Tilapia is a farmed species worldwide whose production reached 103·0 million tons in 2018(1). Genetically improved farmed tilapia (GIFT, Oreochromis niloticus) is a commercially vital farmed tilapia species due to its high adaptability and rapid growth rate(Reference Qiang, Yang and Wang2). However, the lack of freshwater resources and improved breeding costs limit the further development of the tilapia aquaculture industry. Globally, rising temperature causes sea level to rise, which intensifies salinisation in coastal areas. The freshwater shortage is an inevitable burden, and more than 1·2 million ha of land have been lost yearly to salinisation(3). It has been estimated that saline-alkaline water covers approximately 45·87 million ha in China alone, and salinity mainly ranged between 6 and 10 ‰(Reference Zhu4,Reference Yao, Lai and Zhou5) . Fortunately, saline-alkaline could be utilised to produce food through aquaculture(Reference Shivakumar, Seenappa and Venkatappa6). Song et al.(Reference Song, Zhao and Song7) found no significant negative effects of saline-alkaline water on the health of Nile tilapia and Qiang et al.(Reference Qiang, Wang and Kpundeh8) recommended GIFT tilapia juveniles be cultured at 7·8 ‰. Accordingly, as a new euryhaline species, GIFT has more potential as a new alternative for aquaculture in inland and low-salinity waters, which is meaningful for alleviating the pressure of freshwater aquaculture and meeting the increasing demand for global tilapia production (and hence animal protein). Furthermore, there were even reports that tilapia have been successfully raised in seawater with survival of 84·1–93·5 %(Reference El-Sayed9). However, relatively few studies have been devoted to the differences in nutritional adaptation and nutritional requirement of GIFT in different salinity environments.
Comprehensive and balanced nutrition is indispensable for the optimum growth performance and maintenance of fish(Reference Hoseini, Pérez-Jiménez and Costas10). Lysine is an essential amino acid in fish due to its lack of self-synthesis capacity(Reference Wilson11). The shortage of lysine was accompanied by poor growth performance and feed utilisation in several fish species(Reference Li, Wu and Dong12,Reference Mai, Zhang and Ai13) . Indeed, lysine supplementation could reduce the body fat of fish(Reference Burtle and Liu14) and lower dietary protein levels(Reference Cheng, Hardy and Usry15). Furthermore, the activation of the target of rapamycin (TOR) signalling pathway could be influenced by lysine(Reference Wang, Xu and Chi16) and may induce growth hormone and insulin-like growth factor 1(Reference Yang, Wang and Chi17) which is possibly related to the growth-promoting effects of lysine.
Salinity is an important external factor for fish growth, as fish need to maintain osmotic homoeostasis when encountering different salinity ranges(Reference Triantaphyllopoulos, Cartas and Miliou18). Previous researchers have evaluated that growth performance(Reference Cardona19,Reference Peterson, Martin-Robichaud and Berge20) , body composition(Reference Craig, Neill and Gatlin21) and blood parameters(Reference Mylonas, Pavlidis and Papandroulakis22) were closely associated with salinity. In a previous report, Rafael de Souza Romaneli et al.(Reference de Souza Romaneli, do Nascimento and Gous23) revealed that the appropriate dietary lysine requirement for GIFT juveniles was estimated at 1·93–1·95 % of the dry diet. However, changes in salinity might change the energy utilisation of fish, thereby affecting nutritional requirements, probably due to the reorientation of physiological processes under altered salinity conditions(Reference Tseng and Hwang24). For example, Wu et al.(Reference Wu, Liang and Hamunjo25) and Xu et al.(Reference Xu, Ren and Liang26) elucidated that salinity could change the optimal protein and methionine requirements of GIFT, respectively. However, only a few studies have been devoted to stimulating nutrient metabolism by salinity(Reference Da Silva Rocha, Gomes and Van Ngan27,Reference Zhou, Li and Wang28) . While lysine requirements and nutritional metabolism of GIFT under different salinities are still unclear. Therefore, this research extended the knowledge of the dietary lysine requirements at two cultured salinities and explored the effects of dietary lysine level (DLL) and salinity on the growth performance and nutrient metabolism of GIFT juveniles which might be established as a theoretical basis for the development of a particular feed for different salinity environments.
Materials and methods
The experimental protocol was followed by the Institutional Animal Care and Ethics Committee of Nanjing Agricultural University, Nanjing, China (Permit number: SYXK (Su) 20110036).
Experimental diet preparation
Six isonitrogenous and isoenergy purified diets with 16·4 kJ/g metabolisable energy and 33·0 % crude protein were formulated, containing graded lysine levels (1·34, 1·70, 2·03, 2·41, 2·72 and 3·04 % of dry diets) at two salinity levels (0 ‰, freshwater; 8 ‰, brackish water). The primary protein sources were soyabean meal and fish meal, while the primary sources of fat were soyabean oil and soya lecithin (online Supplementary Table S1). The whole-body amino acid profile of GIFT was simulated by supplementation of diets with crystalline l-amino acids (online Supplementary Table S2). Feed raw ingredients were shattered with a small feed grinder and then adequately blended with soyabean oil and water. The granulation process was performed by a pelletiser (F-26 (II), South China University of Technology, China) and then air-dried to approximately 10 % moisture. The pellet feed was sealed into bags and stored at −18°C before the trial initiation.
Experimental procedure
Juvenile GIFT was obtained from the Yixing Experiment Base of the Freshwater Fishery Research Center (Wuxi, China), and all fish were acclimated for 2 weeks under experimental conditions. Before starting the formal feeding experiments, fish were fasted for 24 h and weighed. Then, 720 lively and even size (initial weight 6·24 (sem 0·01) g) fish were randomly allocated into thirty-six recirculating water aquaculture tanks (180 l) with twenty fish per tank. The experimental fish were evenly divided into twelve groups with triplicate tanks, and 0 ‰ salinity and 8 ‰ salinity were adjusted to six groups as described in our previous study(Reference Xu, Ren and Liang26). Fish were fed to apparent visual satiation three times daily (07.00, 12.00 and 17.00 hours). During the 62-d trial, the water temperature (25 (s em 1)°C) and dissolved oxygen (6–7 mg/l) were monitored daily and maintained. Cultured salinity during the experiment was maintained by seawater crystals and measured daily using a salinity meter (ATAGO S-10E).
Sampling and chemical analysis
Sample collection
After an 8-week feeding trial, all fish were starved 24 h from the last feeding day, and then each barrel of fish was bulk-weighed and counted. Then, five fish were randomly selected, and two fish were stored in a –20°C freezer for the analysis of the whole-body composition. Simultaneously, samples of the liver were also collected from the other fish. Blood samples were obtained from the tail vein of five fish, and plasma was obtained by centrifugation (4000 × g , 15 min, 4°C). Finally, all samples of plasma and tissues were frozen at −80°C.
Biochemical analysis
Proximate analysis of raw materials, diets and whole-body composition were conducted using the established methods of AOAC(29). The principal detection equipment and methods are summarised in online Supplementary Table S3. The amino acid composition of ingredients was referred to our previous study(Reference Ren, Liao and Xie30).
Differential expression analyses of the genes were performed with real-time PCR analysis. First, the total RNA of the experimental fish was isolated from liver tissue using the RNAiso plus kit (Takara). Then, the quantity and purity of RNA were checked using spectrometric methods, and real-time PCR was performed with a 7500 real-time PCR machine using the One-Step SYBR® PrimeScript® Plus RT-PCR Kit. The specific primers for the target genes are shown in online Supplementary Table S4. β-actin was employed as a non-regulated reference gene, and no changes of β-actin gene expression were observed in our research. Finally, the expressions of target genes were calculated based on a mathematical model by Pfaffl(Reference Pfaffl31).
Protein extract was isolated using RIPA lysis buffer (50 mM Tris, 150 mM NaCl, 1 % TritonX-100, 0·1 % SDS, 1 % sodium deoxycholate, 1 mM EDTA). Protease and phosphatase inhibitors were added to all buffers before experiments. Western blot analysis was conducted according to the procedures as previously described(Reference Xu, Ren and Liang26). Briefly, protein concentrations were determined by the BCA method (BB-3401, BestBio). Tissue lysates were separated by SDS-PAGE gel and then washed with Tris Buffered Saline with Tween 20 (TBST) at room temperature. Primary antibodies were incubated overnight at 4°C and then incubated with the appropriate secondary antibody for 1 h. mTOR (#2972), phosphor-mTOR (#2971), 4E binding protein 1 (4E-BP1) (#9452) and phosphor-4E-BP1 (#9459) were obtained from Cell Signaling Technology Inc. β-actin (#ab0035) was obtained from Abways Technology. S6 kinase 1 (S6K1) (#1485–1-AP) was obtained from Proteintech Group. After incubation, TBST was used to wash the membrane. Specific proteins were visualised by Enhanced Chemiluminescence (ECL) luminescent solution. Band intensities were measured using a chemiluminescence imaging system (Clinx).
Statistical analysis
All data were presented as the means with their standard error of the means, and P < 0·05 was regarded as statistically significant. The effect of DLL and salinity and their interaction were analysed by two-way ANOVA, significant interaction between the main effects was observed for variables and a one-way ANOVA was then used as an aid for the interpretation of data. Then, Tukey’s multiple comparisons were assessed by SPSS 26.0 software (IBM SPSS). Meanwhile, after estimating the coefficients (R 2) of different mathematical models (broken-line regression model(Reference Robbins, Norton and Baker32) and quadratic regression model(Reference Zeitoun, Ullrey and Magee33)), the quadratic regression model was selected to estimate the optimum dietary lysine supplementation level in the practical diet.
Results
Growth performance
The growth and feed utilisation parameters of fish are presented in Table 1. Fish promptly accepted experimental diets, and the survival rate of GIFT was independent of DLL (1·34–3·04 %) and salinity. As shown, the specific growth rate (SGR) and weight gain rate were significantly affected by salinity and DLL, which increased significantly with the improvement of DLL up to 2·41 % and then decreased (P < 0·05). Moreover, higher weight gain rate and SGR were observed in 8 ‰ salinity level than 0 ‰ salinity level; however, DLL did not influence growth performance at 8 ‰ salinity. The lowest feed conversion ratio (FCR) was observed in 2·41 % DLL group, and a lower FCR was observed in 8 ‰ salinity level than 0 ‰ salinity level. From the perspective of lysine level, 8 ‰ salinity compared with 0 ‰ salinity had no significant influence on FCR (P ≥ 0·05). According to the quadratic regression model of SGR and FCR, the recommended range of lysine requirement of GIFT under different salinity was 2·03–2·20 % (0 ‰) and 2·20–2·41 % (8 ‰) (Fig. 1 and 2).
Table 1. Growth performance and feed utilisation of GIFT juvenile fed with diets containing six levels of dietary lysine at two salinities for 62 d
(Mean values with their standard errors of the mean)

GIFT, genetically improved farmed tilapia; WGR, weight gain rate; FCR, feed conversion ratio; SGR, specific growth rate; AIW, average initial weight; AFW, average final weight; SR, survival rate.
Values are mean of three replicates ± standard error of the mean. Means in the same column with different superscriptsabcd are significantly different (P < 0·05), superscriptsxy denote a significant P < 0·05 difference in values between 0 ‰ and 8 ‰ salinity as determined by t test.
* Means P < 0·05.
** Means P < 0·01, ‘ns’ means P ≥ 0·05. Means with the same letters or absence of letters indicate not significantly different between treatments.
WGR (%) = 100 × (final body weight (g) - initial body weight (g))/initial body weight (g).
FCR = dry feed fed (g)/(final body weight (g) - initial body/weight (g)).
SGR (% day−1) = 100 × ((In (final body weight (g)) – In (initial body weight (g)))/days).
SR (%) = 100 × (Number of fish survived/Total number of fish stocked).

Fig. 1. Quadratic regression model analysis of specific growth rate (SGR) against graded different levels of dietary lysine at () 0 ‰ and (
) 8 ‰ salinity.

Fig. 2. Quadratic regression model analysis of feed conversion ratio (FCR) against graded different levels of dietary lysine at () 0 ‰ and (
) 8 ‰ salinity.
Whole-body composition
The whole-body composition results are presented in Table 2. The whole-body moisture, crude protein, lipid and ash content of GIFT were significantly affected by salinity (P < 0·05), and crude protein and lipid were also affected by DLL. In addition, all composition indicators were significantly higher at 0 ‰ salinity than 8 ‰ salinity except for moisture (P < 0·05). The whole-body lipid content was higher in the treatment group fed 1·34 % DLL than other groups. Compared with lipid, the protein content was higher in the treatment groups with 2·03 and 2·41 % DLL, and the maximal protein content appeared at 2·03 % (0 ‰) and 2·41 % (8 ‰). Moreover, there was no significant difference among ash by DLL, and no interaction effects between DLL and salinity were detected in the whole-body composition.
Table 2. Whole-body composition of GIFT juvenile fed with diets containing six levels of dietary lysine at two salinities for 62 d
(Mean values with their standard errors of the mean)

GIFT, genetically improved farmed tilapia.
Values are mean of three replicates ± standard error of the means. Means in the same column with different superscriptsabcd are significantly different (P < 0·05), superscriptsxy denote a significant P < 0·05 difference in values between 0 and 8 ‰ salinity as determined by t test.
* Means P < 0·05.
** Means P < 0·01, ‘ns’ means P ≥ 0·05. Means with the same letters or absence of letters indicate not significantly different between treatments.
Plasma biochemical analysis
The results of glucose (GLU), triacylglycerol (TAG), total protein (TP) and total cholesterol (TC) in plasma are presented in Table 3. TAG concentration was not affected by salinity (P ≥ 0·05) but was higher in fish fed 2·41 % DLL than in the other groups. Meanwhile, there was no significant difference in the levels of TC and total protein between DLL (P ≥ 0·05), whereas salinity levels were positively related to TC and total protein levels. Glucose was not influenced significantly by DLL (P ≥ 0·05) but was inversely associated with increasing salinity (P < 0·05). Furthermore, no interaction was found between DLL and salinity (P ≥ 0·05).
Table 3. Plasma biochemical composition of GIFT tilapia juvenile fed with diets containing six levels of dietary lysine at two salinities for 62 d
(Mean values with their standard errors of the mean)

GIFT, genetically improved farmed tilapia; GLU, glucose; TP, total protein; TAG, triacylglycerol; TC, total cholesterol.
Values are mean of three replicates ± standard error of the means. Means in the same column with different superscriptsabcd are significantly different (P < 0·05), superscriptsxy denote a significant P < 0·05 difference in values between 0 and 8 ‰ salinity as determined by t test.
** Means P < 0·01, ‘ns’ means P ≥ 0·05. Means with the same letters or absence of letters indicate not significantly different between treatments.
Relative gene expressions
The results of TOR pathway-related genes in the liver of GIFT fed with different levels of lysine at two salinities are presented in Fig. 3. The expressions of TOR (Fig. 3(a)), ribosomal protein S6K1 (Fig. 3(b)) and eukaryotic initiation factor 4E-BP1 (Fig. 3(c)) were affected significantly by DLL and salinity. The TOR and S6K1 expression levels initially increased and then decreased with increasing DLL, reaching maximum mRNA level in both 2·41 % DLL and the maximum mRNA level of 4E-BP1 in 2·01 % DLL (0 ‰) and 2·41 % DLL (8 ‰). Additionally, a significantly higher expression of TOR was presented in the 8 ‰ salinity level than 0 ‰ salinity level (P < 0·05). Meanwhile, the expressions of S6K1 and 4E-BP1 had a similar trend with TOR. Furthermore, there were significant interactions among TOR, S6K1 and 4E-BP1 expression levels between DLL and salinity (P < 0·05). 8 ‰ salinity improved the protein and its phosphorylation protein levels of mTOR when compared with 0 ‰ salinity groups. The protein levels of SK61 and 4E-BP1 had the similar trends (Fig. 3(d)). Moreover, higher DLL groups (2·41 ‰, 2·72 ‰ and 3·04 ‰) have higher protein and its phosphorylation protein levels of mTOR in 8 ‰ salinity level.

Fig. 3. Relative gene expressions of TOR signalling pathway in the liver of GIFT juvenile fed with six dietary lysine levels at () 0 ‰ and (
) 8 ‰ salinity. (a) TOR, (b) S6K1, (c) 4E-BP1 and (d) Western blot analysis. Data are expressed as means with their standard error of the means, values with different superscripts are significantly different and 0‰ salinity labelled with lower case, 8‰ salinity labelled with upper case (P < 0.05). Means with the same letters or absence of letters indicate not significantly different between treatments. Asterisk (*) indicates a significant difference between 0 ‰ groups and 8 ‰ groups with P < 0·05. TOR, target of rapamycin; GIFT, genetically improved farmed tilapia; S6K, S6 kinase 1; 4E-BP1, 4E binding protein 1.
The relative expressions of glucose metabolism-related genes in the liver of GIFT are presented in Fig. 4. Glucokinase (GK) (Fig. 4(a)) and glucose-6-phosphatase (G6 Pase) (Fig. 4(b)) expression levels were significantly influenced by DLL, salinity and their interaction (P < 0·05). Moreover, the expressions of GK and G6 Pase were significantly higher at 0 ‰ salinity than 8 ‰ salinity (P < 0·05). Meanwhile, the expressions of GK and G6 Pase first increased and then reached a maximum at 2·41 % DLL. The expression level of glucose transporter 2 (GLUT2) (Fig. 4(c)) was not influenced by DLL at 0 ‰ salinity (P ≥ 0·05), while the GLUT2 expression level was significantly higher in the 2·41 % DLL group than the other groups at 8 ‰ salinity. Additionally, the expression level of GLUT2 was decreased with increasing salinity (P < 0·05), and there was no significant interaction between DLL and salinity (P < 0·05).

Fig. 4. Relative gene expressions of glycometabolism in the liver of GIFT juvenile fed with six dietary lysine levels at () 0 ‰ and (
) 8 ‰ salinity. (a) GK, (b) G6 Pase and (c) GLUT2. Data are expressed as means with their standard error of the means, values with different superscripts are significantly different and 0‰ salinity labelled with lower case, 8‰ salinity labelled with upper case (P < 0.05). Means with the same letters or absence of letters indicate not significantly different between treatments. Asterisk (*) indicates a significant difference between 0 ‰ groups and 8 ‰ groups with P < 0·05. GIFT, genetically improved farmed tilapia; GK, glucokinase; G6 Pase, glucose-6-phosphatase; GLUT2, glucose transporter 2.
Hepatic fatty acid synthase (FAS) (Fig. 5(a)) and sterol regulatory element-binding protein 1 (SREBP1) (Fig. 5(b)) expression levels were significantly affected by salinity and DLL. There were significantly high expressions of FAS and SREBP1 at 8 % salinity (P < 0·05). Moreover, the expression of FAS and SREBP1 was decreased with increasing DLL at 0 ‰ salinity, and then the minimum values were shown in 2·72 % and 3·04 % DLL groups, respectively. Similarly, the gene expression of FAS and SREBP1 showed a general downward trend in the 8 ‰ salinity groups except for 2·41 % DLL group. Moreover, the expression levels of FAS and SREBP1 exhibited a significant interaction between DLL and salinity (P < 0·05).
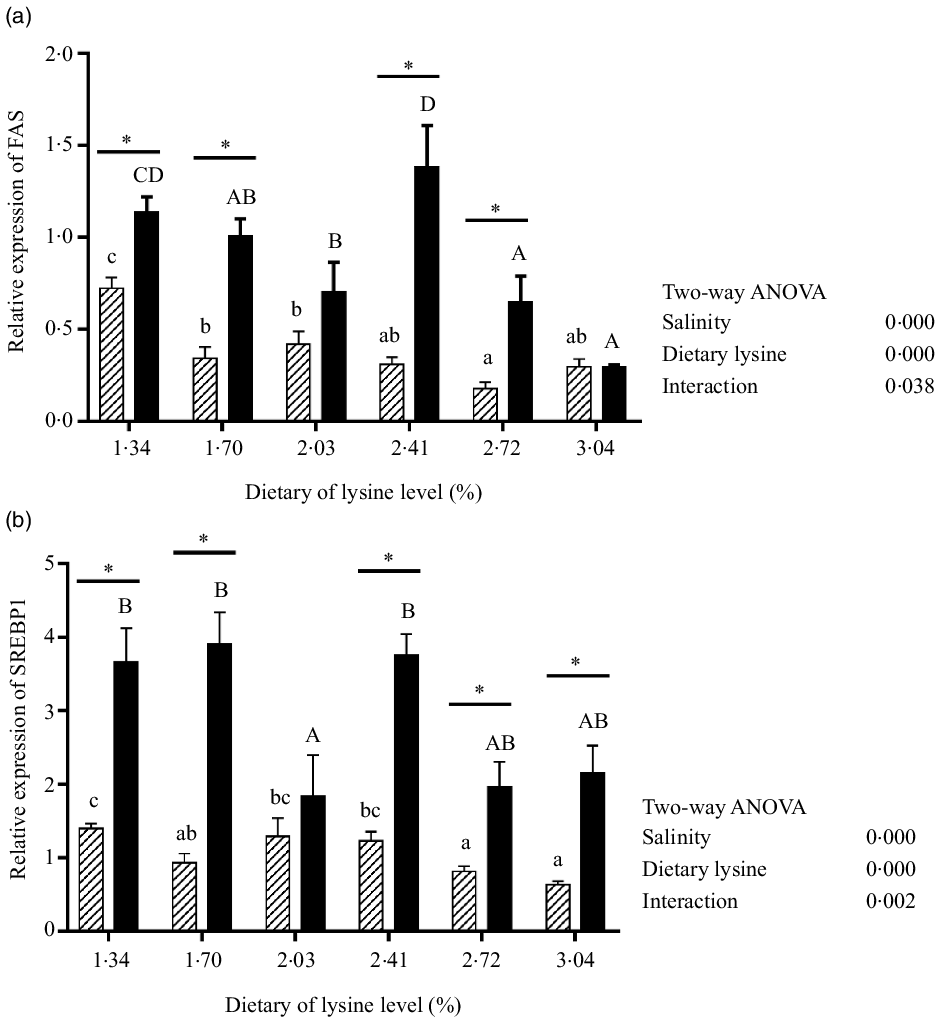
Fig. 5. Relative gene expressions of lipid synthesis in the liver of GIFT juvenile fed with six dietary lysine levels at () 0 ‰ and (
) 8 ‰ salinity. (a) FAS and (b) SREBP1. Data are expressed as means with their standard error of the means, values with different superscripts are significantly different and 0‰ salinity labelled with lower case, 8‰ salinity labelled with upper case (P < 0.05). Means with the same letters or absence of letters indicate not significantly different between treatments. Asterisk (*) indicates a significant difference between 0 ‰ groups and 8 ‰ groups with P < 0·05. GIFT, genetically improved farmed tilapia; FAS, fatty acid synthase; SREBP1, sterol regulatory element-binding protein.
Discussion
Lysine is commonly considered the first limiting essential amino acid in plant protein sources utilised in many fish foodstuffs, and lysine was the most abundant essential amino acid found in many fish species(Reference Kim and Lall34,Reference Wilson-Arop, Liang and Ge35) . The present results indicated that DLL and salinity had a remarkable effect on the growth performance and feed utilisation of GIFT, which were shown by SGR and FCR. Moreover, there was a marked decline in growth performance when DLL was greater than 2·41 % at 0 ‰ and 8 ‰ salinity. Therefore, this result showed that lysine played a crucial role in the normal growth of GIFT juveniles. Similarly, previous studies have demonstrated that the optimum growth performance of some fish, including Nile tilapia, grass carp (Ctenopharyngodon idellus) and turbot (Scophthalmus maximus), could be significantly affected by DLL(Reference de Souza Romaneli, do Nascimento and Gous23,Reference Wang, Liu and Tian36,Reference Zhou, Wu and Chi37) . Salinity was also an essential factor in fish growth because an improper salinity environment could change growth, macronutrient selection in fish and even cause death(Reference Cardona19,Reference Mylonas, Pavlidis and Papandroulakis22,Reference De Boeck, Vlaeminck and Van der Linden38) . In the present study, fish living in 8 ‰ salinity environment showed a relatively good growth performance. This may elucidate that GIFT might have been more inclined to accept brackish water. Compared with the hypertonic environment, fish in isotonic environments could store more energy to use for growth, and Qiang et al.(Reference Qiang, Wang and Kpundeh8) have found that 8 ‰ salinity near the isotonic state of GIFT. Similarly, our results parallel those reported by Likongwe et al.(Reference Likongwe, Stecko and Stauffer39) and González-Félix et al.(Reference González-Félix, Perez-Velazquez and Cañedo-Orihuela40), in which the highest growth rate of fish was observed at salinities close to the isosmotic point compared with freshwater and seawater, which might also be the explanation why GIFT juveniles have higher growth performance under 8 ‰ salinity.
Additionally, according to SGR and FCR, the quadratic regression analysis illustrated that the optimum requirement DLL of GIFT cultured in 8 ‰ salinity was higher than in freshwater, which was 2·31 %, 2·45 % (8 ‰), and 2·13 %, 2·15 % (0 ‰), respectively, possibly related to the requirement for more protein in fish at high salinity. For example, Larumbe-Morán et al.(Reference Larumbe-Morán, Hernández-Vergara and Olvera-Novoa41) and Wu et al.(Reference Wu, Liang and Hamunjo25) both found that GIFT juveniles might need more protein to satisfy the demand of growth in brackish water. Moreover, 8 ‰ salinity also significantly elevated the plasma content of total protein in this study. Generally, it was considered that the essential amino acid needs for protein accretion correspond to the amino acid content of tissue protein gain(Reference Kaushik and Seiliez42). Similarly, the present study indicated that a higher DLL of GIFT might be required to maintain growth needs at 8 ‰ salinity. However, no convincing evidence shows that protein requirements are affected by environmental factors (e.g. salinity)(Reference Lupatsch and Kissil43,44) , which still needs further investigation and confirmation.
In previous research, Prabu et al.(Reference Prabu, Felix and Uma45) reported that DLL did not influence the whole-body composition of juvenile GIFT. Nevertheless, in the present study, DLL significantly affected whole-body crude protein content, and in fish fed 2·03 %, which showed the maximum value at 0 ‰ salinity groups. Generally, protein deposition seemed to govern live weight gain in fish(Reference Dumas, De Lange and France46). This result indicated that proper lysine addition might promote protein deposition. Similar findings were also found in other fish, such as Nile tilapia(Reference de Souza Romaneli, do Nascimento and Gous23) and large yellow croaker (Pseudosciaena crocea)(Reference Zhang, Ai and Mai47). These variations in whole-body composition might include size, age, dietary constituents, feeding strategy, rearing environment and research design(Reference Kim, Kayes and Amundson48). Moreover, whole-body moisture and ash content were not affected by dietary lysine supplementation. Similar results were also previously observed in large yellow croaker and grass carp by Zhang et al.(Reference Zhang, Ai and Mai47) and Li et al.(Reference Li, Zhou and Mo49). In addition, a higher crude protein content of GIFT juveniles was observed at 8 ‰ salinity than 0 ‰ salinity, which was in agreement with the study of Wu et al.(Reference Wu, Liang and Hamunjo25) on GIFT. Increasing crude protein content in the brackish water might result from lower energy requirements for osmoregulation than in 0 ‰ salinity, indicating that the fish have more energy to use for protein deposition under 8 ‰ salinity. Of the major energy-yield nutrients, lipids have the greatest energy density. In the present study, an overall downward trend was detected in whole-body lipid content with increasing DLL, which corresponded well to the study of Mai et al.(Reference Mai, Zhang and Ai13), who found that the whole-body lipid content significantly decreased with increasing DLL in juvenile Japanese seabass. Furthermore, Deng et al.(Reference Deng, Dominy and Ju50) found that juvenile Pacific threadfin (Polydactylus sexfilis) showed higher lipid deposition in groups fed the lower DLL, and the authors hypothesised that deficiency in DLL impaired lipogenesis metabolism. Meanwhile, the whole-body lipid content in the present study was positively affected by 8 ‰ salinity. Correspondingly, Liu et al.(Reference Liu, Gao and Yu51) reported that American shad (Alosa sapidissima) at 7 ‰ salinity had a higher lipid content than freshwater. In summary, the lower DLL at the higher salinity might induce lipid deposition in the fish body.
TOR signalling is a key nutrient-sensing pathway that plays a crucial role in inducing protein synthesis through the regulation of 4EBP1 and S6K1 phosphorylation(Reference Wullschleger, Loewith and Hall52). Previous research has found that DLL could stimulate protein synthesis in fish by activating the TOR pathway such as largemouth bass (Micropterus salmoides)(Reference Burtle and Liu14) and juvenile turbot(Reference Kroeckel, Dietz and Schulz53). Similarly, significantly enhanced effects were observed in the expression of TOR pathway-related genes by DLL and showed a similar trend of whole-body protein content in juvenile GIFT, indicating that the balance of DLL could improve the protein synthesis of GIFT. Additionally, compared with 0 ‰ salinity, the expression of hepatic TOR pathway-related gene levels was higher in 8 ‰ salinity at the same lysine level, indicating that the protein synthesis metabolism of GIFT was more vigorous under 8 ‰ salinity. Wu et al.(Reference Wu, Liang and Hamunjo25) reported similar results in GIFT juveniles. The catabolism of protein provides an important energy source for fish to adapt to environments(Reference Cara, Moyano and Zambonino54). Hence, this phenomenon might be due to the priority of protein used as the main energy source of GIFT juveniles. Furthermore, 8 ‰ salinity improved the protein and its phosphorylation protein levels of mTOR, and the protein levels of SK61 and 4E-BP1 had the similar trends. This was consistent with measurements of gene expression. Xu et al.(Reference Xu, Ren and Liang26) found similar results in a study regarding salinity and methionine level. Interestingly, higher DLL (2·41 ‰, 2·72 ‰ and 3·04 ‰) had a relatively higher protein and phosphorylation levels in 8 ‰ salinity. The above aspects might also explain why the lysine requirement was higher in brackish water than in freshwater environments.
Glycolysis is the route of glucose catabolism in animals, including fish, and GK is a key rate-limiting enzyme in glucose utilisation(Reference Enes, Panserat and Kaushik55,Reference Massa, Gagliardino and Francini56) . In the present experiments, GIFT juveniles fed 2·41 % DLL showed the highest hepatic GK mRNA levels and increased significantly with increasing salinity, indicating that appropriate DLL could increase glycolysis in the liver. Additionally, the positive result was observed in grass carp by Huang et al.(Reference Huang, Liang and Ren57). GLUT2 specifically diffuses glucose and plays an essential role in the bidirectional transport of glucose to cells(Reference Granner and Pilkis58,Reference Terova, Rimoldi and Brambilla59) . In this study, the expression of GLUT2 was positively influenced by salinity but was not affected by DLL. Song et al.(Reference Song, Zhao and Song7) reported that a significantly higher expression of GLUT2 in Nile tilapia in brackish water (10 ‰) groups compared with freshwater. Likewise, GLUT2 gene expression paralleled changes in plasma glucose levels, which probably resulted from shifting a priority for glucose utilisation. Furthermore, gluconeogenesis is another vital pathway of glycometabolism in maintaining glucose homoeostasis, and the last and rate-limiting step of gluconeogenesis is regulated by G6 Pase(Reference Carneiro and Amaral60,Reference Panserat, Capilla and Gutierrez61) . The current study showed that 8 ‰ salinity significantly lowered the relative mRNA expression of G6 Pase. Similarly, G6 Pase enzyme activity changes were reported by Woo and Kelly(Reference Woo and Kelly62) in sea bream (Sparus sarba). Interestingly, we found that both gluconeogenesis and glycolytic processes were simultaneously higher at 0 ‰ salinity. The reason might be that nutrient metabolism has been reorganised to maintain glucose homoeostasis and proper energy supplementation(Reference Saha, Jyrwa and Das63). Correspondingly, we noticed that glucose levels were significantly lower at 8 ‰ salinity among the salinity treatments. Likewise, plasma glucose levels of juvenile turbot have been strongly affected by salinity(Reference Zeng, Lei and Ai64). Overall, these results implied that 0 ‰ salinity could boost carbohydrate use and increase carbohydrate utilisation. Few examples have clarified the effect of salinity on glucose metabolism-related genes, and further mechanistic research is needed to elucidate these.
The whole-body lipid contents of GIFT showed that DLL and salinity could alter lipid deposition in fish. SREBP1 is responsible for the biosynthesis of fatty acids by regulating the FAS gene(Reference Kim, Shin and Kim65), which is a key enzyme and factor-catalysed lipogenesis pathway(Reference Gondret, Ferré and Dugail66). The current results suggested that the expressions of SREBP1 and FAS showed a similar tendency with increasing DLL, indicating that low DLL could increase the rate of lipid anabolism and that crystalline lysine supplementation might contribute to lower lipid deposition in GIFT juveniles. Similar results were also observed in Atlantic salmon (Salmo salar) and rainbow trout (Oncorhynchus mykiss) by Espe et al.(Reference Espe, Lemme and Petri67) and Cheng et al.(Reference Cheng, Hardy and Usry68). Furthermore, related gene expression was consistent with the changing trend of whole-body crude lipid content of GIFT juveniles, which also supported our results. Harpaz(Reference Harpaz69) has reported that carnitine could promote the oxidation of fat and reduce body fat in European sea bass (Dicentrarchus labrax).
As the precursor substance of carnitine synthesis, lysine levels affected carnitine levels in the body(Reference Arslan70), which indicated that the increased lipid deposition in fish fed low-lysine diets might be due to reducing carnitine(Reference Rodehutscord, Becker and Pack71). Conversely, the up-regulated SREBP1 mRNA level was accompanied by up-regulated FAS mRNA levels when the salinity changed from 0 ‰ to 8 ‰. A similar phenomenon was also reported recently(Reference Xu, Ren and Liang26). Therefore, this finding indicated that 8 ‰ salinity might enhance liver lipogenesis of GIFT. Additionally, the highest plasma TAG and TC values of GIFT were observed in the 8 ‰ salinity group. Similarly, Mylonas et al.(Reference Mylonas, Pavlidis and Papandroulakis22) reported a reduction in TAG and TC levels when salinity changed from 4 ‰ salinity to 10 ‰ salinity in shi drum (Umbrina cirrosa). These results, coupled with a reduction in whole-body crude lipid content, further confirmed that 8 ‰ salinity could increase lipid deposition and lipogenic capacities.
Conclusions
In summary, according to the quadratic regression model of SGR and FCR, the recommended range of lysine requirement of GIFT under different salinity was 2·03–2·20 % (0 ‰) and 2·20–2·41 % (8 ‰). Furthermore, GIFT juveniles had higher growth performance at 8 ‰ salinity, and the TOR signalling pathway was further activated. Meanwhile, the gene expression levels of protein synthesis metabolism and lipid lipogenesis in the liver were more vigorous at 8 ‰ salinity. The GIFT juveniles contributed to enhanced carbohydrate utilisation at 0 ‰ salinity. However, lipids and proteins were deposited at 8 ‰ salinity.
Acknowledgements
This study was financially supported by the National Key Research and Development Program of China (2018YFD0900400) and Agriculture Research System of MOF and MARA (CARS-46).
M. R. and H. L. designed the study and reviewed the manuscript. M. S. carried out the experiments and wrote the manuscript. H. X., D. H. provided technical assistance. X. G. and J. Z. provided technical guidance. All authors contributed to the article and approved the submitted version.
The authors declare no conflict of interest.
Supplementary material
For supplementary materials referred to in this article, please visit https://doi.org/10.1017/S0007114522001763