Introduction
My introduction to the world of parasitology began as an undergraduate when I explored the digenean community within communities of freshwater snails. It was mind-blowing to look out over a lake and think of all the snails, parasites, and related invertebrate and vertebrate hosts in the system. In preparing this review for the centenary of The Journal of Helminthology, it has been exciting to draw together recent examples from the explosion of knowledge on ecological interactions among helminth parasites, their hosts, and their environment. The underlying conceptual framework is that each helminth is affected by and influences its biotic and abiotic environment, intrinsic factors within the host, and social interactions among hosts, and that human-induced changes to the local and global environment will affect, and may also be affected by, many of these interactions. The information compiled here makes it abundantly clear how our understanding has advanced but also how our current understanding only touches the surface.
The parasite lens
This section considers the interactions of helminth parasites with their environment from the lens of the parasite. What factors affect the ability of trematodes, tapeworms, nematodes, and acanthocepthalans to complete their life cycle? Which ones are beneficial? Which ones are of concern to the parasite? What do helminths ignore? Helminths directly or indirectly interact through predator–prey relationships, competition, and symbiotic or parasitic relationships with a diverse range of other organisms within the host, in the external environment, and even within the helminth itself, and these are explored in depth. The impact of abiotic factors on helminths focuses on temperature, water chemistry, wind, light, UV radiation, and pollutants, as well as helminth responses to chemical signals from the host. Social and intrinsic host factors are considered briefly. Social factors are very important for human helminths but for non-human helminths, the ‘social’ dimension is often embedded in how the helminth interacts with its biotic and abiotic environment or how human actions alter the environment of the parasite. Here, a few specific examples of social factors are provided. Host intrinsic factors such as physiology, immune status, reproductive status, and nutritional status often influence the outcomes of ecological influences on the parasite. Because these factors have been the subject of many reviews, only age, sex, reproductive status, and diet are considered briefly. A summary of key hypotheses emerging from studies below is presented in Figure 1.

Figure 1. The parasite lens – hypothesized pathways through which biotic, abiotic, social, and intrinsic factors may positively or negatively influence helminths, based on literature cited in this review.
Predation: Beyond the obvious
For helminths that are transmitted through the food chain (‘trophic transmission’), it is self-evident that predators that serve as the next host in the life cycle are critically important to the persistence of the helminth. When predators prey on the infected intermediate host, both host and parasite usually benefit as the host attains nutrients, and the parasite attains its next host. It has long been recognized that helminths can manipulate the morphology or behaviour of intermediate hosts, making them more susceptible to predation (de Vries & van Langevelde Reference De Vries and Van Langevelde2017). In the case of Euhaplorchis californiensis metacercariae that encyst in the brain of the California killifish, modulation of serotonin signaling has been hypothesized to make infected fish more conspicuous and at greater risk of predation by avian birds that serve as the definitive host (Helland-Riise et al. Reference Helland-Riise, Vindas, Johansen, Nadler, Weinersmith, Hechinger and Øverli2020). Using repeated experimental infection of killifish designed to mimic natural intensities, a non-linear negative association between serotonergic activity and number of metacercariae/g body mass was observed, but only above a low-intensity threshold (Helland-Riise et al. Reference Helland-Riise, Vindas, Johansen, Nadler, Weinersmith, Hechinger and Øverli2020). This is consistent with the hypothesis that a parasite-mediated shift in risk-taking is linked to neurochemical changes (serotonergic activity) in the killifish brain.
In the case of Dicrocoelium dendriticum metacercariae in ants (Formica aserva), the mechanism underlying increased susceptibility of infected ants to predation is controlled by a single metacercariae in the ant brain. When ants ingest cercariae within a slime ball released by a terrestrial snail host, the majority of cercariae migrate to the ant abdomen, but one migrates to the ant brain. Using DNA-based pedigree analysis, Criscione et al. (Reference Criscione, van Paridon, Gilleard and Goater2020) demonstrated a clonal relationship between the single metacercaria in the ant brain and the many metacercariae in the ant abdomen. They found that the thick-walled metacercariae in the abdomen are infective to ungulates and lagomorphs, whereas the single thin-walled metacercaria in the brain is unable to establish in the definitive host. Thus the ‘choice’ of one cercaria to go to the brain enhances transmission of its clonemates, but not itself.
Predation that occurs before the parasite is infective is disadvantageous to the parasite, as shown with plerocercoids of the tapeworm Ligula intestinalis transmitted from cyprinid fish to piscivorous aquatic birds (Gabagambi et al. Reference Gabagambi, Salvanes, Midtøy and Skorping2019). A simulated predation test of Lake Malawi sardines compared uninfected sardines with sardines infected with an average of either one pre-infective Ligula plerocercoid or one infective plerocercoid. Fish infected with infective plerocercoids were more active and were caught more quickly than fish with pre-infective plerocercoids, independent of the size of the plerocercoid or the fish. Gabagambi et al. (Reference Gabagambi, Salvanes, Midtøy and Skorping2019) suggested that the pre-infective larvae may suppress those behavioural alterations that make fish more susceptible to predation during the infective phase.
Non-host predators have the potential to reduce both parasite and host abundance and are sometimes considered biological control agents. The riverine prawn (Macrobrachium spp.) feeds on snail hosts of human schistosomiasis. Sokolow et al. (Reference Sokolow, Lafferty and Kuris2014) demonstrated that prawns can control the population growth of both Biomphalaria and Bulinus snails. Despite a positive impact of small prawns on the number of snail egg masses, predation on juvenile snails dramatically slowed snail population growth (Sokolow et al. Reference Sokolow, Lafferty and Kuris2014). Furthermore, prawns preferentially preyed on schistosome-infected snails compared with uninfected snails (Swartz et al. Reference Swartz, De Leo, Wood and Sokolow2015), perhaps due to reduced anti-predator behaviors in the schistosome-infected snails.
Predation is also relevant to free-living stages of helminths that can be an important component of the diet of non-host consumers. Koprivnikar et al. (Reference Koprivnikar, Thieltges and Johnson2023) recently reviewed the range of factors that influence this ‘loss’ to the parasite population and the implications for both food webs and biocontrol of helminths. The functional response of three-spined sticklebacks has been shown to respond to cercarial density, size, behaviour, and emergence time (Born-Torrijos et al. Reference Born-Torrijos, Paterson, van Beest, Vyhídalová, Henriksen, Knudsen, Kristoffersen, Amundsen and Soldánová2021). Stickleback feeding initially increased linearly with the density of the small, less dense, less active, and nocturnally released Plagiorchis cercariae, whereas the stickleback functional response to low densities of the larger, more dense, and more active Trichobilharzia cercariae released during the daytime was initially exponential (Type III functional response).
Intraspecific helminth competition
Evidence that an individual helminth responds to the number of conspecifics within the host has been known for decades. Some of the earliest work, done in tapeworms, focused on the ‘crowding effect’ observed when adult Hymenolepis diminuta from more heavily infected hosts had smaller mass (Roberts Reference Roberts1961). The expectation that the individual parasite will ‘suffer’ at higher intensities of infection due to competition for resources has been shown, for example, in isopods where glycogen stores within the larval acanthocephalan Acanthocephalus dirus were negatively correlated with larval intensity, indicating intraspecific competition for this energy store (Caddigan et al. Reference Caddigan, Pfenning and Sparkes2017). Sibship reconstruction that inferred parental genotypes from miracidial microsatellites has provided clear evidence that fecundity of Schistosoma haematobium in humans declines with increasing worm burden (Neves et al. Reference Neves, Gower, Webster and Walker2021). As described below, the scope of intensity-dependent relationships now extends to helminth mating strategies, host selection, and positive density dependence as observed through growth facilitation. Furthermore, there is now evidence of inequality in an individual helminth’s response to high-intensity infection.
Tapeworm intensity has been shown to alter mating strategies but not sperm competition in tapeworms. Using gravid Oochoristica javaenis collected from the Mediterranean gecko, Detwiler et al. (Reference Detwiler, Caballero and Criscione2017) examined the impact of intensity of infection on self-fertilization versus outcrossing in these hermaphroditic tapeworms. Genetic analysis of each worm was done using a non-gravid proglottid. Then gravid proglottids were fed to flour beetles, and DNA from the resulting metacestodes was analysed. The frequency of self-fertilization was higher than expected by chance and the rate of self-fertilization declined with higher infection intensities (Detwiler et al. Reference Detwiler, Caballero and Criscione2017). Andreou and Benesh (Reference Andreou and Benesh2014) placed individual Schistocephalus solidus plerocercoids in a simulated in vitro bird gut for 36 hours until they had mature spermatozoa and then allowed the adults to reciprocally fertilize for 4 hours, first with a worm obtained from a fish that had only one plerocercoid and then with a worm from a multiple infection, or vice versa. Based on the genotype of resulting eggs, there was no evidence of sperm competition because neither plerocercoid numbers in the stickleback nor sequence of exposure to worms from single or multiply infected sticklebacks altered paternity.
Infection intensity has been shown to be a determinant of whether additional parasites join the helminth population within the host and their site preference. Mermithid nematodes that infect Culex pipiens pipiens larvae avoid infected hosts (Sanad et al. Reference Sanad, Sun, Shamseldean, Wang and Gaugler2017). Both Romanomermis iyengari and Strelkovimermis spiculatis detect not only whether the host is infected but also the intensity of infection. They prefer to infect uninfected mosquito larvae, and the time required for pre-parasites to infect the larval mosquito increases with intensity of infection (Sanad et al. Reference Sanad, Sun, Shamseldean, Wang and Gaugler2017). Leung et al. (Reference Leung, Keeney and Poulin2010) explored factors influencing the site location of Curtuteria australis metacercariae in the cockle, Austrovenus stutchburyi. Incoming cercariae were more likely to move to the tip of the foot if other metacercariae were already located in this site, perhaps because cercariae detect scars around the foot and use this to choose a penetration site. This strategy seems to benefit transmission as incoming metacercariae cooperate with the metacercariae already in the tip of the foot to promote predation by shore bird definitive hosts (Leung et al. Reference Leung, Keeney and Poulin2010) – an example that contrasts the earlier sample of Dicrocoelium metacercariae in ants.
Individual helminths can benefit from being in a heavily infected host. The relationship of parasite density with body size and egg production was explored in two species of monogeneans of the bluegill sunfish in New Jersey, USA (Bromagen Reference Bromagen2022). Gills of virtually all sunfish were infected with Onchocleidus sp 1 and Actinocleidus sp. Body size of both species was positively – not negatively – correlated both with infrapopulation density and with the combined density of both species (infracommunity density), but fecundity was unaffected. Because the host response to both monogeneans involves mucus production and because mucus is a food resource for the parasites, high amounts of mucus may on balance benefit growth of both species of monogeneans (Bromagen Reference Bromagen2022). As with these monogeneans, growth of Diplostomum pseudospatheceum metacercariae was enhanced when rainbow trout were experimentally infected with higher numbers of cercariae, perhaps because metacercariae perforate the eyeball as they move into the eye, increasing available food resources (Mironova et al. Reference Mironova, Gopko, Pasternak, Mikheev and Taskinen2022). These examples of positive density dependence appear to be rare cases of facilitation of growth at higher intensities of infection.
The well-documented aggregation of helminths within a host population means that those parasites within the relatively few heavily infected hosts are most likely to experience density-dependent reductions in survival or fecundity. It is often assumed that the average response of the infrapopulation to infection intensity is representative of each individual’s response. However, even though average fecundity of the adult acanthocephalan Corynosoma cetaceum in fransciscan dolphins was unaffected by parasite density, a high degree of inequality in fecundity among gravid females was observed at high-intensity infections (Aznar et al. Reference Aznar, Hernández-Orts, Vélez-Rubio, Fernández, Muriel and Raga2018).
Interspecific competition among helminths
In snails, there is considerable evidence that rediae-producing digeneans dominate over those that produce sporocysts. Like social insects, rediae of some digeneans have two morphologically distinct castes, larger rediae with small mouthparts that are responsible for producing cercariae (a ‘reproductive caste’), and very small rediae with large mouthparts that attach to and ingest sporocysts of competing digenean species (a ‘soldier caste’) (Garcia-Vedrenne et al. Reference Garcia-Vedrenne, Quintana, DeRogatis, Martyn, Kuris and Hechinger2016; Poulin et al. Reference Poulin, Kamiya and Lagrue2019). Long-term lab experiments using Zeacumantus subcarinatus snails demonstrated the dominance of the redia-producing Philophthalmus sp. over the sporocyst-producing Maritrema novaezealandense (Lagrue et al. Reference Lagrue, MacLeod, Keller and Poulin2018). When snails infected with Philophthalmus were exposed biweekly to different concentrations of gull feces, Maritrema establishment was higher in Philophthalmus-infected snails than uninfected snails, but co-infection resulted in fewer Maritrema sporocysts and fewer ‘reproductive’ Philophthalmus rediae, and the temporal increase in cercarial production by both species over a year was dampened. Furthermore, emerging evidence indicates that the soldier caste of Philophthalmus rediae limits bacterial infection (MacLeod et al. Reference MacLeod, Poulin and Lagrue2018) and that the reproductive caste can assume the function of soldiers, but not the other way around (Poulin et al. Reference Poulin, Kamiya and Lagrue2019), indicating asymmetric plasticity in the two castes.
Two additional competing digenean pairs have been studied extensively. Co-infection of periwinkles with Himasthla elongata rediae and Renicola roscovita sporocysts resulted in smaller cercariae of both species, compared with single species infections (Mouritsen & Elkaer Reference Mouritsen and Elkjaer2020), but production and longevity of Renicola cercariae were lower in Himasthla-infected snails (Mouritsen & Andersen Reference Mouritsen and Andersen2017). As with Philophthalmus–Maritrema competition, a key determinant of the asymmetrical competition between Himasthla and Renicola was the higher proportion of the Himasthla soldier caste during co-infections with Renicola (Mouritsen & Andersen Reference Mouritsen and Andersen2017). When Biomphalaria glabrata snails were simultaneously infected with Echinostoma caproni and Schistosoma mansoni, echinostome rediae ingested sporocysts, reducing schistosome cercarial production (Sandland et al. Reference Sandland, Rodgers and Minchella2007) and thus making the echinostome the dominant competitor. However, when snails were first infected with schistosomes, even though echinostome development was delayed, echinostomes remained the dominant competitor as echinostome cercarial release was always higher in co-infected snails (Carpenter et al. Reference Carpenter, Vannatta and Minchella2021).
At least one example indicates that rediae are not always dominant over sporocysts. Microphallus simillimus sporocysts contain 2 to 5 metacercariae (Alda & Martorelli Reference Alda and Martorelli2014) and thus fill the snail digestive gland. This was used to explain the very high prevalence of Microphallus relative to other digeneans that produce rediae in a field survey of the intertidal snail Heleobia australis (Alda et al. Reference Alda, Bonel, Cazzaniga, Martorelli and and Lafferty2019).
In the second intermediate host, sequence of co-infection shapes interspecific competition. Prior infection of the Pacific chorus frog with metacercariae of Echinostoma trivolvis that live in the frog kidney negatively affected infection success of Ribeiroia ondatrae, whose metacercariae are found around the limb buds. The impact on this interspecific competition on Ribeiroia was more than 80% stronger than the impact of intraspecific Ribeiroia competition (Hoverman et al. Reference Hoverman, Hoye and Johnson2013).
Evidence for interspecific helminth competition in mammals is largely inferred from surveys and experimental field studies. A monthly survey of European rabbits in Scotland over 8 years revealed distinct relationships between infection intensity and fecundity for two competing intestinal nematodes, Trichostrongylus retortaeformis and Graphiodium strigosum (Cattadori et al. Reference Cattadori, Wagner, Wodzinski, Pathak, Poole and Boag2014). Co-infected rabbits had fewer T. retortaeformis and more G. strigosum than rabbits infected with only one nematode. Furthermore, in utero T. retortaeformis fecundity corrected for length increased with worm intensity only in co-infected hosts, whereas corrected in utero G. strigosum fecundity decreased with increasing intensity in both single and mixed infections. When sheep were experimentally infected with either Haemonchus placei or H. contortus and then grazed together on a worm-free pasture, H. placei transmission had been eliminated after 3 months, and over the following 9 months, only H. contortus was present, indicating its dominance (Santos et al. Reference Santos, Amarante and Amarante2020). In Wales, Jones et al. (Reference Jones, Brophy, Mitchell and Williams2017) found a negative correlation between egg output of Fasciola hepatica and the less pathogenic digenean Calicophoron daubneyi in cattle and reported that the odds of Calicophoron infection were higher both in habitats suitable for the shared intermediate host Galba truncatula and when anthelmintic treatment was applied. Because Calicophoron outcompetes Fasciola in the snail, is less affected by anthelmintics and is less pathogenic, the presence of this emerging infection may be beneficial for the health of cattle. However, deworming cattle may favour Calicophoron in the snail, increasing its transmission back to cattle. Also, streams suitable for the snail provide a conduit by which snails and their parasites disperse through the landscape, potentially spreading infection and drug resistance. This example highlights some of the implications of helminth competition at the landscape level.
A final example considers competition between strains of the same species. By tracking infectivity of cercariae during single and mixed infections with two strains of S. mansoni (PR and NMRI) in mice, Gleichsner et al. (Reference Gleichsner, Reinhart and Minchella2018a) showed that the infectivity of the PR strain was lower during mixed exposures than with the single strain. This finding was particularly intriguing as the opposite relationship was shown in the snail intermediate host where the PR strain was the stronger competitor (Gleichsner et al. Reference Gleichsner, Reinhart and Minchella2018b).
Interspecific digenean–tapeworm interactions were explored in a field experiment using three-spined sticklebacks experimentally infected with one S. solidus plerocercoid (either a high- or low-growth form) and then caged in a lake for 3 months where the fish acquired other infections (Benesh & Kalbe Reference Benesh and Kalbe2016). More Diplostomum pseudospathaceum sensu lato but fewer Apatemon cobitis metaceracariae were found in the eyes of sticklebacks infected with the high-growth form of S. solidus when compared both with uninfected fish and with fish infected with the low-growth form. Plerocercoid-infected fish had more Proteocephalus filicollis tapeworms but fewer adult Sphaerostomum globiporum digeneans than uninfected control fish, independent of the S. solidus growth form (Benesh & Kalbe Reference Benesh and Kalbe2016).
Considering the enormous diversity of helminth co-infections, the nature and relative impact of interspecific associations within and among helminth taxa have only begun to be explored.
Helminth–microbe interactions within the host
Given the explosion of information about the microbiome, several recent reviews have explored interactions between the host microbiome and helminth parasites (see Herczeg et al. Reference Herczeg, Ujszegi, Kásler, Holly and Hettyey2021; Lawson et al. Reference Lawson, Roberts and Grencis2021; Castañeda et al. Reference Castañeda, Paniz-Mondolfi and and Ramírez2022; Salloum et al. Reference Salloum, Jorge, Dheilly and Poulin2023). As with any emerging field, much of the evidence is based on associations such as those of intestinal nematodes with altered relative abundance, composition, and diversity of the host microbiome. For example, samples collected from live European shag, a Scottish seabird, revealed that both presence and intensity of the anisakid nematode Contracaecum rudolphis were associated with increased diversity of the core and total cloacal and fecal microbiomes, but this proventricular infection was associated with only a modest difference in the microbiome of the proventriculus, perhaps because of the localized anti-inflammatory response induced by the nematode (Newbold et al. Reference Newbold, Burthe, Oliver, Gweon, Barnes, Daunt and van der Gast2017). Interestingly, more heavily infected shags had a higher abundance of Cetobacterium somerae phylotypes that are common in the gut microbiome of fish that serve as both prey for the shag and intermediate hosts for Contracaecum.
When the microbiome of Cuban tree frog tadpoles was experimentally simplified, Knutie et al. (Reference Knutie, Wilkinson, Kohl and Rohr2017) demonstrated increased susceptibility of adult frogs to the nematode Aplectana hamatospicula. In experimental mouse models, the higher caecal abundance of Firmicutes and other genera of bacteria, but lower abundance of Parabacteroides and Prevotella in response to Trichuris muris infection (see Lawson et al. Reference Lawson, Roberts and Grencis2021) were shown to limit T. muris egg hatching in the mouse gut, thus promoting a chronic T. muris infection by preventing reinfection, to the benefit of the parasite (White et al. Reference White, Houlden, Bancroft, Hayes, Goldrick, Grencis and Roberts2018). Expansion of Salmonella in the lumen of the mouse duodenum was limited following anthelmintic treatment of Heligmosomoides polygyrus (also referred to as H. p. bakeri and H. bakeri), indicating that the presence of this intestinal nematode promotes expansion of Salmonella (Brosschot et al. Reference Brosschot, Lawrence, Moeller, Kennedy, FitzPatrick, Gauthier, Shin, Gatti, Conway and Reynolds2021). The impact of nematode infection on the host microbiome has also been shown to cross host generations. Although repeated infection of pregnant and lactating mice with H. bakeri had no impact on the vaginal or milk microbiome of the dam, maternal infection increased alpha diversity and differential abundance of Lactobacillus in the stomach microbiome of the uninfected 7-day-old offspring, compared with pups of uninfected dams (Haque et al. Reference Haque, Koski and Scott2021).
Helminth–microbiome interactions have been shown experimentally to be influenced by host strain, by host sex and genotype, and by infection protocol. Infection with H. polygyrus increased the duodenal abundance of lactobacilli in a susceptible but not resistant strain of mouse, and administration of Lactobacillus taiwanensis to the resistant strain increased the regulatory T cell population making this mouse strain more susceptible to H. polygyrus (Reynolds et al. Reference Reynolds, Smith, Filbey, Harcus, Hewitson, Redpath, Valdez, Yebra, Finlay and Maizels2014). Prolonged antibiotic treatment of mice infected with H. polygyrus had no impact on parasite numbers or fecundity during a primary infection, but following a challenge infection, antibiotic-treated mice had more worms but lower fecundity (Elizalde-Velázquez et al. Reference Elizalde-Velázquez, Yordanova, Liublin, Adjah, Leben, Rausch, Niesner and Hartmann2022). Relative to control uninfected pigs, the microbial diversity of Ascaris suum-infected pigs declined gradually following a single infection, whereas microbial diversity was less stable over time in response to trickle infection (Springer et al. Reference Springer, Wagner, Koehler, Klinger, Breves, Brüggemann and Strube2022). In a fish–tapeworm system, Ling et al. (Reference Ling, Steinel, Weber, Ma, Smith, Correa, Zhu, Bolnick and Wang2020) experimentally infected F2 and backcrossed male and female three-spine stickleback with S. solidus-infected copepods and examined the impact of plerocercoids in the body cavity on the stickleback gut microbiome. They found statistical interactions between host sex and autosomal genotype in microbe Orders. For example, the relative abundance of Clostridiales was lower in infected compared with uninfected males but unaffected in females, and Caulobacterales had higher relative abundance in the F2 hybrids than in both backcrosses.
Many of the helminth-induced shifts in the microbiome may be due to bactericidal molecules released by helminths. Ascaris suum produces excretory/secretory products with antimicrobial activity (Midha et al. Reference Midha, Janek, Niewienda, Henklein, Guenther, Serra, Schlosser, Hengge and Hartmann2018), and H. bakeri antimicrobial gene expression only occurs in the presence of bacteria (Rausch et al. Reference Rausch, Midha, Kuhring, Affinass, Radonic, Kühl, Bleich, Renard and Hartmann2018). Such antimicrobials limit growth of pathogenic strains of Escherichia coli, Staphylococcus aureus, Salmonella enterica, and Enterococcus faecium, at least in vitro (Rausch et al. Reference Rausch, Midha, Kuhring, Affinass, Radonic, Kühl, Bleich, Renard and Hartmann2018), and induce a Th2 cytokine response that upregulates expression of a proline-rich protein with antibiotic properties, thus limiting tissue invasion by bacteria (Hu et al. Reference Hu, Zhang, Sifuentes-Dominguez, Zarek, Propheter, Kuang, Wang, Pendse, Ruhn, Hassell, Behrendt, Zhang, Raj, Harris-Tryon, Reese and Hooper2021). When schistosome cercariae penetrate the skin, they release antimicrobials or endotoxins that attract neutrophils and promote bacterial killing, thus limiting secondary bacterial infections at the site of penetration (Ashour & Othman Reference Ashour and Othman2020) to the benefit of both the parasite and the host. Similarly, Fasciola hepatica cercariae secrete cysteine pepsidases and other molecules during penetration across the intestinal wall and release molecules that limit movement of Escherichia coli across the intestinal barrier and dampen pro-inflammatory responses (Ashour & Othman Reference Ashour and Othman2020).
It is important to note that helminths also co-occur with viral and fungal infections and that combination, sequence, and timing intervals impact the outcome of these microbe–helminth co-infections. In fact, the outcome of helminth–virus co-infection may be mediated through helminth-induced shifts in the host microbiome which, in turn, affect viremia (Desai et al. Reference Desai, Diamond and Thackray2021a). Post-metamorphic Cuban tree frogs are naturally infected with the highly pathogenic FV3 ranavirus and the highly pathogenic chytrid fungus Batrachochytrium dendrobatidis, as well the nematode Aplectana hamatospicula. In a comprehensive experimental study, Ramsay and Rohr (Reference Ramsay and Rohr2021) found that prior fungal infection, but not viral infection, increased nematode load regardless of interval between infections. Prior infection with either the fungus or the nematode increased viral loads above that of a single infection, also regardless of the interval between exposures. However, fungal load was affected by the timing between infections. The interval between nematode and fungal infection was negatively correlated with fungal load, whereas the interval between viral and fungal infection was positively correlated with fungal load (Ramsay & Rohr Reference Ramsay and Rohr2021).
Helminths serve as hosts to microparasites
Bacteria have been found in the tegument and gastrodermis of digeneans (LoVerde et al. Reference LoVerde, Amento and Higashi1980; Gobert et al. Reference Gobert, McManus, McMullan, Creevey, Carson, Jones, Nawaratna, Weerakoon and You2022) and tapeworms (see Morley Reference Morley2016). For example, Salmonella typhimurium replicate within the S. mansoni tegument where they are protected from antibiotics. This may account for cases of septicemia following praziquantel treatment of schistosomiasis (LoVerde et al. Reference LoVerde, Amento and Higashi1980; Barnhilll et al. Reference Barnhill, Novozhilova, Day and Carlson2011). Bacterial diversity and abundance within S. japonicum differ from that of the blood of the infected host (Gobert et al. Reference Gobert, McManus, McMullan, Creevey, Carson, Jones, Nawaratna, Weerakoon and You2022). A mutually beneficial association seems to exist in that the higher abundance of facultative anaerobes such as Proteobacteria in schistosomes may be associated with the oxygen available in the microenvironment of the worms, and S. japonicum may benefit from a higher abundance of Escherichia coli that reduces adherence of immune molecules to the tegument (Gobert et al. Reference Gobert, McManus, McMullan, Creevey, Carson, Jones, Nawaratna, Weerakoon and You2022). Adult Fasciola hepatica in sheep have a novel Neorickettsia bacteria that is found in reproductive tissues and in mature eggs within the host liver, raising the possibility that this bacterium may be vertically transmitted within Fasciola (McNulty et al. Reference McNulty, Torto, Rinaldi, Fischer, Rosa, Smircich, Fontenia, Choi, Tyagi, Hallsworth-Pepin, Mann, Kammili, Latham, Dell’Oca, Dominguez, Carmona, Fischer, Brindley and Mitreva2017). In tapeworms, the abundance and density of bacteria in the tegument depend on the life history of freshwater fish hosts, season, and location within the host intestine (Morley Reference Morley2016). It was recently shown that the intestinal microbiome of Ascaris lumbricoides has a predominance of Firmicutes and Proteobacteria Phyla and is enriched with Streptococcus in heavily infected hosts, but with Lactococcus in lightly infected hosts (Klomkliew et al. Reference Klomkliew, Sawaswong, Chanchaem, Nimsamer, Adisakwattana, Phuphisut, Tipthara, Tarning, Payungporn and Reamtong2022). These intensity-driven differences were also reflected in the metabolite profile of the Ascaris intestine.
Bacteria within helminths have been shown to affect the behaviour and life history traits of a variety of nematodes (Salloum et al. Reference Salloum, Jorge, Dheilly and Poulin2023). Salmonella typhinurium infection of the chicken nematode Ascaridia galli reduced nematode egg production, and high dose infection prolonged Salmonella secretion from the infected nematodes (Foka et al. Reference Foka, Yamssi, Enyetornye, Anangmo, Mbida and Mayaka2021). The distinctive intestinal microbiome of T. muris adults (White et al. Reference White, Houlden, Bancroft, Hayes, Goldrick, Grencis and Roberts2018) may promote nematode fitness as egg hatching within the host gut was higher in the presence of Escherichia coli and other facultative anaerobes (Hayes et al. Reference Hayes, Bancroft, Goldrick, Portsmouth, Roberts and Grencis2010). This may at least partly explain differences in T. muris egg hatching and larval development in an intriguing outdoor experiment conducted by Leung et al. (Reference Leung, Budischak, The, Hansen, Bowcutt, Neill, Shellman, Loke and Graham2018). These authors compared infections in a naturally resistant strain of mouse that was either maintained in the lab (‘Lab’), maintained in an outdoor environment (‘Long-term Wild’), or infected in the lab until eggs had hatched and molted to the L2 stage and then transferred to the outdoor environment (‘Short-term Wild’). In vivo egg hatching and larval development were higher in both the Short-term Wild and Long-term Wild mice compared with the Lab mice, and the difference was associated both with shifts in the bacterial and fungal composition of the host gut community and with a dampening of the host immune-protective Th2 environment (Leung et al. Reference Leung, Budischak, The, Hansen, Bowcutt, Neill, Shellman, Loke and Graham2018).
Host–nematode–bacterial associations have been particularly well-studied in two nematode systems. One involves the symbiotic bacterium Xenorhabdus bovienii and entomopathogenic nematodes such as Steinernema affine that parasitize larval insects (Heryanto & Eleftherianos Reference Heryanto and Eleftherianos2020). Reproduction of the nematodes and release of their larvae into the environment are dependent on the slow decay of the caterpillar cadaver. This is promoted by symbiotic bacteria that are released by the nematode into the insect haemocoel at the time of nematode infection and that benefit the nematode through bioconversion of required nutrients. Once the cadaver is depleted of all its nutrients, the nematode ingests the bacteria, leaves the cadaver, and retains the bacteria until the infective nematode juveniles find an insect host. This mutualism is, at least in part, driven by bacterial metabolites that delay decay of the insect cadaver by protecting it from fungal and bacterial saprophytes, thus providing sufficient time for the nematode and bacteria to complete their life cycles. Together, the nematode–bacteria complex is highly pathogenic to the larval insect.
The second example involves Wolbachia pipientis, an obligate intracellular, gram-negative rickettsial symbiont of most filarial nematodes that is transmitted transovarially through germline cells of the adult females (Kaur et al. Reference Kaur, Shropshire, Cross, Leigh, Mansueto, Stewart, Bordenstein and Bordenstein2021; Landaman Reference Landaman2019) and that is also found in the hypodermis just below the cuticle of larval and adult filaria (Landaman Reference Landaman2019). Wolbachia relies on filariae for amino acids and other vitamins and co-factors (Foster et al. Reference Foster, Ganatra, Kamal, Ware, Makarova, Ivanova, Bhattacharyya, Kapatral, Kumar, Posfai, Vincze, Ingram, Moran, Lapidus, Omelchenko, Kyrpides, Ghedin, Wang, Goltsman, Joukov, Ostrovskaya, Tsukerman, Mazur, Comb, Koonin and Slatko2005), and filariae benefit from Wolbachia’s biosynthetic pathway for riboflavin and heme (Foster et al. Reference Foster, Ganatra, Kamal, Ware, Makarova, Ivanova, Bhattacharyya, Kapatral, Kumar, Posfai, Vincze, Ingram, Moran, Lapidus, Omelchenko, Kyrpides, Ghedin, Wang, Goltsman, Joukov, Ostrovskaya, Tsukerman, Mazur, Comb, Koonin and Slatko2005), from release of effectors that sabotage host signaling pathways to stimulate embryogenesis (Foray et al. Reference Foray, Pérez-Jiménez, Fattouh and Landmann2018), and from chitinases required for exsheathment of microfilaria prior to crossing the mosquito midgut (Quek et al. Reference Quek, Cook, Wu, Marriott, Steven, Johnston, Ford, Archer, Hemingway, Ward, Wagstaff, Turner and Taylor2022). Wolbachia also recruits neutrophils that limit eosinophil infiltration and degranulation around Onchocerca microfilaria, reducing pathology in the eye (Hansen et al. Reference Hansen, Trees, Bah, Hetzel, Martin, Bain, Tanya and Makepeace2011; Tamarozzi et al. Reference Tamarozzi, Turner, Pionnier, Midgley, Guimaraes, Johnston, Edwards and Taylor2016). The reliance of filaria on Wolbachia for egg production and adult survival has led to use of antibiotics that kill Wolbachia as a means of controlling filarial infections (Hoerauf et al. Reference Hoerauf, Specht, Marfo-Debrekyei, Büttner, Debrah, Mand, Batsa, Brattig, Konadu, Bandi, Fimmers, Adjei and Büttner2009). However, at high concentrations, antibiotics can reduce filarial worm motility and survival even though they do not limit Wolbachia. Bulman et al. (Reference Bulman, Chappell, Gunderson, Vogel, Beerntsen, Slatko, Sullivan and Sakanari2021) found that low concentrations of a range of antibiotics effectively reduced Wolbachia titers in adult female Brugia pahangi, effectively controlling the nematode. However, at higher concentrations, Wolbachia titers were unaffected by the antibiotic, perhaps because high concentrations of antibiotic have a negative effect on filarial cells that may, in turn, stimulate Wolbachia either to proliferate or transition to a ‘persister’ state that is unaffected by antibiotics (Bulman et al. Reference Bulman, Chappell, Gunderson, Vogel, Beerntsen, Slatko, Sullivan and Sakanari2021).
The complexity of the symbiont–nematode interaction is further demonstrated by interspecific competition between nematodes mediated by ‘inhibiting’ versus ‘non-inhibiting’ strains of symbiotic Xenorhabdus bacteria. Using the eastern tent caterpillar as host of the nematode Steinernema affine, Meli and Bashey (Reference Meli and Bashey2018) showed that ‘inhibiting’ strains of Xenorhabdus outcompete ‘non-inhibiting’ strains by releasing bacteriocins but that this benefit comes at a cost. Nematodes carrying an ‘inhibiting’ strain of symbiont were less successful at killing their caterpillar host and released fewer juvenile nematodes into the soil (Meli & Bashey Reference Meli and Bashey2018). Thus, although production of bacteriocins allowed the ‘inhibiting’ strain to outcompete the ‘non-inhibiting’ strain, it was a disadvantage to both the symbiont and the nematode. Furthermore, there is evidence using caterpillars of the great wax moth that the natural inhibiting strain of bacteria in Steinernema affine is directly pathogenic to the competing conspecific S. feltiae that carries a non-inhibiting strain of bacteria (Murfin et al. Reference Murfin, Ginete, Bashey and Goodrich-Blair2019). This pathogen-mediated interaction eliminated S. feltiae in in vivo competition assays (Murfin et al. Reference Murfin, Ginete, Bashey and Goodrich-Blair2019).
In addition to hosting bacteria, helminths are hosts to viruses and simple eukaryotes. A recent survey of soil nematodes identified over 150 viruses within the nematode virome (Vieira et al. Reference Vieira, Subbotin, Alkharouf, Eisenback and Nemchinov2022), and two negative-sense RNA viruses identified from Capillaria hepatica were localized at the anterior end of adult worms and in the nematode eggs found in the liver of mice and rats in New York City (Williams et al. Reference Williams, Che, Oleynik, Garcia, Muller, Zabka, Firth, Corrigan, Briese, Jain and Lipkin2019). Species of the cercozoan eukaryote Urosporidium parasitize larval digeneans in marine molluscs and crustaceans (Cho et al. Reference Cho, Kang, Le, Kwon, Jang and Choi2020). Also, microsporidia, classified as a sister group to true fungi and common invertebrate and vertebrate pathogens, are also hyperparasites of helminths. For example, a few Nosema species infect larval stages of digeneans (Toguebaye et al. Reference Toguebaye, Quilichini, Diagne and Marchand2014), including Schistosoma within the snail host (Le Clec’h et al. Reference Le Clech’h, Nordmeyer, Anderson and Chevalier2022), and all the known species of Unikaryon infect digeneans and cestodes (Sokolova et al. Reference Sokolova, Overstreet, Heard and Isakova2021). Metacercariae of Posthodiplostomum minimum from bluegill sunfish were infected with Ovipleistophora diplostomuri, a hyperparasite localized in the outer cyst wall that kills the metacercariae (Lovy & Friend Reference Lovy and Friend2017). This raises the possibility that, in some instances, hyperparasites could be considered as a form of biological control of their parasite host.
Context dependence of helminth and helminth-infected host responses to temperature
There is considerable evidence that temperature influences survival and transmission of free-living stages of helminth parasites and indirectly affects parasitic stages within poikilotherm hosts through physiological, behavioural, and immunological mechanisms (Marcogliese Reference Marcogliese2023). Recognizing that temperature under field conditions is not constant, the impact of a shift in temperature was recently investigated in Dactylogyrus vasator, a monogenean of common carp and goldfish. Egg hatching success at 20°C was negatively associated with the duration that eggs had been maintained at 5°C. However, low temperature did not affect hatching success if eggs were first exposed to 20°C for 12 or 24 hours, indicating that embryonation within a day protected the developing oncomiracidium from impact of subsequent cold temperature (Zhang et al. Reference Zhang, Shang, Cheng, Wang, Stojanovski and Li2022).
Optimal temperatures often differ among life history traits. For example, egg hatching, sexual maturation, and life cycle completion of Neobenedenia sp., a monogenean of the Almaco jack fish, were highest at 30°C, whereas optimal survival of oncomiriacidia occurred at 24°C and infection success was highest at 20° (Valles-Vega et al. Reference Valles-Vega, Ascencio, Sicard-González, Angulo, Fajer-Avila, Inohuye-Rivera and Pérez-Urbiola2019). In digeneans, Trichobilharzria szidati-infected snails, Lymnaea stagnalis, released more cercariae per day at 26°C compared with 19°C, but more cercariae were released over the lifespan of the infected snail at 19°C (Żbikowska & Marszewska Reference Żbikowska and Marszewska2018). A study exploring a wider range of temperatures found that daily release of T. szidati cercariae was almost 15 times higher at 27°C compared with 7°C but that cercarial lifespan decreased linearly between 5° and 30°C (Al-Jubury et al. Reference Al-Jubury, Kania, Bygumm and Buchmann2020). The maximum distance travelled by S. mansoni miradia and cercariae increased with temperature, although lifespan decreased (Nguyen et al. Reference Nguyen, Gemmel and Rohr2020).
Distinct temperature-dependent patterns of release, activity, and survival were identified among three genera of cercariae in simulated subarctic lake conditions (Born-Torrijos et al. Reference Born-Torrijos, van Beest, Vyhlídalová, Knudsen, Kristoffersen, Amundsen, Thieltges and Soldánová2022). Mortality of Diplostomum spp., Apatemon spp., and both a large and small morphotype of Plagiorchis spp. was higher at 13° than 6°C. However, Apatemon and large-sized Plagiorchis cercariae were more active at 13° than 6°C throughout the experiment, whereas Diplostomum were initially more active at 6°C and small-sized Plagiorchis were initially more active at 13°C (Born-Torrijos et al. Reference Born-Torrijos, van Beest, Vyhlídalová, Knudsen, Kristoffersen, Amundsen, Thieltges and Soldánová2022). Broadly similar patterns were observed by Selbach and Poulin (Reference Selbach and Poulin2020), who compared cercarial productivity, movement, and survival at 16° versus 20°C for Coitocaecum parvum, Maritrema poulini, Apatemon sp., and Aporocotylid sp. I that infect the New Zealand mudsnail. As with the subarctic system, mortality of all parasites was higher at the higher temperature (in this case, 20°C). Cercarial production and movement were higher at 20°C only for Maritrema, and distance moved by Aporocotylid was lower at 20°C. Both these studies highlight that it is inappropriate to generalize across parasite species.
In a simulation analysis of snail (Bulinus globosus) population dynamics and production of S. haematobium cercariae based on a projected rise of 1° or 2°C (Kalinda et al. Reference Kalinda, Chimbari, Grant, Wang, Odhiambo and Mukaratirwa2018), maximum cercarial production and snail survival were predicted to be lower at the higher temperature, but snail reproduction was predicted to be higher. When Nguyen et al. (Reference Nguyen, Boersch-Supan, Hartman, Mendiola, Harwood, Civitello and Rohr2021) incorporated temperature-dependent relationships for nine snail and S. mansoni parameters into a Susceptible-Infected model framework, they found that the S. mansoni Basic Reproduction Ratio (Ro) was also temperature-dependent with a projected maximum at 21.7°C. Their models indicated that control measures would be most effective at lower temperatures and that snail control would reduce transmission more than controlling cercaria or drug treatment of the human population.
Digenean infection in snails has been shown to alter the snail thermal preference, infection-induced pathology, and community structure. When given a choice across a temperature gradient, H. trivolvis snails infected with Echinostoma spp. moved to a warmer temperature (25.4°C) than uninfected snails (23.3°C) (Wang et al. Reference Wang, Tattersall and Koprivnikar2018). Similarly, both T. szidati infection of L. stagnalis and Bilharziella polonica infection of Planorbarius corneus altered the thermal preference of the snail from a higher to a lower temperature (Żbikowska & Marszewska Reference Żbikowska and Marszewska2018). The volume of cataract per Diplostomum metacercaria in the eye of salmon and trout was reduced when fish that had been maintained at 15°C for 12 weeks were transferred to 5°C compared with fish held at 15°C for another 8 weeks (Klemme et al. Reference Klemme, Hyvärinen and Karvonen2021).
In nematodes, Ascaris eggs develop optimally at high temperatures but survive best at lower temperatures (Larsen and Roepstorff Reference Larsen and Roepstorff1999). The impact of temperature on free-living larvae is often linked to additional abiotic factors such as humidity and moisture content. For example, trichostrongylid larvae need faecal water to migrate away from faeces (Gyeltshen et al. Reference Gyeltshen, Kahn and Laurenson2022), and Cooperia oncophora infective larvae held in climate chambers survived temperature and drought stress better in soil than on vegetation (Knapp-Lawitzke et al. Reference Knapp-Lawitzke, von Samson-Himmelstjerna and Demeler2016). Infective larvae of several intestinal nematodes of cattle in western Canada were shown to survive overwinter by remaining within fecal pats in the snow (Wang et al. Reference Wang, Avramenko, Redman, Wit, Gilleard and Colwell2020). The positive association between snow cover days and cases of Trichinella britovi in wild boar in Latvia between 1976 and 1993 was explained by prolonged survival of nematode larvae in carcasses buried in the snow, and reduced trichinellosis in foxes in the Alps may be linked to a reduction in snow cover (Pozio Reference Pozio2022). The water content of soil can also have negative impacts. For example, the range expansion of lungworms (Rhabdias pseudospaerocephala) in invasive cane toads in Australia was associated with drier conditions perhaps because water-logged soil kills free-living larvae (Pizzatto et al. Reference Pizzatto, Kelehear and Shine2013).
Helminth response to temperature is modified by water chemistry
In aquatic systems, the impact of water temperature is often dependent on other dimensions of water chemistry. Among the studies on monogeneans, Villar-Torres et al. (Reference Villar-Torres, Montero, Raga and Repullés-Albelda2018) conducted a detailed study on the influence of temperature, pH, and salinity on Sparicotyle chrysophrii infection of gilthead sea bream. Low pH (7.0 compared with 7.9) reduced egg development and hatching, maximum egg hatching occurred between 14° and 22°C, and oncomiracidial survival was highest at the combination of 37 ppt and 18°C. Salinity had little impact. In aquaculture, tilapia from ponds with high levels of nitrogenous waste, high conductivity, and higher temperature were more likely to be infected with monogeneans (Cichlidogyrus spp, Gyrodactylus cichlidarum, and Scutogyrus longicornis), whereas Clinostomum marginatum metacercariae were more abundant at higher dissolved oxygen that may have benefitted the snail first intermediate host (Paredes-Trujillo et al. Reference Paredes-Trujillo, Mendoza-Carranza, del Río-Rodriguez and Cerqueda-García2022).
In the Himasthla elongata–periwinkle–blue mussel system, the swimming duration of Himasthla cercariae declined with increasing temperature regardless of pCO2 (Franzova et al. Reference Franzova, MacLeod, Wang and Harley2019). Experimentally induced severe high temperature in a low-tide environment revealed that the combination of Himasthla infection and high temperature increased mussel mortality at moderate intensities, but the negative impact of infection on mussel survival was reversed in mussels with more than 250 metacercariae (Selbach et al. Reference Selbach, Barsøe, Vogensen, Samsing and Mouritsen2020). When considered in isolation, cercarial emergence and infectivity to mussels were higher at 22°C compared with 10° and 16°C. However, given the shorter swimming window, shorter lifespan of cercariae, and higher infection-induced mortality of mussels at 22°C compared with 16°C, net transmission to mussels was lower at 22°C (Díaz-Morales et al. Reference Díaz-Morales, Bommarito, Vajedsamiei, Grabner, Rilov, Wahl and Sures2022).
Digenean co-infections respond differently to water conditions, as shown in a freshwater field setting (Paull & Johnson Reference Paull and Johnson2018) and in a simulated tidal cycle (Leiva et al. Reference Leiva, Manríquez, Aguilera and González2019). The field infection dynamics of Ribeiroia ondatrae and Echinostoma spp. in H. trivolvis and in chorus frog tadpoles and metamorphs were explored in the context of temperature, drying, water chemistry, and host density (Paull & Johnson Reference Paull and Johnson2018). In snails, Ribeiroia prevalence increased more rapidly over the summer in warmer ponds, whereas a more rapid decline in Echinostoma prevalence was observed in sites with large daily temperature fluctuations. In the chorus frog, Ribeiroia prevalence was higher in ponds that dried rapidly, whereas Echinostoma prevalence was influenced primarily by the density of infected snails. Tadpole deformities were positively associated with both Ribeiroia intensity and a higher combined concentration of dissolved phosphorus and nitrogen (Paull & Johnson Reference Paull and Johnson2018). When the intertidal snail Echinolitterina peruviana was maintained in a simulated tidal cycle, emergence of both echinostomid and philophthalmid cercariae was higher at 20°C than at 15°C and both cercariae survived better at 15°C. However, when pCO2 was also considered, philopthalmid emergence was higher at 1400 than 500 μatm regardless of temperature, whereas echinostamid emergence was highest at the higher temperature regardless of pCO2 (Leiva et al. Reference Leiva, Manríquez, Aguilera and González2019).
Evidence that temperature, rainfall, and salinity are relevant in a tropical coastal lagoon was shown in a 13-year, monthly survey of the digenean Oligogonotylus mayae in snails (Pyrgophorus coronatus) and in the fish (Mayaheros urophthalmus) that serves as both second intermediate host and definitive fish host (May-Tec et al. Reference May-Tec, Herrera-Castillo, Vidal-Martínez and Aguirre-Macedo2020). Prevalence in the snail as well as prevalence and mean intensity of metacercariae and adults responded with a time lag to water temperature, rainfall, and salinity. The authors speculated that the observed 2-year cycle for all digenean life cycle stages may be associated with El Niño-Southern Oscillation and that the increase in intensity over the 13-year study may reflect recovery from the devastating effect of Hurricane Isidore that had severe consequences on the snail and its parasite community (May-Tec et al. Reference May-Tec, Herrera-Castillo, Vidal-Martínez and Aguirre-Macedo2020).
Free-living stages of helminths are dispersed by wind, and UV radiation reduces survival and infectivity
Wind speed, direction, and currents influence schistosome transmission and helminth infracommunities. Based on a 4-year data set including daily wind speed and direction and reported cases of swimmers’ itch caused by the bird schistosome Trichobilharzia stagnicolae, and after controlling for the number of swimmers on a given day, the risk of swimmers’ itch was highest on mornings with gentle direct onshore winds that would carry cercariae toward the shore (Sckrabulis et al. Reference Sckrabulis, Flory and Raffel2020). Similarly, the risk of child infection with S. haematobium in Senegal was associated with a high density of Bulinus snails 90 m from shore (Jones et al. Reference Jones, Sokolow, Chamberlin, Lund, Jouanard, Bandagny, Ndione, Senghor, Schacht, Riveau, Hopkins, Rohr, Remais, Lafferty, Kuris, Wood and Leo2021) presumably because cercariae or infected snails would have drifted inshore. Interestingly, the risk of S. mansoni infection in the same study was highest at water access points where low water flow promoted survival of Biomphalaria pfeifferi. Results from a field survey by Vidal-Martínez et al. (Reference Vidal-Martínez, Velázquez-Abunader, Centeno-Chalé, May-Tec, Soler-Jiménez, Pech, Mariño-Tapia, Enriquez, Zapata-Pérez, Herrera-Silveira, Hernández-Mena, Herzka, Ordoñez-López and Aguirre-Macedo2019) suggested that currents may contribute to differences in the helminth infracommunities in the dusky flounder. Among the 43 helminth species (predominantly digeneans) recovered from 17 sites across the Yucatan Peninsula of Mexico, species diversity was higher in the Caribbean region than in the western or mid Yucatan Shelf. This may have resulted from an interruption of the east to west current that would reduce movement of free-living stages of parasites and invertebrate hosts into the Yucatan Shelf or from removal of top predators needed for completion of some of the life cycles (Vidal-Martínez et al. Reference Vidal-Martínez, Velázquez-Abunader, Centeno-Chalé, May-Tec, Soler-Jiménez, Pech, Mariño-Tapia, Enriquez, Zapata-Pérez, Herrera-Silveira, Hernández-Mena, Herzka, Ordoñez-López and Aguirre-Macedo2019).
In addition to the well-known impact of light on emergence of cercariae (Al-Jubury et al. Reference Al-Jubury, Kania, Bygumm and Buchmann2020; Soldánova et al. Reference Soldánová, Born-Torrijos, Kristoffersen, Knudsen, Amundsen and Scholz2022), UV radiation and sun exposure have been shown to reduce viability and survival of a variety of tapeworm and nematode eggs as well as infectivity of Schistosoma miracidia and cercariae (Hazell et al. Reference Hazell, Braun and Templeton2019).
Pollutants can have positive and negative effects on helminths
Much of the work on the impact of pollutants has focused on digeneans (see review by Marcogliese Reference Marcogliese2023). For example, agricultural pollutants affect E. trivolvis cercariae as evidenced by temperature-dependent reductions in swimming duration in response to an insecticide (carbaryl), an herbicide (atrazine), and a fungicide (azoxystrobin) (Billet et al. Reference Billet, Belskis and Hoverman2022). The complexity of pollutant–host–parasite interactions was seen in the net positive impact of agrochemicals on S. haematobium transmission. The harmful impacts on the parasite and on snail survival and reproduction were countered by an increase in snail biomass associated with more periphyton and fewer snail predators (Hoover et al. Reference Hoover, Rumschlag, Strgar, Arakala, Gambhir, de Leo, Sokolow, Rohr and Remais2020). In contrast, silver and gold nanoparticles were found to be effective both at killing Biomphalaria alexandrina snails and reducing the swimming ability of S. mansoni cercariae in a dose-dependent manner. Furthermore, exposure of cercariae to silver nanoparticles just prior to infection of mice reduced adult worm numbers, and exposure to gold nanoparticles lowered worm numbers compared with control mice (Moustafa et al. Reference Moustafa, Mossalem, Sarhan, Abdel-Rahman and Hassan2018).
Copper oxide and zinc oxide nanoparticles are both important anthropogenic pollutants (Neff & Dharmarajan Reference Neff and Dharmarajan2021 ). Both free-living and parasitic stages of Marshallagia marshalli, a trichostrongylid nematode of sheep, are sensitive to copper oxide and zinc oxide nanoparticles in vitro, as evidenced by concentration-dependent decreases in egg hatching, larval motility, and adult motility and survival (Jalali et al. Reference Jalali, Malekifard, Esmaeilnejad and Rezaie2021). This raises the possibility that nanoparticles in the environment may negatively affect free-living eggs and nematode larvae. Copper has also been suggested to influence transmission of Dirofilaria by altering vector competence (Neff & Dharmarajan Reference Neff and Dharmarajan2021). Exposure of larval mosquitoes to 600 ug/L copper, a concentration considerably below the U.S. Environmental Protection Agency safe drinking limit of 1300 ug/L, not only lowered survival of the mosquito larvae but also lowered survival and fecundity of Dirofilaria-infected adult females. This indicates that environmental exposure to copper concentrations exceeding 600 ug/L may reduce both mosquito abundance and the likelihood that Dirofilaria larvae will mature within the adult female mosquitoes.
In marine mammals, the blubber concentrations of three forms of DDT were higher in stranded Indo-Pacific finless porpoises whose death was attributed to verminous pneumonia due to heavy infections of Halocercus lungworms, compared with apparently healthy porpoises that died of trauma (Gui et al. Reference Gui, He, Zhang, Tu, Chen, Feng, Liu, Mai and Wu2018). Whether DDT increased host susceptibility to lungworms or nematode infection altered host DDT metabolism is unclear. In a 3-year study of Australian sea lion pups that were all infected with the nematode Uncinaria sanguinis, Taylor et al. (Reference Taylor, Terkildsen, McQuilty, Lee, Wing-Simpson and Gray2022) explored relationships among heavy metals, markers of anemia and immunity, and hookworm infection status. Among pups shedding hookworm eggs, above-median cadmium concentration was associated with lower packed cell volume, and above-median lead concentration was associated with higher platelet counts but with lower mean corpuscular haemoglobin concentrations, which are all indicators of anemia. These findings raise the possibility that cadmium and lead pollution modifies (and possibly exacerbates) hookworm-induced anemia (Tayler et al. Reference Taylor, Terkildsen, McQuilty, Lee, Wing-Simpson and Gray2022).
Helminths perceive chemical signals from the host
The concept that helminths use chemical signals to navigate within the host to their preferred site has been known for decades. However, it is becoming increasingly apparent that helminths also use chemicals to recognize, locate, and differentiate suitable hosts and to distinguish infected from uninfected hosts (see Wheeler et al. Reference Wheeler, Hallem and Zamanian2022). In digeneans, miracidia change their behaviour in the presence of cues released from competent host snails but not from non-host species. For example, Trichobilharzia ocellata miracidia changed direction more frequently when close to their host snail L. stagnalis but not when close to other snails, and the rate of miracidial penetration was higher in L. stagnalis ‘active’ zones where miracidia presumably detected the higher concentrations of snail cues (Hertel et al. Reference Hertel, Holweg, Haberl, Kalbe and Haas2006). In two-way choice chambers, S. mansoni miracidia avoided conditioned water from E. caproni-infected snails and preferred conditioned water from either uninfected snails or S. mansoni-infected snails. These findings indicate that miracidia detect and avoid snails that are infected with a dominant competitor. In contrast, E. caproni miracidia showed a preference for conditioned water from S. mansoni-infected snails over uninfected snails (Vannatta et al. Reference Vannatta, Knowles, Minchella and Gleichsner2020). This latter observation is consistent with the nutritional and reproductive benefit that E. caproni gains from infecting an S. mansoni-infected snail (Carpenter et al. Reference Carpenter, Vannatta and Minchella2021). A follow-up study (Laidemitt et al. Reference Laidemitt, Cleichsner, Ingram, Gay, Reinhart, Mutuku, Oraro, Minchella, Mkoji, Loker and Steinauer2022) extended these findings by showing that miracidia avoided snails infected with the dominant competitor Patigifer but moved toward snails infected with a subordinate xiphiodiocercarial infection or with the metacerarcial stage of Patagifer.
Infective third stage (L3) nematode larvae find their host by relying on a range of sensory cues, including soluble chemicals and volatiles emitted by skin, exhaled CO2, sweat, temperature, light, moisture, humidity, and vibrations that induce ‘crawling’ toward the host cue (Gang & Hallem Reference Gang and Hallem2016). The L3s of the sheep nematodes H. contortus and Teladorsagia circumcincta increase their likelihood of transmission by migrating vertically on vegetation in response to light, temperature, and humidity (Callinan & Westcott Reference Callinan and Westcott1986). In contrast, natural transmission of H. polygyrus usually occurs after the L1 and L2 larvae have ‘crawled’ away from the host faeces and the L3s have moved toward CO2. Interestingly, this ‘experience-dependent olfactory plasticity’ to CO2 has not been observed for skin-penetrating nematodes (Ruiz et al. Reference Ruiz, Castelletto, Gang and Hallem2017). Filarial infections in mosquitoes alter the attraction of infected mosquitoes to human chemical cues in a way that promotes transmission to the vertebrate host. Mosquitoes with non-infective first (L1) and second (L2) stage Brugia malayi larvae were less attracted to human chemical cues than mosquitoes with infective third stage (L3) larvae, indicating a co-evolved relationship that promotes filarial transmission (Gleave et al. Reference Gleave, Cook, Taylor and Reimer2016). Release of L3 larvae from mosquitoes while feeding may involve mechanical, thermal, and chemical cues (Wheeler et al. Reference Wheeler, Hallem and Zamanian2022).
There is considerable interest in whether chemical cues released from infected hosts alter the probability of subsequent infection. In the case of the digenean Coitocaecum parvum that infects amphipods as a second intermediate host, McPherson et al. (Reference McPherson, Friesen, Selbach and Poulin2018) found that amphipods did not respond to cues released from infected snails as there was no impact on amphipod behaviour or the subsequent infection by the cercariae. However, amphipods that were infected with C. parvum metacercariae were more likely to acquire more metacercariae, indicating that an existing infection promoted reinfection, a phenomenon that has been reported in other invertebrate infections. In contrast, di Bacco & Scott (Reference Di Bacco and Scott2023) reported that prior exposure to a putative chemical cue released by G. turnbulli-infected guppies delayed and dampened transmission in experimental epidemics.
Host social factors influence helminth transmission
Most of the research on social factors has focused on helminths of humans where virtually every social dimension directly or indirectly affects the probability of transmission and disease. Factors associated with poverty such as crowding, lack of access to clean water, solid and liquid waste disposal, education, and health care are often highlighted. For example, a survey of mono- and co-infections of Strongyloides stercoralis and hookworm in Cambodian villages demonstrated that those who walked more than 20 minutes to reach the health centre had an increased relative risk of co-infection, whereas boiling drinking water throughout the year reduced the relative risk of co-infection even though neither distance to the health centre nor boiling drinking water altered the risk of mono-infections (Forrer et al. Reference Forrer, Khieu, Schär, Vounatsou, Chammartin, Marti, Muth and Odermatt2018).
Social factors are also relevant for helminths of animals as highlighted by one recent example involving two species of zebras. Plains zebras need to drink every day and thus remain close to waterholes, compared with Grevy’s zebra that are more tolerant of dry conditions and range farther from water holes. These differences have been suggested to alter transmission of strongyle nematodes (Tombak et al. Reference Tombak, Easterling, Martinez, Seng, Wait and Rubenstein2022) as they affect dung density in the environment. Higher densities of dung were found near waterholes, and the decline in fecal egg counts with distance from the waterholes was steeper for plains zebras than for Grevy’s zebras.
As more studies consider social factors of non-human helminths, it seems extremely likely that a multitude of examples will be found where host social factors affect helminth transmission dynamics.
Helminths respond to host age, sex, and reproductive status
Among the many examples of the impact of host age and sex on helminth infections, a recent field study on German polecats highlighted the interrelationships of co-existing helminth infections with host age and sex. Using generalized linear mixed effects models, Frantz et al. (Reference Frantz, Salazar, Müller, Steinbach, Wittische and Heddergott2022) determined the most parsimonious explanation for the presence of the digenean Troglotrema acutum and the nematode Skryabingylus nasicola, both of which are transmitted primarily through paratenic hosts and live as adults in the polecat sinus cavity. Older polecats were more likely to be infected with Skryabingylus than younger polecats but only if polecats were co-infected with Troglotrema. Otherwise, the risk of Skryabingylus infection decreased with age. The likelihood of Skryabingylus infection was higher in females than males but, among polecats that were infected with Skryabingylus, males were more likely to be infected with Troglotrema than females.
Reproductive status is also relevant – at least for gastrointestinal nematodes. For example, a peri-parturient rise in trichostrongylid egg counts is commonly reported in livestock and is associated with a reduction in host immunity in the perinatal window (Beasley et al. Reference Beasley, Kahn and Windon2010). In a field study of male red deer, De La Peña et al. (Reference De La Peña, Martín, Barja, Pérez-Caballero, Acosta and Carranza2020) related the presence of helminths (predominately strongylids and Elaphostrongylus) to two indicators of reproductive effort: testosterone concentration and dark ventral patch size. The positive association between both indicators of reproductive effort and the presence of parasites was only detected when male–male competition occurred. The authors suggested that the negative impact of testosterone on host immunity increased susceptibility of males to infection, especially during periods of intense male–male competition. Furthermore, in a review by Coulson et al. (Reference Coulson, Cripps, Garnick, Bristow and Beveridge2018), it was evident that the risks and benefits of avoidance of infective nematode larvae through choice of grazing sites differed depending on host age, sex, and reproductive status.
Reviews by Vemuri et al. (Reference Vemuri, Sylvia, Klein, Forster, Plebanski, Eri and Flanagan2019) and Wesolowska (Reference Wesolowska2022) highlight the relevance of sex hormones on innate and adaptive immune responses to helminths, on ways that helminths have evolved to exploit sex hormones, on the distinctive microbiome between male and female hosts, and on the implications for prevention and control. The above examples align with the premise that routinely ignoring host sex in the studies of helminths may obscure our understanding of their ecological relationships.
Helminths are affected by host diet, food availability, and nutritional supplements
For over 50 years, it has been understood that malnutrition increases helminth infection primarily by dampening host immunity and that helminths, in turn, have a negative impact on host nutritional status (Scrimshaw et al. Reference Scrimshaw, Taylor and Gordon1968). Much of the experimental research on helminths has been conducted in mice and livestock, and insights have also emerged from epidemiological studies in human populations (Shea-Donohue et al. Reference Shea-Donohue, Qin and Smith2017; Scott & Koski Reference Scott, Koski, Humphries, Scott and Vermund2021). A few recent studies are extremely interesting given their complex design and their attempt to mimic natural interactions.
Resource competition negatively impacted not only B. glabrata but also S. mansoni, as the presence of an uninfected snail substantially reduced growth of the infected snail. Furthermore, when an infected and uninfected snail were paired, the growth and rate of daily cercaria production of the infected snail was negatively related to the size of the uninfected snail (Civitello & Hartman Reference Civitello and Hartman2021). The impacts of resource competition were also seen in mesocosms founded by small, medium, or large B. glabrata. Mesocosms were supplemented weekly with either a low or high concentration of nutrients to promote growth of edible periphyton algae and seeded biweekly with S. mansoni eggs (Civitello et al. Reference Civitello, Angelo, Nguyen, Hartman, Starkloff, Mahalila, Charles, Manrique, Delius, Bradley, Nisbet, ’hi and and Rohr2022). Temporal changes in algae, snail size, biomass, and reproduction, as well as total and per snail cercarial release, were monitored. As expected, snail numbers and biomass increased more quickly in the mesocosms founded by larger snails and provided with more nutrients, and snail numbers began to decline when algae were least abundant. Also as predicted, snails remained infected for longer in the high-nutrient mesocosms. However, resource competition affected per capita cercarial production, which decreased considerably as snail biomass peaked and algal supply declined, indicating that an assumption of higher transmission potential at higher host densities may not always apply.
Intriguing interactions have been described between H. diminuta, its rat host, and the plant Arabidopsis helleri that hyperaccumulates cadmium and zinc from the soil. Compared with uninfected rats, faecal excretion of cadmium and zinc was lower in H. diminuta-infected rats whose diet was supplemented with A. helleri, possibly because H. diminuta actively absorbs these trace elements (Sloup et al. Reference Sloup, Jankovská, Száková, Magdálek, Sloup and and Langrová2018). Moreover, tapeworms from rats fed this plant also bioaccumulated manganese relative to rat muscle concentrations, but iron concentration was lower in the tapeworm than in all tested host tissues (Jankovská et al. Reference Jankovská, Sloup, Száková, Magdálek, Nechybová, Peřinková and and Langrová2018).
The impact of low dietary protein on the murine nematode T. muris was investigated in outdoor enclosures where mouse foraging, activity, and selection of wild plants were also monitored (Budischak et al. Reference Budischak, Hansen, Caudron, Garnier, Kartzinel, Pelczer, Cressler, van Leeuwen and Graham2018). Low- versus high-protein diet treatments that began in the lab continued when mice were transferred to outdoor enclosures and then experimentally infected with T. muris and monitored for four weeks. Infected mice gained weight more slowly than uninfected mice, and mice in the low protein group allocated 30% more time per day to feeding, presumably to make up for dietary deficiencies. This and the absence of differences in most immunological measures suggest that the increased foraging may have been sufficient to overcome the impact of low protein on the parasite. At least 26 wild plants were selected by mice in the enclosures, primarily legumes grasses, wood sorel, roses, violets, and asters. Although there was no evidence that diet altered the wild plant selection, wild plants can contain antioxidants (Adamu et al. Reference Adamu, Naidoo and Eloff2014) and many have anthelmintic properties (see Ali et al. Reference Ali, Rooman, Mussarat, Norin, Ali, Adnan and Khan2021).
In a field experiment in Scotland, Sweeny et al. (Reference Sweeny, Clerc, Pontifes, Venkatesan, Babayan and Pedersen2021) provided high-quality whole-diet food supplements and anthelmintic treatment over 8 weeks to wood mice infected with H. polygyrus. Even transient access to supplemental food resulted in lower parasite egg output and fewer adult worms, higher body mass and fat mass of mice, and higher anthelmintic efficacy. Parallel lab experiments demonstrated that, as expected, high-quality nutrient supplements improved resistance to challenge H. polygyrus infection, but surprisingly, the benefit of high-quality supplements on body condition was only modest compared with other studies where supplementation followed the restriction of a single nutrient (see Sweeny et al. Reference Sweeny, Clerc, Pontifes, Venkatesan, Babayan and Pedersen2021). The authors suggest that, under field conditions, animals may have higher nutritional demand than in the lab and thus benefit from the supplemented high-quality diet that contains both micronutrients and macronutrients.
Moving forward
A full understanding of the life history of helminth parasites cannot rely on measures of prevalence, average abundance, and intensity of single or multiple helminths within a host population. There is now evidence that helminths can perceive whether a host is infected by other parasites that may positively (or negatively) affect their success. They interact in remarkable ways with other helminths and microbes that co-occur within the host, and some of these interactions have impacts that persist even after the helminth has left its host. Some of the most revealing hints to complexity and context-dependence come from experiments conducted in natural or semi-natural settings where a fuller scope of interactions can be tracked compared with studies done under controlled experimental conditions. Although more challenging to design, although the scope of relevant data (and relevant collaborators) seems endless, and although data analysis is much more complicated, these more natural experiments are a gateway to a fuller appreciation of the helminth’s perspective on its world.
The host lens
From the host perspective, the range of negative consequences of helminth infection on host survival and infection is well-known and not covered in this review. What insights do we have into ways in which microbes modulate the impact of helminths on the host? Might the presence of helminths benefit the host? This section provides evidence that, within the helminth community, co-existing species can exert opposing impacts that dampen negative impacts on host health, and some helminths limit pathology associated with microparasite infections by modulating host immunity. Helminth-induced shifts in the microbiome generate metabolites that are needed by the host, and many helminths bioaccumulate heavy metals, thus lowering tissue concentrations in their host. There is also recent evidence that helminth infection can alter host feeding ecology and enhance spatial memory of uninfected offspring to the benefit of the host. A summary of key hypotheses emerging from the studies described below is presented in Figure 2.
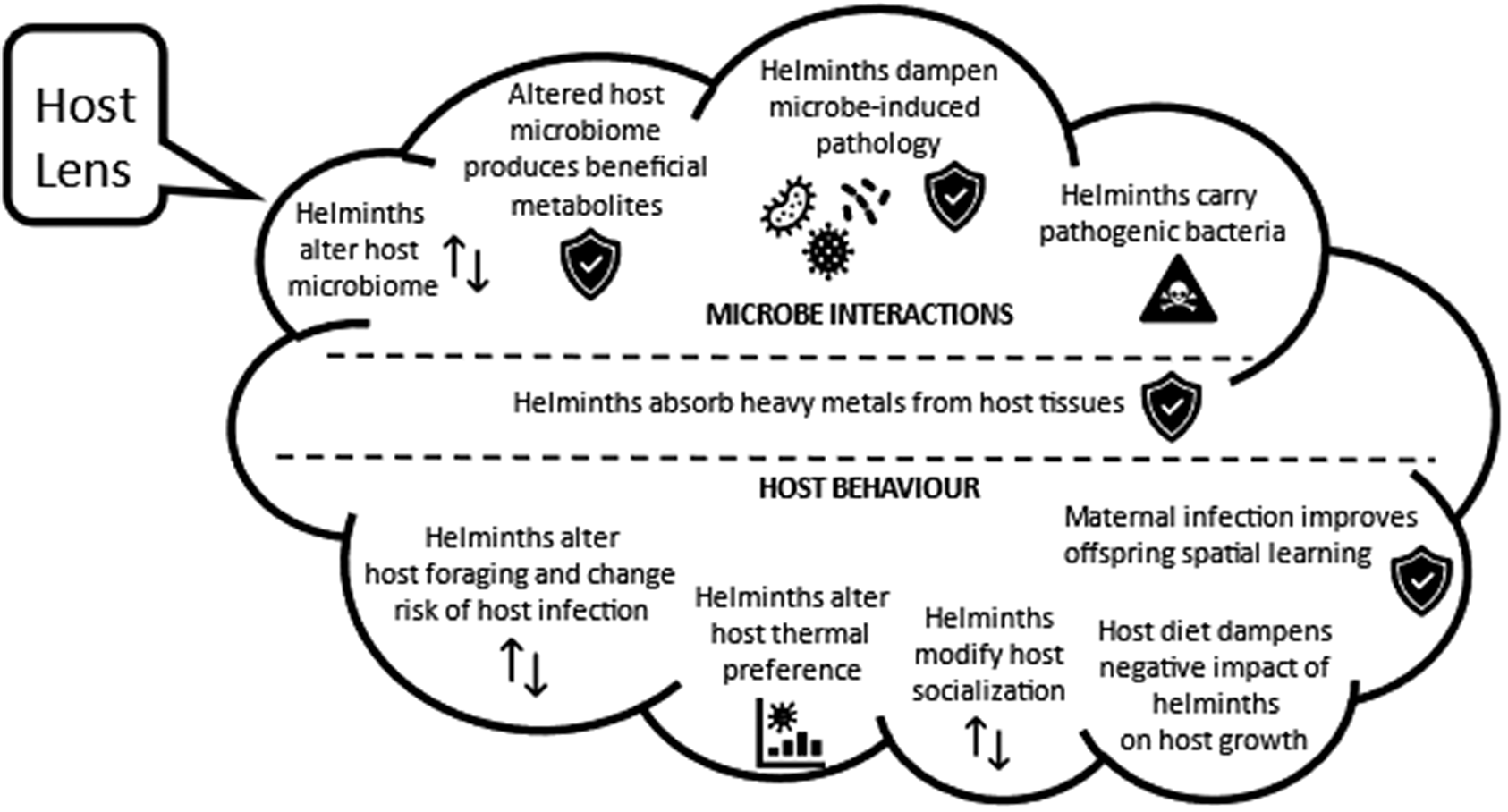
Figure 2. The host lens – hypothesized beneficial and harmful ways in which helminth infections may interact with host microbes, host heavy metals, and host behaviour, based on literature cited in this review.
Helminths bring microbes into the host
Helminths bring bacteria into the host that contribute to, or exacerbate, the pathology caused by the helminth infection (Morley Reference Morley2016). Several examples are seen with human nematode infections (Ashour & Othman Reference Ashour and Othman2020). Staphylococcus can be transferred by Toxocara larvae from the gut to tissues including the liver, causing liver abscesses. During disseminating strongyloidosis, juvenile nematodes transport bacteria to the blood and cerebrospinal fluid causing bacterial meningitis associated with pathogenic E. coli, Klebsiella pneumonia, Enterococcus faecalis, and Streptococcus bovis. In addition, hydatid cysts have become infected with Klebsiella, Streptococcus, and the fungus Aspergillus, all of which can increase the size of the cyst and the probability of rupture.
Helminth infections can also increase the risk of secondary bacterial infection. The extreme lymphedema that accompanies human brugian filariasis provides an ideal site for bacterial overgrowth unless proper and thorough hygiene is used (Ashour & Othman Reference Ashour and Othman2020), and the higher relative abundance of Campylobacter jejuni in A. suum-infected pigs raises the possibility of a risk of secondary infection with this pathogen (Wang et al. Reference Wang, Liu, Urban, Paerewijck, Geldhof and Li2019).
Helminths often dampen pathology of microparasite co-infections
The direct and indirect impact of helminths on host survival and reproduction is influenced in part by helminth interactions with co-existing microparasites. Microparasites can limit helminth pathology, as probiotic bacteria have been shown to lower schistosome and Trichinella infection and reduce granulomas caused by Toxocara (Ashour & Othman Reference Ashour and Othman2020). However, helminths can exacerbate microparasite infections. For example, prior infection of mice with the nematode H. polygrus increased the number of Toxoplasma gondii cysts in the spleen and then the brain, which is a result attributed to the immunoregulatory consequences of H. polygyrus infection that inhibited the ability of the host to control this protozoan during the early acute phase of infection (Rovira-Diaz et al. Reference Rovira-Diaz, El-Naccache, Reyes, Zhao, Nasuhidehvani, Chen, Gause and Yap2022).
Several lab models have been used to investigate the impact of filarial and intestinal nematodes on malaria, and many (but not all) show that nematode infection reduces Plasmodium parasitemia and host pathology. Despite higher early weight loss, pre-infection of gerbils with Brugia pahangi larvae lowered Plasmodium berghei parasitemia, reduced the drop in body temperature and hemoglobin and glucose concentrations, and prolonged survival compared to gerbils infected only with P. berghei (Junaid et al. Reference Junaid, Vythilingam, Khaw, Sivanandam and Mahmud2020). Pre-infection with Trichinella spiralis similarly lowered P. berghei parasitemia, although liver pathology and mouse survival were unchanged (Mei et al. Reference Mei, Ye, Chang, Huang, Song and Lu2020). Fairlie-Clarke et al. (Reference Fairlie-Clarke, Hansen, Allen and Graham2015) infected mice with Plasmodium chaboudi alone or simultaneously with a single dose of Nippostrongylus brasiliensis larvae. Secondary analysis of their data using statistical and mechanistic models revealed that low-dose Nippostrongylus infection slowed the increase in P. chaboudi parasitemia (Wait et al. Reference Wait, Kamiya, Fairlie-Clarke, Metcalf, Graham and Mideo2021). However, simultaneous infection of mice with P. berghei and Ascaris suum eggs had no impact on P. berghei parasitemia. Instead, co-infection increased lung damage and mortality and was associated with the more rapid migration of A. suum larvae through the lungs into the airways (Vieira-Santos et al. Reference Vieira-Santos, Leal-Silva, de Lima Silva Padrão, Ruas, Nogueira, Kraemer, Oliviera, Caliari, Russo, Fujiwara and Bueno2021).
Two human surveys indicate that Ascaris and Trichuris limit microparasite pathology, whereas hookworms exacerbate it, raising the likelihood that communities of pathogens may have co-evolved to limit host pathology. Abbate et al. (Reference Abbate, Ezenwa, Guegan, Choisy, Nacher and Roche2018) explored the direct and interactive effects of three nematodes – Trichuris trichiura, A. lumbricoides, and hookworm (primarily Necator americanus) – on cerebral malaria caused by Plasmodium falciparum. Co-infection with Ascaris or Trichuris reduced cerebral malaria, presumably because these infections dampen the immunopathology in the brain. However, the benefit provided by Trichuris was reduced by 46% in individuals co-infected with hookworm, which is perhaps due to the distinct type of immunity induced by hookworms. In a malaria-free region of rural Panama where virtually all indigenous pregnant women had vaginal infections and almost 20% had caries (González-Fernández et al. Reference González-Fernández, Koski, Sinisterra, EdC, Murillo and Scott2015), systemic inflammation (measured using C reactive protein) was positively associated with hookworm and caries but negatively associated with Ascaris and with two of the vaginal pathogens (González-Fernández et al. Reference González-Fernández, EdC, Rueda, Sinisterra, Murillo, Scott and Koski2017). Similarly, an indicator of maternal hypertension linked to poor fetal outcomes was positively associated with hookworm but negatively associated with Ascaris and one of the vaginal pathogens (González-Fernández et al. Reference González-Fernández, EdC, Rueda, Sinisterra, Murillo, Scott and Koski2020). It seems entirely plausible that similar co-evolved communities of helminths and microparasites exist in wild animals and that they moderate the negative impact of any one infection. These examples highlight the value of applying a systems approach in studies on the ecology of infections in natural populations, as recommended by Garza-Cuartero et al. (Reference Garza-Cuartero, Garcia-Campos, Zintl, Chryssafidis, O’Sullivan, Sekiya and Mulcahy2014).
Of three viral-helminth co-infection studies, helminth infection was beneficial in reducing viral pathology in two studies but not the third. Hyla versicolor tadpoles are host to ranaviruses such as FV-3 and to larval echinostomes (Echinoparyphium sp.). Prior infection with echinostome cercariae was beneficial to the host as it reduced FV-3 viremia and limited host mortality (Wuerthner et al. Reference Wuerthner, Hua and Hoverman2017). Similarly, co-infection of mice with S. mansoni and the pneumonia virus resulted in less weight loss and lower viremia compared with mice infected only with the virus (Scheer et al. Reference Scheer, Krempl, Kallfass, Frey, Jakob, Mouahid, Moné, Schmitt-Gräff, Staeheli and Lamers2014). However, co-infection of mice with H. p. bakeri and West Nile virus increased mouse mortality (Desai et al. Reference Desai, Janova, White, Reynoso, Hickman, Baldridge, Urban, Stappenbeck, Thackray and Diamond2021b).
Helminth-induced changes to the host microbiome provide beneficial metabolites
Nematode-induced shifts in the host microbiome can alter the profile of microbial metabolites in a direction that may have benefits for the host. Short-chain fatty acid (SCFA) concentrations were elevated in the colon of A. suum-infected pigs and in the caecum and portal blood of H. bakeri-infected mice (Zaiss et al. Reference Zaiss, Rapin, Lebon, Dubey, Mosconi, Sarter, Piersigilli, Menin, Walker, Rougemont, Paerewijck, Geldhof, McCoy, Macpherson, Croese, Giacomin, Loukas, Junt, Marsland and Harris2015). Maternal H. bakeri infection in mice altered the stomach microbiome of their uninfected pups (Haque et al. Reference Haque, Koski and Scott2021). The predicted pup stomach metabolome had upregulated SCFA production as well as essential and branched-chain amino acids and vitamins, all of which may provide nutritional or developmental benefits for the uninfected pup (Haque et al. Reference Haque, Koski and Scott2021). Kennedy et al. (Reference Kennedy, Brosschot, Lawrence, FitzPatrick, Lane, Mariene, Wasmuth and Reynolds2021) found that the higher concentrations of isovaleric acid in the proximal small intestine of H. polygyrus-infected mice was dependent on the presence of bacteria. Furthermore, they identified a benefit to the parasite, as addition of isovaleric acid to the drinking water increased worm fecundity. In contrast to these examples, the altered microbial community resulting from infection of pigs with A. suum was negatively associated with carbohydrate and amino acid metabolism and may increase the vulnerability of the infected pig to microbial invasion (Wang et al. Reference Wang, Liu, Urban, Paerewijck, Geldhof and Li2019).
Helminths bioaccumulate heavy metals
It has been known for over two decades that acanthocephalans and tapeworms that absorb nutrients across their tegument bioaccumulate heavy metals including cadmium and lead from their fish definitive hosts in both marine and freshwater settings (Mehana et al. Reference Mehana, Khafaga, Elblehi, El-Hack, Naiel, Bin-Jumah, Othman and Allam2020). Whether acanthocephalans actively compete with the host for these heavy metals is unclear (Hassanine et al. Reference RME-S, Al-Hasawi, Hariri and HE-S2019), but the negative relationship between parasite numbers and concentrations per worm reported by Costa Duarte et al. (Reference Costa Duarte, Lehun, Leite, Consolin-Filho, Bellay and Takemoto2020) suggests dilution of cadmium among parasites at higher-intensity infections. There is also evidence that cadmium and lead become concentrated through the acanthocephalan life cycle. Mijošek et al. (Reference Mijošek, Marijić, Dragun, Ivanković, Krasnići and Erk2022) reported that concentrations of cadmium and lead were higher in the acanthocephalan Dentitruncus truttae than in both the intermediate host Gammarus balcanicus and the brown trout definitive host. Tapeworm bioaccumulation has been reported from fish, bird, and mammalial hosts. Adult Proteocephalus macrophallus in butterfly peacock bass collected from rivers of Southeastern Brazil bioaccumulated arsenic, cadmium, and lead (Leite et al. Reference Leite, Januário, Padilha, do Livramento, de Azevedo and Abdallah2019); Atractolytocestus huronensis bioaccumulated chromium, nickel, and platinum relative to liver and muscle tissue samples from common carp (Erasmus et al. Reference Erasmus, Wepener, Nachev, Zimmermann, Malherbe, Sures and Smit2020); concentrations of a wide range of heavy metals were four orders of magnitude higher in the tapeworm Raillietina saudiae than in the infected pigeons (Quraishy et al. Reference Quraishy, Abdel-Gaber, Alajmi, Dkhil, Jawher and Morsy2019); and lead concentrations were up to 5-fold higher in the rat tapeworm H. dimunata than in the rat liver (Tripodi et al. Reference Tripodi, Hancke and Suarez2018).
Even nematodes and adult digeneans that ingest food and contaminants bioaccumulate heavy metals. Among nematodes, Contracaecum spp. in the Sharptooth catfish bioaccumulated nickel, lead, and platinum (Erasmus et al. Reference Erasmus, Wepener, Nachev, Zimmermann, Malherbe, Sures and Smit2020); Cucullanus spp. in prawns (Macrobrachium vollenhovenii) had extraordinarily high bioaccumulation factors for chromium (>10,000) relative to the host intestine (Bamidele et al. Reference Bamidele, Lawal-Are, Iniobong, Uchenna, Kayode and David2020); and nematodes in sea lions have been shown to bioaccumulate mercury (McGrew et al. Reference McGrew, O’Hara, Stricker, Salman, Van Bonn, Gulland, Whiting and Ballweber2018). Three species of adult digeneans (Acanthostomum absconditum, Acanthostomum spiniceps, and Haplorchoides cahirinus) in the silver catfish bioaccumulated iron and magnesium (Mashaly et al. Reference Mashaly, El-Naggar, El-Tantawy and Al-Gaafari2021) and more so in summer and autumn compared with winter and spring. The intestinal digenean Ityogoniumus spp bioaccumulated mercury and lead in Iberian moles collected from agricultural fields in Spain (Adalid et al. Reference Adalid, Torres, Miñarro, Miquel, Fuentes and Eira2019). The authors suggested that mercury may have come agricultural application of fungicides and insecticides and that lead from lead shot may have bioaccumulated in earthworms that were then eaten by moles.
Only a few studies have compared bioaccumulation among multiple helminths within the same host. For example, Leite et al. (Reference Leite, WdRP, de Azevedo and Abdallah2021) examined the freshwater fish Hoplias malabaricus that was infected with larval Hysterothylacium sp. nematodes in the stomach and intestine and adult Phyllodistomum sp. digeneans in the urinary bladder. Bioconcentration of chromium, lead, and aluminum was high in larval nematodes, and the digenean had higher lead concentrations relative to host muscle samples. Canonical discriminant analysis clearly separated trace metal concentrations between mono-infected and co-infected fish (Leite et al. Reference Leite, WdRP, de Azevedo and Abdallah2021).
Finally, it seems that trace and heavy metals influence the diversity of helminth communities. A survey of raccoons infected with one acanthocephalan, one tapeworm, and five intestinal nematodes showed that intestinal helminth species richness was higher in sites where raccoons had a higher liver selenium concentration, and that total parasite abundance was positively associated with liver mercury concentration (Borchert et al. Reference Borchert, Leaphart, Bryan and and Beasley2019). However, Prüter et al. (Reference Prüter, Franz, Auls, Czirják, Greben, Greenwood, Lisitsyna, Syrota, Sitko and Krone2018) found that a higher concentration of lead in mallard ducks was associated with both lower diversity of the 19 species of digeneans, cestodes, nematodes, and acanthocephalans, and with a lower total number of helminth individuals in the mallard intestine. They also noted that lead concentrations in the host coracoid bone were lower in mallards infected with tapeworms or acanthocephalans, indicating the likelihood that these parasites bioaccumulated lead.
To date, the potential impact of helminth bioaccumulation on the host and on the helminths remains speculative.
Helminths affect host behaviour
Several recent reviews have explored the impact of helminth infection on host behaviour (Coulson et al. Reference Coulson, Cripps, Garnick, Bristow and Beveridge2018; Buck Reference Buck2019; Hawley et al. Reference Hawley, Gibson, Townsend, Craft and Stephenson2021). Throughout the present review, evidence has been provided that echinostome infection alters the thermal preference of snails (Wang et al. Reference Wang, Tattersall and Koprivnikar2018; Żbikowska & Marszewska Reference Żbikowska and Marszewska2018), and that helminths increase host susceptibility to predation, as seen by E. californiensis metacercariae in California killifish (Helland-Risse et al. 2020), C. australis metacercariae in cockles (Leung et al. Reference Leung, Keeney and Poulin2010), and D. dentriticum metacercariae in ants (Criscione et al. Reference Criscione, van Paridon, Gilleard and Goater2020). Of note, it now seems that the increased susceptibility of S. solidus-infected three-spined stickleback to predation results from a generalized lethargy. Although this promotes transmission to the definitive host, infected sticklebacks are also more susceptible to predation by non-host predators (Svensson et al. Reference Svensson, Eghbal, Eriksson and Nilsson2022).
The ability of hosts to forage optimally can be affected by helminth infection. This has been clearly demonstrated for metacercariae of the eye fluke Tylodelphys clavata in both European perch and bullies. Compared with uninfected perch feeding on an isopod (Asellus aquaticus) and a cladoceran (Daphnia magna), infected perch attacked both prey species at a closer distance and had lower attack success on isopods but not cladocerans (Vivas Muñoz et al. Reference Vivas Muñoz, Bierbach and Knopf2019). When pairs of juvenile perch preyed on the isopod, the more heavily infected individual was a less effective forager with longer reaction times (Vivas Muñoz et al. Reference Vivas Muñoz, Staaks and Knopf2017). In addition, more heavily infected perch selected benthic macroinvertebrates, whereas fish with fewer metacercariae also fed on zooplankton (Vivas Muñoz et al. Reference Vivas Muñoz, Feld, Hilt, Manfrin, Nachev, Köster, Jochmann, Schmidt, Sures, Ziková and Knopf2021). Compared with uninfected bullies, T. clavata-infected bullies had increased brain c-Fos gene expression following exposure to a flashing light for 15 min, suggesting that high intensity infections interfere with the ability of the fish to discriminate among visual cues (Stumbo et al. Reference Stumbo, Poulin and Ruehle2021). This was consistent with the observation that more heavily infected bullies hid in shelters near the food source, presumably allowing them easier access to the food to compensate for their impaired vision (Ruehle & Poulin Reference Ruehle and Poulin2021).
Larval digenean infection has been shown to impact both snail grazing (Vivas Muñoz et al. Reference Vivas Muñoz, Hilt, Horák and Knopf2018) and mussel filtration (Selbach et al. Reference Selbach, Marchat and Mouritsen2022). In snails, Cotylurus sp. infection of Planorbarius corneus doubled the snail’s mass-corrected grazing rate on periphyton, whereas T. szidati infection of L. stagnalis reduced the grazing rate by approximately half. No impact was detected for Trichobilharzia regenti infection of Radix lagotis or for D. pseudospathecum infection of L. stagnalis. Vivas Muñoz et al. (Reference Vivas Muñoz, Hilt, Horák and Knopf2018) speculated that the differences among these host–parasite combinations may be a function of whether the parasite causes castration or gigantism. In contrast to snails, establishment of H. elongata metacercariae in mussels (Mytilus edulis) was positively associated with filtration rate (Selbach et al. Reference Selbach, Marchat and Mouritsen2022). Moreover, exposure to cercariae reduced the filtration rate of infected but not uninfected mussels. Although this would reduce accumulation of metacercariae in the infected mussel, it would also limit food intake (Selbach et al. Reference Selbach, Marchat and Mouritsen2022). To the extent that filtration is an ecosystem service, this may have downstream consequences for coastal ecosystems.
Helminths have also been shown to affect foraging of monkeys. Agostini et al. (Reference Agostini, Vanderhoeven, Pfoh, Tiddi and Beldomenico2023) explored the impact of anthelmintic treatment in the context of supplemental provision of bananas (low or high levels) on foraging by black capuchin monkeys (Sapajus nigritus) throughout the winter in Argentina. Untreated monkeys infected with the nematode Filariopsis sp. and an Hymenolepididae tapeworm spent more time foraging than treated monkeys, possibly indicating a higher metabolic cost of infection. However, this difference in foraging behaviour was only found at the low level of banana supply. Perhaps the nutritional benefit of the higher supply of bananas lasted longer, eliminating the need for the infected monkeys to feed more frequently.
Three studies showing that diet has a stronger impact on host fitness than infection also reveal important nuances. In an attempt to disentangle the impacts of tapeworm infection and host nutritional status as potential explanations for different growth profiles of young-of-the-year European perch among three lakes, Frantz et al. (Reference Frantz, Perga and Guillard2018) concluded that diet (and an earlier switch to littoral prey that occurs once perch reach a certain size) was more responsible for differences in size than parasitism. However, in the lake where perch were heavily infected, it seems likely that tapeworm infection had a secondary impact on host growth by altering liver lipidogenesis and, in turn, delaying the switch of diet to the littoral zone (Frantz et al. Reference Frantz, Perga and Guillard2018). A field study in the Iberian Peninsula explored the relative importance of food quality and infection with the lungworm Dictyocaulus on chronic stress of roe deer that compete with free-range livestock when high-quality, oak forest forage is limited. Although faecal samples revealed a higher prevalence and intensity of Dictyocaulus in plots where roe deer overlapped with cattle (Horcajada-Sánchez et al. Reference Horcajada-Sánchez, Escribano-Ávila, Lara-Romero, Virgós and Barja2019), there was no evidence that Dictyocaulus directly contributed to chronic stress, which was measured using fecal cortisol. Instead, stress was highest when roe deer shared the poor-quality pine habitat with cattle (Horcajada-Sánchez et al. Reference Horcajada-Sánchez, Escribano-Ávila, Lara-Romero, Virgós and Barja2019). In a third example, when lambs were given a choice of a diet with or without plant bioactive molecules (polyphenols and terpenes), uninfected lambs and those recently infected with H. contortus preferred the basal diet without bioactive molecules, whereas lambs infected for 3 weeks selected the diet with the bioactive molecules. Although this choice had no impact on worm numbers or indicators of immunity or pathology measured up to 3 weeks post-infection, the host efficiency of converting feed intake to weight gain was improved (Poli et al. Reference Poli, Thornton-Kurth, Legako, Bremm, Hampel, Hall, Ipharraguerren and Villalba2018).
Two recent studies consider the impact of infection on the socialization of the host. In a field study of vervet monkeys infected with a wide variety of nematodes, Chapman et al. (Reference Chapman, Friant, Godfrey, Liu, Sakar, Schoof, Sengupta, Twinomugisha, Valenta and Goldberg2016) conducted behavioural observations prior to and after anthelmintic treatment. They observed an increased frequency of interactions following anthelmintic treatment, especially for juveniles, and speculated that removal of the nematodes would have reduced the risk of transmission during social interactions. In contrast, in the guppy–monogenean (G. turnbulli) system, males that were heavily infected were more likely to socialize with other males compared with males with low-intensity infections (Stephenson Reference Stephenson2019). In both cases, mechanisms by which the presence of helminths would influence social interactions are unclear.
As early as the 1990s, there was interest in whether helminths influenced host cognition (Townsend et al. Reference Townsend, Sewall, Leonard and Hawley2022). Recently, maternal infection with H. bakeri was shown to improve spatial memory of uninfected mouse offspring (Noël et al. Reference Noël, Fortin-Hamel, Haque and Scott2022). After a repeated trickle infection during pregnancy and lactation, spatial learning and memory of the uninfected pups were tested beginning at 23 days of age using a Barnes Maze. Pups of both infected and uninfected dams rapidly learned the location of the escape box and remembered it one day later, indicating no impact of maternal infection on spatial learning or short-term memory. However, one week later, pups of infected dams retained their ability to find the escape box, but pups of uninfected dams did not, indicating improved long-term spatial memory as a consequence of the maternal infection. This finding was consistent with a previous brain gene expression study that revealed elevated expression of genes required for memory retention in 7-day-old pups of H. bakeri-infected compared with uninfected dams (Haque et al. Reference Haque, Koski and Scott2019). The demonstration that a nematode restricted to the maternal intestine improves spatial memory of the uninfected offspring raises many ecological and evolutionary questions.
Moving forward
The presence of helminth infection can be both detrimental and beneficial to the host. Beyond the direct pathology that they induce, it is now clear that helminths may modulate host responses to co-infections in a direction that reduces host pathology, that they reduce concentrations of heavy metals in the host, that they may either increase or reduce host feeding, and even that they may improve spatial memory of uninfected offspring. This expanding scope of impacts on the host is a reminder that simplistic perspectives on the association of helminths with their hosts may, at least in some cases, ignore key processes of relevance to host ecology and even to the control of helminths.
The environment lens
Turning to an environment lens, what evidence do we have that the individual infected host or host population impacts the community of interacting competitors, predators, and prey, and even biodiversity? Might helminths even impact the abiotic environment? Is there any evidence that these host–helminth–environment interactions are influenced by global environmental change, and if so, under what conditions is transmission likely to be promoted or limited? The studies below provide a ‘taste’ of the extent to which helminths respond to, and influence, the biotic composition and diversity of host communities, with ramifications for the ‘health’ of the environment. Even the abiotic environment can be impacted as shown by an increased methane release from helminth-infected livestock. Consideration of helminths is therefore important for decisions on how to manage wildlife populations and what to expect from global environmental changes such as climate change and land-use change. A summary of key hypotheses emerging from the studies below is presented in Figure 3.

Figure 3. The environment lens – hypothesized beneficial and harmful ways in which helminths shape the environment and are affected by environmental change, based on literature cited in this review.
Helminths influence the biotic environment
The impact of helminth infection extends well beyond any harm or benefit it may provide to its host. The following examples used mesocosms to explore interactions in various combinations of single or multiple helminths in communities with multiple hosts, with prey, or with predators, as well as impacts at the landscape level.
Using mesocosms, Friesen et al. (Reference Friesen, Poulin and Lagrue2018) demonstrated that the outcome of competition between two isopods – Austridotea annectens and A. lacustris – for preferred habitats was altered when A. annectens was infected with Maritrema poulini metacercariae. Regardless of competition with A. lacustris or infection with Maritrema, A. annectens was more commonly found under the sand. However, site preference of A. lacustris differed depending on whether its competitor was infected or not. When an uninfected competitor was present, A. lacustris was more frequently found under the sand than on rocks, but the opposite was seen if the competitor was infected. The authors suggested that the infection-induced shift of the uninfected amphipod species away from the sand may increase the probability that birds will prey on the infected species, increasing transmission of Maritrema to its definitive host. In a follow-up study, Friesen et al. (Reference Friesen, Goellner, Poulin and Lagrue2020) used mesocosms to explore the impact of the introduction of Maritrema cercariae into a more complex community that included the same two isopods as well as two species of amphipods, two digeneans, one nematode, and one acanthocephalan parasite. Although Maritrema infected all four species of crustaceans, ongoing exposure to cercariae reduced the survival and fecundity of one of the amphipods (Paracolliope fluviatilis), allowing relative abundance of its competitor, P. excavatum, to increase.
Anaya-Rojas et al. (Reference Anaya-Rojas, Best, Brunner, Eizaguirre, Leal, Melián, Seehausen and Matthews2019) explored ecosystem-level impacts of helminths in mesocosms containing male three-spined sticklebacks that were either naturally infected with two digeneans (Apatemon spp. and Diplostomum spp.), one monogenean (Gyrodactyus spp), and a protozoan (Ichthyopthirius multifillis), or that had received anthelmintic treatment. Guppies were maintained for 33 days along with zooplankton, zoobenthos, and phytoplankton as primary producers, and several ecosystem parameters were compared across two fish densities, two levels of infection (natural and anthelmintic-reduced), and two fish lineages. Pelagic trophic cascades were more evident in mesocosms with reduced parasite load – especially reduced Gyrodactylus infection – and this was linked to a weaker impact of the high density sticklebacks on the zooplankton community when fish were infected.
Preston & Sauer (Reference Preston and Sauer2020) highlighted the impact of snail castration on snail demographics by linking their theoretical studies with empirical data from mesocosms containing two species of snails and four digeneans: Helisoma trivolvis and its three digeneans, Ribeiroia ondatrae, Echinostoma trivolvis, and Alaria mestelae, and Physa gyrina infected with Echinostoma sp. When the initial density of Helisoma was high, digenean-induced snail castration reduced production of snail egg masses and juvenile snails, allowing more rapid juvenile growth with a rapid increase in juvenile biomass. Following this biomass overcompensation, the population of juveniles declined due to lack of recruitment. This study highlights that consideration of stage-specific traits and responses is an important part of understanding host–parasite population dynamics.
Another elegant mesocosm study by Strasburg & Boone (Reference Strasburg and Boone2022) explored the community dynamics of the snail (Planorbella [H.] trivolvis) and frog (Rana pipiens) intermediate hosts of the digenean Echinostoma spp. and their three predators: bluegill sunfish that prey on snails and small and large tadpoles, as well as cercariae; western mosquito fish that prey on small tadpoles and cercariae; and crayfish (Orconectes rusticus) that attack tadpoles and reduce the abundance of snails. Of the three predators, the bluegill exerted the strongest overall impact on the system as it eliminated the negative impact of echinostomes on metamorph survival, reduced snail survival, and lowered the number of echinostome metacercariae in metamorphs and overwintering frogs. Furthermore, both the parasite and bluegill contributed to the larger size of the frogs at metamorphosis.
Finally, theoretical efforts that attempt to track this complexity have highlighted often unexpected outcomes. For example, based on the S. mansoni–B. glabrata system, Malishev & Civitello (Reference Malishev and Civitello2019) combined an individual-based model of populations of susceptible and infected snails, snail eggs, snail food resources, miracidia, and cercariae and their relevant linkages with an energetic model that tracked parasite and host biomass over one transmission season. In addition to the expected feedbacks over time, they found that cercarial density did not respond linearly to infected host density because increasing resource competition among B. glabrata through the season negatively affected snail conditions, limiting host resources for the parasite and reducing cercarial output.
Together, these examples clearly demonstrate that individual helminth infections directly and indirectly influence interactions among host species and highlight the need for experimental studies that begin to capture the complexity of natural systems.
Helminth associations with host biodiversity and invasive species
Helminth community structure can be disrupted by invasive species. Loxton et al. (Reference Loxton, Lawton, Stafford and Holland2017) explored the impact of bank voles that are considered an invasive species in Ireland on the native wood mouse helminth community by sampling two sites with only wood mice and two sites with wood mice and bank voles over 2 years. The wood mouse helminth community included four intestinal nematodes, two adult tapeworms, two larval tapeworms and two intestinal digeneans. Comparisons between invaded and uninvaded sites revealed three interesting findings. The abundance of the nematode Syphacia stroma was higher in the uninvaded sites. This was interpreted as an example of a dilution effect whereby the presence of bank voles reduced the density of wood mice, which, in turn, reduced mouse–mouse contact and direct transmission of this nematode. In contrast, the abundance of the nematode Aonchotheca murissylvatici was higher in invaded sites and was only detected in the second year of the study. This may be an example of spillback from the invasive voles that seem to be a more competent host than wood mice. Finally, the tapeworm Taenia martis, not known to be a parasite of wood mice, was found only in invaded sites. This may be an example of spillover from the invading bank vole to native wood mice, as a previous study had found that bank voles were infected with T. martis in these invaded sites. Follow-up studies will be useful in confirming the hypotheses emerging from the study.
The impact of top predators on three nematodes of the East African pouched mouse was compared in 4-hectare wildlife enclosures in the Kenyan Savannah that were either natural control plots or ‘exclosure’ plots where exclusion of predators and megaherbivore populations resulted in higher mouse density (Weinstein et al. Reference Weinstein, Titcomb, Agwanda, Riginos and Young2017). The higher prevalence and mean abundance of the nematode Neoheligmonella in ‘exclosure’ plots was likely mediated indirectly through an increase in mouse density resulting from fewer grazing mammals and predators. The magnitude of difference between plots was dampened during periods of high rainfall that would promote survival of larval nematodes regardless of the presence or absence of predators and large herbivores. Similar results were observed for the nematode Syphacia but only after removal of an outlier whose extremely heavy infection was likely due to autoinfection. Rainfall was negatively correlated with Syphacia intensity. Prevalence and abundance of Trichuris were similar in control and ‘exclosure’ plots. This study demonstrates that removal of top predators may increase infection levels by releasing predation pressure even on rodents but that results differ between parasites and are affected by abiotic conditions.
Impact of helminths on the abiotic environment
Only rarely has the impact of helminths on the abiotic environment been explored or documented. However, in a study on methane (CH4) emissions (g/d and g/kg body weight) from lambs, emissions were higher from lambs infected with Trichostrongylus colubriformis and H. contortus than from uninfected lambs (Lima et al. Reference Lima, Crouzoulon, Sanches, Zabré, Kabore, Niderkorn, Hoste, do Amarante, Costa-Júnior, Abdalla and and Louvandini2019), which is consistent with an earlier study of T. circumcincta in sheep (Fox et al. Reference Fox, Smith, Houdijk, Athanasiadou and Hutchings2018). This finding raises the possibility of an environmental benefit of anthelmintic treatment of livestock.
Helminths and environmental management
One of the many strategies envisioned for adapting the natural environment to accommodate human-induced changes is rewetting of grasslands. May et al. (Reference May, Raue, Blazejak, Jordon and Strube2022) considered whether this practice would increase transmission of helminth parasites of sheep and cattle, as larval survival, development, and migration to the tip of vegetation are moisture dependent, and as most snail hosts of digeneans require moist habitats. However, in their 3-year evaluation of a pasture rewetting initiative that had started 11 years earlier, they found no evidence that rewetting had altered helminth prevalence or abundance.
‘Food provisioning’ is one approach to the management of wildlife populations whose habitat has been limited or altered by anthropogenic changes. It is an approach that is especially popular given the psychological benefits to those compelled to help wildlife by providing them with food (see Cox & Gaston Reference Cox and Gaston2018). Food provisioning is potentially beneficial as the improved nutritional status of wild animals is likely to boost immune responses, thus reducing helminth transmission among wildlife (Strandin et al. Reference Strandin, Babayan and Forbes2018). However, as described by Altizer et al. (Reference Altizer, Becker, Epstein, Forbes, Gillespie, Hall, Hawley, Hernandez, Martin, Plowright, Satterfield and Streiker2018), food provisioning also concentrates animals at the sites of supplemental food. The higher host density may increase transmission of some helminths due to higher probability of contact with infective stages and may also increase stress that, in turn, may lower immune responsiveness. Also, concentrating animals and/or supplemental food may alter the host microbiome with consequences for susceptibility to infection. Wright & Gompper (Reference Wright and Gompper2005) compared helminth communities among sites prior to and during focal food supplementation, as well as control sites where supplemented food was dispersed through the landscape, and their results provide evidence that focal food provisioning can increase helminth transmission. Raccoons with access to food supplementation at focal points had higher helminth infracommunity richness as well as higher prevalence of two nematodes: Placoconus lotoris (transmitted by penetration of larvae) and Baylisascaris procyonis (transmitted by ingestion of eggs). Some of the negative consequences of food provisioning can be mitigated by dispersing the food rather than concentrating at focal locations (Strandin et al. Reference Strandin, Babayan and Forbes2018).
Gecchele et al. (Reference Gecchele, Pedersen and Bell2020) examined associations of social and environmental variables with red fox (Vulpes vulpes) helminths based on scat samples collected from greenspaces within Edinburgh, Scotland. Neither human density nor road cover emerged as significant in statistical models. However, both prevalence and intensity of the hookworm Uncinaria stenocephala and of the airway nematode Eucoleus aerophilus were positively associated with greenspace connectivity and ‘wildness’ of the landscape. Thus, connectivity that enhances the ability of foxes to move through the urban space and to find prey such as rabbits is likely to promote transmission. When wildlife species are drawn to urban settings – for example, by provision of supplemental food – the potential for movement of animal parasites into humans or pets and vice versa becomes a risk. For example, the increase in prevalence of the zoonotic cestode Echinocococcus multilocularis in the red fox population in southern Germany was associated with a subsequent doubling of human cases of alveolar echinococcosis (Schweiger et al. Reference Schweiger, Ammann, Candinas, Clavien, Eckert, Gottstein, Halkic, Muellhaupt, Prinz, Reichen, Tarr, Torgerson and Deplazes2007).
Finally, in reflecting on the potential relevance of helminths for environmental management, it is important to note the study by Wilber et al. (Reference Wilber, Briggs and Johnson2020). The authors explored amphibian mortality caused by Ribeiroia metacercariae from a landscape perspective. Though not surprising given our knowledge that helminths are aggregated in host populations, they demonstrated that this aggregation also extends to the spatial distribution of Ribeiroia-induced host mortality. By combining experimental, field, and theoretical studies, they demonstrated high heterogeneity in mortality among populations and sites and, furthermore, that the spatial pattern of host mortality was highly aggregated in the environment with relatively few ‘hotspots’ where over 80% of host mortality occurred in less than 20% of sites.
Helminths and environmental change
The topic of how climate change is likely to impact helminth infections continues to be an important focus of research, and findings to date have been reviewed extensively (see, for example, Adekiya et al. Reference Adekiya, Aruleba, Oyinloye, Okosum and Kappo2019; Rocklöv et al. Reference Rocklöv, Ahlm, Scott, Humphries, Humphries, Scott and Vermund2021; Marcogliese Reference Marcogliese2023; Thompson Reference Thompson2023). Over and above the direct impact of shifts in temperature and rainfall on geographical range of hosts and survival of free-living stages (Marcogliese Reference Marcogliese2023), a more integrated approach to exploring the impacts of climate change is needed. A systematic review of the anticipated impact of climate change on thermal tolerance for human schistosomiasis revealed that a shift in the geographical distribution of the intermediate hosts to new areas within their thermal tolerance may be expected and that, given the co-evolved relationship of schistosomes and their snail host, the distribution of disease is likely to parallel the new snail distribution (Stensgaard et al. Reference Stensgaard, Vounatsou, Sengupta and Utzinger2019). However, if our understanding of schistosome transmission and distribution in the context of climate change is to improve, these authors highlighted the need to not only recognize the distinctness of the species of schistosome and the species of snail host but also consider environmental factors such as land cover, rainfall, and dams and other human infrastructures that also influence the distribution and density of the intermediate host.
Simulated climate change in outdoor mesocosms revealed that the presence of Maritrema novaezealandensis metacercariae strongly affected the intertidal amphipod community at 21°C but not at 17°C. This was likely driven, at least in part, by species-specific vulnerabilities among amphipods to infection-induced mortality at 21°C. At 25°C, infected amphipod communities were almost extirpated due to high infection-induced mortality (Mouritsen et al. Reference Mouritsen, Sorensen, Poulin and Fredensborg2018).
O’Brien et al. (Reference O’Brien, Bowman, Newar and Garroway2022) explored the potential implications of geographical range on helminth-induced host competition in a field study of squirrels in southern Ontario, Canada. The directly transmitted nematode Strongyloides robustus infects a range of squirrels and is considered more pathogenic in northern flying squirrels than southern flying squirrels. A northward range shift for southern flying squirrels may increase transmission of Strongyloides to northern flying squirrels, potentially reducing or excluding northern flying squirrels from habitats, especially if other sympatric squirrels are available to amplify Strongyloides transmission. Their data showed that, relative to northern flying squirrels, average prevalence across 30 woodlots was higher in red squirrels and lower in southern flying squirrels. Thus, the broader range of hosts for this nematode would potentially minimize the possible parasite-induced competitive exclusion of northern flying squirrels.
Large-scale agriculture necessitates changes to the landscape that have been shown to affect helminth transmission. A study in southern Ontario, Canada, explored the impact of corn production on the digenean community in snails. Ponds exposed to the herbicide atrazine had fewer snails and a lower total number of cercariae from six different genera (Koprivnikar et al. Reference Koprivnikar, Baker and Forbes2007). Associations with landscape features were species-specific. For example, Echinostoma trivolvis-infected snails were more common in ponds within 100 m of forests, a habitat that may be important for their waterfowl definitive host.
Helminths have also been affected by land-use change (White & Razgour Reference White and Razgour2020). The impact of deforestation and associated habitat fragmentation on nematodes in two small primates and two rodents from Madagascar was recently shown to depend on the type of nematode life cycle (Kiene et al. Reference Kiene, Andriatsitohaina, Ramsay, Rakotondravony, Strube and Radespiel2021). Although habitat fragmentation had no detectable impact on direct life cycle pinworms whose eggs remain around the rodent anus, pinworms were more common in mature forests that would provide tree holes for the gray mouse lemur Microcebus murinus. However, the risk of Strongyloides infection increased with the size of continuous forest, perhaps because the free-living generations of this nematode may be more sensitive to abiotic stresses such as UV exposure and desiccation at the forest edge. Similarly, nematodes transmitted through insects were less common at forest edges, perhaps indicating the vulnerability of their insect host to edge effects.
Concluding thoughts
It seems abundantly obvious that we are only at the tip of the iceberg in our understanding of host–helminth–environment interactions, but that this ‘tip’ is rich with inspiring, unimagined linkages and complexity. Three broad themes come to mind based on this compilation of ecological research related to helminths.
What about host regulation?
Anderson and May (Reference Anderson and May1978) and May and Anderson (Reference May and Anderson1978) demonstrated the theoretical ability of direct life cycle helminth parasites to regulate host abundance, and ecologists and parasitologists acknowledged this potential and included helminth infection among the list of forces that can regulate host populations. Anderson and May also made it clear that the likelihood and nature of this regulation was influenced not only by the requisite parasite-induced reduction of host survival or host fecundity but also by the proportion of hosts affected. Today, it is reasonable to ask whether our considerably advanced understanding of the complexity of the ‘host–helminth’ interaction changes our perspective on the likelihood that a specific helminth will regulate the abundance of a specific host population.
This review has demonstrated the depth and breadth of the interactions that helminths have with each other; with viruses, bacteria, protozoans, and fungi inside the host; and with viruses, bacteria, and microsporidia that use helminths as their host. It has highlighted the upstream and downstream interactions with the host population, community, and ecosystem. It has also reinforced the warning that ignoring helminths may mislead our understanding of host ecology (Frantz et al. Reference Frantz, Perga and Guillard2018; Timi & Poulin Reference Timi and Poulin2020). The take-home message is what seems to be a remarkable co-evolved balance that promotes good of the whole, where the complexity of interactions helps stabilize the entire biotic system. However, this balance is vulnerable to disruption, such as a change in temperature, a hurricane, removal of a top predator, introduction of a pesticide, provision of supplemental food to wildlife, or arrival of an invasive species. In light of the human-induced changes that the world is facing, it seems very likely that many co-evolved balances will be disrupted. Some helminths may increasingly affect, and regulate, host populations. Other helminths may have difficulty coping and decline in number, potentially releasing their host population from a subtle regulatory force with ramifications through the ecosystem. The more we come to understand specific systems and to determine where generalizations are possible, the better we will be able to project consequences.
Are we convinced that microbes are important to host–helminth dynamics?
It took a long time to convince ecologists that helminth parasites are a relevant, and important, component of ecosystems. Now, parasitologists need to be convinced that bacteria, viruses, simple eukaryotes, and fungi are an important partner influencing helminth population dynamics and host–helminth interactions. As noted by Salloum et al. (Reference Salloum, Jorge, Dheilly and Poulin2023), characterization of the diversity of ecological interactions among helminths and microbes continues to shift each day as more is understood about each relationship. Microbial ecologists are still learning about the elegant (yet delicate) balance in the microbial community within each host tissue; the influence of phyla, orders, families, genera, species and even strains of bacteria on one another and on the host both in a given tissue and systemically; and the influence of host factors on the microbial community. From the helminth perspective, we are still uncovering the consequences of helminths on the tissue-specific composition of the microbiome and mechanisms involved, such as release of helminth molecules with bactericidal properties. We are only beginning to recognize ways in which the ‘normal’ or the ‘altered’ microbial metabolome may benefit or harm the host. We know almost nothing about the ways in which helminth life history is shaped by the host microbiome or by microbes that use helminths as their host. Thus, it seems reasonable to suggest that we are far from drawing any generalizations about microbiome–helminth interactions. Projecting even 10 years into the future, it seems impossible to imagine where our understanding, or continued underappreciation, of microbial–helminth interactions will lie.
Is a One Health approach the way forward?
Thompson (Reference Thompson2023) has argued elegantly and persuasively for the critical importance of a One Health approach for advancing understanding, management, and control of zoonotic helminth infections. Based on the information collated in this review, it seems that we need the same sort of highly interdisciplinary approach that engages sociologists and behavioural scientists, environmental engineers and climate scientists, health practitioners and molecular biologists, microbiologists and bioinformaticians, wildlife biologists and limnologists, and mathematicians and ecologists, along with parasitologists if we are to unravel and repackage our conceptualization of host–helminth–environment interactions. Of the over 100 helminths mentioned in this review, multiple in-depth studies have been done on only a handful. The detailed insights that emerge when a system is studied from multiple scales and perspectives generates the excitement of discovery about the complexity of systems, yet each host–helminth system is a window into the spectrum of associations we have yet to uncover. In the context of the global challenges that we face and their implications for humans, domestic animals, and wild animals, using helminths as a window into the world may provide unexpected contributions of real value.
Financial support
Research is funded by a Discovery Grant (RGPIN/04563-2017) from the Natural Sciences and Engineering Research Council of Canada.
Competing interest
None.
Ethical standard
Not applicable.