400 cal. yr; for definition of plateaus see Text Box 1) in a sediment section of Lake Suigetsu (Figure 2 of Sarnthein et al. Reference Sarnthein, Küssner, Grootes, Ausin, Eglinton, Muglia, Muscheler and Schlolaut2020) vs. tree ring-based 14C age jumps and plateaus 10–15 cal ka BP (Reimer et al. Reference Reimer, Austin, Bard, Bayliss, Blackwell, Ramsey, Butzin, Cheng, Edwards and Friedrich2020; 14.0–14.8 cal. ka BP: suppl. by data of Adolphi et al. Reference Adolphi, Muscheler, Friedrich, Güttler, Wacker, Talamo and Kromer2017). Also, 14C age jumps and most plateau boundaries are highlighted by maximums in the 1st derivative record of 14C per cal years and 1σ uncertainty range (bandwith of 150 yr for tree-ring record; 200 yr for Suigetsu record). Blue line averages paired double and triple 14C ages of Suigetsu plant macrofossils. Age control points (black, at plateau boundaries, in cal. ka) follow varve counts (Schlolaut et al. Reference Schlolaut, Staff, Marshall, Brauer, Bronk Ramsey, Lamb and Nakagawa2018) incorporated by U/Th model-based ages of Bronk Ramsey et al. (Reference Bronk Ramsey, Staff, Bryant, Brock, Kitagawa, van der Plicht, Schlolaut, Marshall, Brauer and Lamb2012, Reference Bronk Ramsey, Heaton, Schlolaut, Staff, Bryant, Lamb, Marshall and Nakagawa2020) on the 2020-time scale. YD = Younger Dryas, B/A = Bølling-Allerød (after Steffenson et al. Reference Steffensen, Andersen, Bigler, Clausen, Dahl-Jensen, Fischer, Goto-Azuma, Hansson, Johnsen and Jouzel2008). Red lines depict long-term trend of tree ring- and Suigetsu-based 14C ages 10–15 cal ka BP each. The perfectly overlapping trendlines (joint slope of 0.78 ± 0.03 and intercepts of 1009 ± 364 yr for Suigetsu vs. 992 ± 415 yr for tree ring record) are derived from linear ordinary least-squares regression and used to derive short-term trend residuals and binned correlation coefficients both for 14C records, shown in Figure 4 and Supplement Figure S1.
For the period from 27 to 30 cal ka BP, plateaus numbered 10b and 11 deviate from the Bayesian plot. Here the Suigetsu 14C data appear to have a problem since 14C ages, generated by different laboratories, diverge by up to 1000 yr at analytical 1σ uncertainties of ∼150 to below 400 yr (Bronk Ramsey et al. Reference Bronk Ramsey, Staff, Bryant, Brock, Kitagawa, van der Plicht, Schlolaut, Marshall, Brauer and Lamb2012). Our plateau definitions tried to bridge the discrepancy by weighted average values. The Bard and Heaton (Reference Bard and Heaton2021) Bayesian spline in Figure 2, apparently, treated the discrepancy differently.
Although comparison of Figure 1 and 2 indicates that it is possible to objectively and reproducibly identify a fine structure in the noisy atmospheric 14C Suigetsu record, the question remains whether this fine structure is local or has wider significance. The answer is found in Figure 3 that presents a further important line of evidence for the reasoning discussed in this study: It shows the high-resolution Suigetsu atmospheric record (Bronk Ramsey et al. Reference Bronk Ramsey, Heaton, Schlolaut, Staff, Bryant, Lamb, Marshall and Nakagawa2020) (Figure 3, right Y-axis) together with the highly detailed tree-ring-based 14C master record (Reimer et al. Reference Reimer, Austin, Bard, Bayliss, Blackwell, Ramsey, Butzin, Cheng, Edwards and Friedrich2020; Adolphi et al. Reference Adolphi, Muscheler, Friedrich, Güttler, Wacker, Talamo and Kromer2017) (Figure 3, left Y-axis). Atmospheric 14C-age jumps and plateaus between ∼10 and ∼15 cal ka BP have been defined by scatter bands of largely constant 14C ages extending over >400 yr each. Based on visual inspection most Suigetsu structures agree with the analogous, largely coeval 14C-age structures of the tree-ring-based master record of atmospheric 14C ages. The fit largely applies to the length of the various plateaus, in some cases also to internal plateau structures. This finding suggests that the clearly paired Suigetsu-based centennial-to-millennial-scale fluctuations in 14C concentration for the period 10 to ∼15 cal ka BP are indeed real and reflect a global atmospheric 14C signal.
DISCUSSION
In a next step, we tried to corroborate the authenticity of Suigetsu atmospheric 14C structures by testing our visual match of Suigetsu- and tree ring-/floating tree ring-based 14C structures from ∼10 to ∼15 cal ka BP by means of statistical methods, that is, by calculating the “binned correlation coefficients” (Mudelsee Reference Mudelsee and Cham2014: Section 7.5.1 therein) for both time series (Figures 3 and 4a, b). Indeed, the visual match is well supported by these quantitative analyses of the association between the two time least-squares regression and used to derive short-term trend residuals and binned correlation coefficients both for 14C records, shown in Figure 4 and Supplement Figure S1.

Figure 4 Binned correlation coefficient for the atmospheric 14C records of Lake Suigetsu and tree-ring-based 14C record of IntCal20 (Bronk Ramsey et al. Reference Bronk Ramsey, Heaton, Schlolaut, Staff, Bryant, Lamb, Marshall and Nakagawa2020; Reimer et al. Reference Reimer, Austin, Bard, Bayliss, Blackwell, Ramsey, Butzin, Cheng, Edwards and Friedrich2020; methods of binned correlation in Mudelsee Reference Mudelsee and Cham2014). (a) Binned correlation coefficient of Suigetsu- vs. tree-ring-based trend residuals, assuming a bin width of 100 yr. Correlation coefficient is r = 0.90 with 95% confidence interval (0.85; 0.93). (b) Trend residuals (plus zero lines) of Suigetsu- and tree ring-based 14C ages 10–15 cal ka BP (shown in Figure S1) are compared to the cal. age range of the Younger Dryas (YD), Bølling-Allerød (BA), and Heinrich-1 stadial (HS-1) (following Steffensen et al. Reference Steffensen, Andersen, Bigler, Clausen, Dahl-Jensen, Fischer, Goto-Azuma, Hansson, Johnsen and Jouzel2008), the position and extent of atmospheric 14C plateaus (sensu Sarnthein et al. Reference Sarnthein, Küssner, Grootes, Ausin, Eglinton, Muglia, Muscheler and Schlolaut2020), and the late deglacial rise in atmospheric pCO2 at 15–10 cal ka BP (West Antarctic CO2 record of Marcott et al. Reference Marcott, Bauska, Buizert, Steig, Rosen, Cuffey, Fudge, Severinghaus, Ahn and Kalk2014), showing that 14C plateaus are clearly related to a coeval rise in pCO2.
The “binned correlation coefficient” is the same as the ordinary Pearson’s correlation coefficient, however, calculated on the respective averages within time bins of the two time series. The very existence of persistence or “memory” in a time series (which is typical for climate) on a particular grid of time values allows us to infer the correlation with other time series that are observed on a different grid. From the estimated persistence times and the average temporal spacings, we determined an optimal bin width of 100 years (Mudelsee Reference Mudelsee and Cham2014: Eq. 7.48 therein). The calculated binned correlation coefficient between the Suigetsu- and the tree-ring-based values then equals 0.90 with a 95% calibrated Student’s t confidence interval of [0.86; 0.93], which means a significant correlation that is very high. Thus, the visual match is well supported by these quantitative analyses of the association between the two time series.
Thus, the paired tree ring-based master record of annual changes in atmospheric 14C concentration provides conclusive evidence that the suite of semi-millennial features of Suigetsu provides insights into a kind of authentic feature of 14C records that was intrinsic for late deglacial times, a feature with global significance.
Necessarily, the significance of the centennial to millennial structure of the Suigetsu atmospheric 14C record may be extrapolated to the preceding early deglacial and peak glacial time span 15–30 cal ka BP, when major tree ring records are widely absent for further confirmation and 14C records without much fine structure from carbonate archives have largely dominated the IntCal20 record. Accordingly, Suigetsu terrestrial macrofossils extend—without break—the actual atmospheric 14C record beyond the range of tree rings from ∼15 cal ka BP back to >∼35 cal ka BP. The latest results on 14C variability in floating tree-ring sections older than 14 cal ka BP, presented at the 24th Radiocarbon Conference in Zürich (Friedrich et al. Reference Friedrich2022), largely agree with the Suigetsu atmospheric 14C record.
From 20 to 35 cal ka BP, the global significance of the plateau and jump structures in the Suigetsu 14C record is supported by the fact that a large portion of these structures can also be seen in a Bayesian spline of analogous Δ14C-based structures in the independent deconvoluted U/Th-dated 14C record derived from Hulu Cave data (Figure 5; Bronk Ramsey et al. Reference Bronk Ramsey, Heaton, Schlolaut, Staff, Bryant, Lamb, Marshall and Nakagawa2020). The Hulu Cave record was admittedly smoothed and is generally interpreted as being subject to a ∼460 yr dead-carbon reservoir effect with limited variability. In fact, however, the Hulu speleothem may have (also) been affected by allegedly constant, but fairly unknown variations of the local “reservoir effect of soil organic carbon” in the Hulu speleothem over the time span under discussion (Southon et al. Reference Southon, Noronha, Cheng, Edwards and Wang2012; Noronha et al. Reference Noronha, Johnson, Southon, Hu, Ruan and McCabe-Glynn2015; Cheng et al. Reference Cheng, Edwards, Southon, Matsumoto, Feinberg, Sinha, Zhou, Li, Li and Xu2018; Reimer et al. Reference Reimer, Austin, Bard, Bayliss, Blackwell, Ramsey, Butzin, Cheng, Edwards and Friedrich2020). In view of the joint U/Th basis of age control for both the 14C records of Suigetsu und Hulu Cave (as presented by Bronk Ramsey et al. Reference Bronk Ramsey, Heaton, Schlolaut, Staff, Bryant, Lamb, Marshall and Nakagawa2020), various distinct centennial-scale offsets of some Hulu Cave 14C features from those of Suigetsu (such as near to 22, 24, 26, and 28.5 cal ka BP) perhaps may reflect short-lasting shifts in the soil-carbon fraction of the Hulu record, now treated as a constant dead carbon fraction of 460 yr (Southon et al. Reference Southon, Noronha, Cheng, Edwards and Wang2012).

Figure 5 A comparison of Bayesian spline compilations of datasets of Suigetsu (green) and Hulu speleothem, transformed using a MatLab deconvolution algorithm (linear ramp with mean of 420 yr) (magenta) over the period 20–35 cal. ka. Gradually decreasing atmospheric Δ14C values reflect atmospheric 14C age plateaus and their uncertainty range (black numbers in brackets). Rising Δ14C values reflect atmospheric 14C age jumps. (Courtesy of Bronk Ramsey et al. Reference Bronk Ramsey, Heaton, Schlolaut, Staff, Bryant, Lamb, Marshall and Nakagawa2020.)
Our conclusions on the authenticity of atmospheric 14C-age structures have important implications for global age correlation of marine and terrestrial sediment records records that concern (i) their dating, (ii) quantification of local differences in 14C concentration, and (iii) information on carbon cycle and climate interactions. As detailed in Sarnthein et al. (Reference Sarnthein, Küssner, Grootes, Ausin, Eglinton, Muglia, Muscheler and Schlolaut2020), both visual inspection and spikes of the 1st derivative of the Suigetsu atmospheric 14C record (Figure 1), that are sharp in marking episodes of rapid change, suggest that the cal. ages of the upper and lower plateau boundaries may serve as global age tie points for the age correlation of marine sediment records. The robustness and uncertainty of these structures are best age-calibrated by the data of Bronk Ramsey et al. (Reference Bronk Ramsey, Heaton, Schlolaut, Staff, Bryant, Lamb, Marshall and Nakagawa2020) at the marked 14C-age jumps that separate two subsequent 14C plateaus each, the range of which is marked by enveloping “boxes” in the Suigetsu record of Figures 1 and 3. Accordingly, the uncertainty of cal. age estimates of the beginning and/or end of an atmospheric 14C plateau hardly exceeds ∼50 to ∼100 years each, when employing the trends of age estimates listed by Bronk Ramsey et al. (Reference Bronk Ramsey, Heaton, Schlolaut, Staff, Bryant, Lamb, Marshall and Nakagawa2020). 14C plateau identification in marine sediment records requires a largely constant marine reservoir age (MRA) over the total length of a plateau. Otherwise, the atmospheric 14C plateaus would not be reflected as a plateau in ocean surface plankton. Also, the average 14C age of any tree-ring- and/or Suigetsu-based 14C plateau may be compared to that of any coeval plateau properly identified in ocean sediment archives. The difference in 14C average age estimated for paired, that is, for coeval atmospheric and marine plateaus will serve as robust estimate of local marine reservoir ages.
Finally, a major question concerns the potential origin of the centennial-to-millennial-scale fluctuations of atmospheric 14C concentration found at Suigetsu, the only purely atmospheric record without any assumptions on secondary effects. 14C decay reduces the original 14C-signal amplitude over time so, different from the Holocene, clear 14C signals of solar modulation with peak-to-peak values of ∼3 pMC (percentage Modern Carbon) necessarily disappear below the 2σ level of detection sensitivity in deglacial-to-glacial records. As a result, most of the 14C structure visible beyond 8–10 cal ka BP originally was connected to larger signals and, most likely, to changes in climate and the ocean-atmosphere CO2 exchange (first suggested by Stuiver and Braziunas Reference Stuiver and Braziunas1993, on the basis of residual Δ14C of the Holocene long-term trend).
The latter hypothesis receives independent support by distinct structures, that is, from two pronounced negative millennial-scale excursions in the record of 14C-age residuals at 15.2–14.4 and 13.2–11.7 cal. ka (Figure 4b top and Figure S1). They represent a negative deviation of actual 14C ages from the long-term detrended 14C-age records 10-15 cal ka BP for the tree ring- and Suigetsu-based 14C records each. The subsequent rise in 14C-age residuals parallels the very end (and subsequent interstadial) of a pronounced cold climate spell such as the Younger (YD) (12.8–11.7 cal ka BP) and the final phase of Heinrich Stadial 1 (HS1) (∼15.1–14.6 cal ka BP). A third increase in 14C-age residuals, a distinct fast rise in 14C age, follows a minor negative excursion of 14C trend residuals, that is centered near 11 cal ka BP and goes along with the Preboreal cold spell.
Near the end of cold stadials, the positive excursion of 14C-age residuals, that is, a relatively fast increase in 14C ages and reduced deviation from the long-term average in years (Figure S1) reflects per se a transient dilution of atmospheric 14C by “old” oceanic CO2 (Figure 4b bottom). In particular, this drop in 14C has paralleled a major 14C plateau and/or cluster of 14C plateaus named “YD” and “1”. Most likely, they were linked to short-term deglacial events of enhanced release of “old” CO2 from the (deep) ocean to the atmosphere reaching 12–13 ppm each (Figure 4b bottom) (Marcott et al. Reference Marcott, Bauska, Buizert, Steig, Rosen, Cuffey, Fudge, Severinghaus, Ahn and Kalk2014). The CO2 release may reflect a major change, in part a reversal in ocean Meridional Overturning Circulation (Sarnthein et al. Reference Sarnthein, Winn, Jung, Duplessy, Labeyrie, Erlenkeuser and Ganssen1994, Reference Sarnthein, Schneider and Grootes2013; Bauska et al. Reference Bauska, Marcott and Brook2021; Yu et al. Reference Yu, Oppo and Jin2022). If the causal relationships deduced for Figure 4b are extrapolated to earlier times, that is, to the early deglacial and peak glacial 14C record, they may suggest a series of events marked by a short-term ocean-driven rise in atmospheric CO2 and corresponding transient, gradually fading out reduction in atmospheric 14C unrecorded till now. Altogether, these processes may form a mechanism suitable to explain the origin of the suite of 14C plateaus characteristic of the Suigetsu 14C record (Figures 1, 2, and 5) (Yu et al. Reference Yu, Oppo and Jin2022; cf. Vettoretti et al. Reference Vettoretti, Ditlevsen, Jochum and Rasmussen2022).
In summary, the Suigetsu 14C fine structure may contain valuable information on past interactions between climate change, ocean-atmosphere CO2 exchange and the carbon cycle beyond 15 cal. ka during deglacial and peak glacial times. These centennial-to-millennial-scale signals can also be traced in high-resolution 14C records of (hemipelagic) ocean plankton sediments (Sarnthein et al. Reference Sarnthein, Küssner, Grootes, Ausin, Eglinton, Muglia, Muscheler and Schlolaut2020).
CONCLUSIONS AND IMPLICATIONS
Detailed evaluation and tests of the Suigetsu atmospheric 14C record suggest the following results:
-
The centennial-to-millennial-scale features in the noisy Suigetsu 14C record can be objectively identified. Such structures in the Suigetsu atmospheric 14C record can be identified by three different techniques, namely visual inspection, first derivative, and Bayesian spline inflections, the results of which largely agree.
-
The atmospheric 14C features of the Suigetsu record are authentic and have global significance because on the basis of “binned correlation coefficients” the fine structures of both the highly detailed global IntCal20 tree ring and the noisier Suigetsu 14C record ∼10–15 cal ka BP are closely coherent.
-
Suigetsu 14C ages extend beyond the reach of tree-ring dates ∼15 cal ka BP, without break, the record of atmospheric 14C oscillations back to >35 cal. ka BP. Between 20 and 35 cal ka BP, they are widely reproduced by structures depicted in the carbonate-based and U/Th dated 14C record of the Hulu speleothem, though the oscillations may have been smoothed.
-
Most likely the origin of semi-millennial-scale 14C fluctuations was linked to the global carbon cycle, that is, to changes in global climate, ocean meridional overturning circulation, and ocean-atmosphere CO2 exchange, since 14C decay has too strongly attenuated the potential forcing of solar modulation of 14C signals prior to the Holocene.
Once precisely age-calibrated, the suite of features and age tie points in the atmospheric Suigetsu 14C record have major implications, providing a potential tool and corner stone for the global stratigraphic correlation of analogous 14C records deduced from shallow-dwelling oceanic plankton in sediment records that follows the 14C-age signals of the atmosphere.
SUPPLEMENTARY MATERIAL
To view supplementary material for this article, please visit https://doi.org/10.1017/RDC.2023.47
ACKNOWLEDGMENTS
We are grateful to Dr. S. Beil, University of Kiel, for generous computer assistance. We particularly appreciate the highly constructive and careful suggestions of a reviewer in two successive reviews. He was exceptionally aware of a robust reasoning important for the general implications of our manuscript, and its linguistically sound wording.