Introduction
Treponema are motile, slender spiral-shaped bacteria that are members of the family Spirochaetaceae. Several Treponema species are present as part of the microbiota of humans and animals. These spirochaetes are, for the most part, cultivable, opportunistic pathogens that are associated with polymicrobial diseases (e.g. human gingivitis and chronic periodontitis, bovine foot and mammary dermatitis, etc.) [Reference Stamm, Trott, Radolf and Lukehart1, Reference Radolf, Pillay, Cox and Versalovic2]. Only a few Treponema are primary pathogens of humans. T. pallidum subspecies (subsp.) pallidum, endemicum, and pertenue are the agents of venereal syphilis, endemic syphilis, and yaws, respectively. Although the T. pallidum subsp. are very closely related on the basis of morphology, antigenic properties, and DNA sequence homology, they exhibit differences in geographical distribution, mode and severity of infection, and infectivity for laboratory animals. Thus far, none of the T. pallidum subsp., nor T. carateum, the agent of pinta, have been continuously cultivated in vitro [Reference Radolf, Pillay, Cox and Versalovic2, Reference Giancani and Lukehart3].
Epidemiology of syphilis
Globally, T. pallidum subsp. pallidum (T. pallidum) is the most significant pathogen of the genus Treponema. Infection is usually transmitted through contact with an active lesion of a sexual partner (acquired syphilis) or from an infected pregnant woman to her fetus (congenital syphilis). Syphilis can also be transmitted via blood transfusion, although this is rare today due to improved blood bank practices. An estimated 11–12 million new cases of adult syphilis occur each year, mostly in developing countries in sub-Saharan Africa and Southeast Asia where the disease is endemic [Reference Radolf, Pillay, Cox and Versalovic2, Reference Gottlieb4]. Additionally, between 700 000 and 1 500 000 cases of congenital syphilis occur with >50% of cases resulting in miscarriage or stillbirth [Reference Krüger and Malleyeck5]. Despite the availability of diagnostic tests and antibiotic therapy, syphilis has re-emerged in the USA, Canada, Europe, Russia, and China [Reference Stamm6, Reference Stamm and Mudrak7]. While the smaller syphilis outbreaks in the USA, Canada, and Europe have mostly involved men who have sex with men (MSM), the larger epidemics in Russia and more recently in China have mostly involved heterosexuals [Reference Stamm and Mudrak7, Reference Tucker8]. The resurgence of syphilis is a major concern to global public health, owing especially to the epidemiological and biological synergy of syphilis and human immunodeficiency virus (HIV) [Reference Gottlieb4, Reference Stamm and Mudrak7, Reference Ho and Lukehart9]. The ulcerative mucosal lesions of early syphilis increase the risk of acquisition and transmission of HIV infection, in part by acting as portals of entry or exit, respectively, for HIV.
Clinical manifestations of untreated adult syphilis
Syphilis is a chronic, systemic infection characterized by periods of active, clinical disease, which are interrupted by periods of latency [Reference Radolf, Pillay, Cox and Versalovic2, Reference Ho and Lukehart9]. Factors involved in syphilis pathogenesis are poorly understood. If left untreated, adult syphilis progresses in four overlapping stages based on clinical findings (i.e. primary, secondary, latent, tertiary) [Reference Ho and Lukehart9, Reference Sparling and Holmes10]. The primary and secondary stages are the most infectious.
Primary syphilis occurs after an incubation period of about 2–6 weeks. This stage is characterized by the presence of an indurated ulcer (chancre) at the site of inoculation (i.e. typically the genital, anal, or oral mucosa) and is accompanied by regional lymphadenopathy. The chancre heals spontaneously in a few weeks due to the phagocytosis of treponemes by activated macrophages, which is enhanced by low levels of opsonic antibodies. Despite destruction of the majority of the treponemes, some escape to cause chronic infection.
Secondary syphilis results from the multiplication and haematogenous dissemination of the surviving treponemes. This stage occurs simultaneously with, or up to 6 months after, healing of the chancre. It is characterized by malaise, low-grade fever, headache, generalized lymphadenopathy, a localized or generalized skin rash with lesions on the palms and soles, mucous patches in the oral cavity or genital tract, condylomata lata (wart-like lesions), and patchy alopecia. Secondary syphilis lasts for weeks or months with relapses occurring in about one-quarter of untreated patients.
The latent stage of syphilis is the period from disappearance of secondary stage manifestations to the occurrence of the tertiary stage. Latent syphilis is divided into early latent (i.e. occurring within 1 year of infection) and late latent (i.e. occurring after 1 year of infection). While clinical symptoms are not apparent during latency, most serological tests are positive for antibodies to T. pallidum [Reference Seña, White and Sparling11]. About two-thirds of untreated syphilis patients remain in the latent stage for life.
Tertiary (i.e. late) syphilis usually presents within several years to decades after the initiation of latency, but is rare today due to curative antibiotic treatment given for early syphilis or coincidentally for unrelated infections. Tertiary syphilis can affect almost any tissue. Manifestations include cardiovascular syphilis, neurosyphilis, and gummatous syphilis, all of which may co-exist. Gummatous syphilis involves granulomatous lesions, mostly in skin and bone, which occur singly or multiply and vary in size from microscopic to large masses. Transmission of syphilis during the tertiary stage is unlikely, presumably due to the low number of treponemes that are present.
Diagnosis of syphilis
Direct detection
Because sustained in vitro cultivation of T. pallidum is not possible and animal (i.e. rabbit) inoculation is time consuming and expensive, laboratory diagnosis of syphilis is usually based on direct detection of T. pallidum and/or serology. Direct detection of T. pallidum by dark-field microscopy has remained the gold standard for diagnosis of syphilis in patients presenting with lesions of early, active syphilis (i.e. primary and secondary stage). Dark-field microscopy has a sensitivity of 39–81% with a specificity of 82–100% [Reference Radolf, Pillay, Cox and Versalovic2]. However, the accuracy of this technique is affected by the age and condition of the lesion and the expertise of the microscopist. Additionally, dark-field microscopy cannot be used for oral or anal lesions since it cannot discriminate T. pallidum from the cultivable Treponema species that are present at these sites. The use of a direct fluorescent antibody (DFA) test employing monoclonal antibody circumvents this problem since only T. pallidum is stained. However, the DFA test cannot discriminate between the T. pallidum subsp. due to the presence of cross-reactive antigens. Nucleic-acid amplification tests (NAATs), such as the polymerase chain reaction (PCR) and reverse transcriptase–PCR (RT–PCR) tests, have greatly improved the sensitivity and specificity of direct detection methods for T. pallidum, but are mainly used in reference or research laboratories [Reference Radolf, Pillay, Cox and Versalovic2].
Serological tests
Serological tests for syphilis (STS) are divided into two types: non-treponemal and treponemal [Reference Radolf, Pillay, Cox and Versalovic2, Reference Seña, White and Sparling11]. It is important to note that infection with the other Treponema subsp. (i.e. endemicum and pertenue) also produces a positive reaction in STS. Non-treponemal STS include the Venereal Disease Research Laboratory (VDRL), rapid plasma reagin (RPR), and toluidine red unheated serum test (TRUST). Serum IgM or IgG antibodies to lipoidal material released from damaged host cells or putatively associated with T. pallidum are detected by flocculation of an antigen suspension that contains cardiolipin. Although non-treponemal STS are widely available, rapid, and relatively inexpensive, their use as qualitative screening tests is complicated by a lack of sensitivity in primary and tertiary syphilis and by false-positive test results.
Treponemal STS use native or recombinant T. pallidum antigens and are based on the detection of serum IgM or IgG antibodies to these components [Reference Radolf, Pillay, Cox and Versalovic2, Reference Seña, White and Sparling11]. These tests include the T. pallidum particle agglutination assay (TPPA), T. pallidum haemagglutination assay (TPHA), fluorescent treponemal antibody absorption assay (FTA-abs), various enzyme immunoassays (EIAs), and rapid immunochromatographic strip tests. Generally, treponemal STS are more sensitive and specific than non-treponemal STS for all stages of syphilis and false-positive test results are less common.
US guidelines for serological diagnosis of syphilis recommend the use of a combination of one non-treponemal STS and one treponemal STS [Reference Radolf, Pillay, Cox and Versalovic2, Reference Seña, White and Sparling11]. The use of only one type of STS is insufficient for diagnosis since each type of STS has limitations, including the possibility of false-positive test results in persons who are not infected. The traditional algorithm for syphilis diagnosis is based on the use of a non-treponemal STS for screening followed by the use of a treponemal STS for confirmation of a positive screening test result. However, some US clinical laboratories and blood banks have reversed this order and are now using treponemal STS for screening and non-treponemal STS for confirmation of a positive treponemal test result [Reference Seña, White and Sparling11]. Guidelines for the UK recommend screening with a treponemal STS (i.e. EIA) or a combination of non-treponemal and treponemal STS, using an IgM-specific EIA when early primary syphilis is suspected [Reference Kingston12]. A positive screening test result is confirmed with a different treponemal STS than the one that was used for screening.
Use of STS for monitoring effectiveness of syphilis treatment
Treponemal STS cannot be used to assess the response of patients to antibiotic treatment since patients with a positive test result usually continue to remain positive for the remainder of their lives, regardless of treatment. Thus, non-treponemal STS are used as quantitative tests to monitor the effectiveness of antibiotic treatment [Reference Radolf, Pillay, Cox and Versalovic2, Reference Ghanem and Workowski13, Reference Seña14]. The non-treponemal STS titre of serum usually declines after effective antibiotic treatment of the patient and may become non-reactive with time. Lack of a fourfold decline in serological titre within 6–12 months after therapy for early syphilis (i.e. primary, secondary, or early latent) or 12–24 months for late latent syphilis may be due to treatment failure or possibly re-infection. Serological titres may decline more slowly in HIV-infected patients. Fifteen to twenty per cent of syphilis patients remain ‘serofast’ (i.e. do not completely revert to a nonreactive test result) after appropriate therapy regardless of HIV infection. The clinical significance of this is unknown, but follow-up is recommended for such patients [Reference Seña14].
Antibiotic treatment of syphilis
Because there is no vaccine to prevent infection with T. pallidum, antibiotic treatment is a key component of syphilis control programmes. The U.S. Centers for Disease Control and Prevention (CDC) 2010 guidelines for treatment of sexually transmitted diseases [Reference Workowski and Berman15] and the UK 2008 guidelines for management of syphilis [Reference Kingston12] recommend penicillin G, administered parenterally, as the first-line drug for treatment of incubating syphilis and for all stages of syphilis (Table 1). The penicillin preparation (i.e. benzathine, aqueous procaine or aqueous crystalline), dose, mode of delivery, and length of treatment are dependent upon the stage and clinical manifestations of syphilis, as well as other management considerations (e.g. pregnancy, HIV status, patient's age). Use of the appropriate penicillin preparation is critical since T. pallidum can reside in sequestered sites (e.g. central nervous system, aqueous humor, synovial fluid) where treponemicidal levels of penicillin are not easily achievable. Because there are no proven alternatives to penicillin for treatment of pregnant women with syphilis, those who are allergic to penicillin must be desensitized and then treated with penicillin.
Table 1. Antibiotic treatment of syphilis in HIV-negative adults*
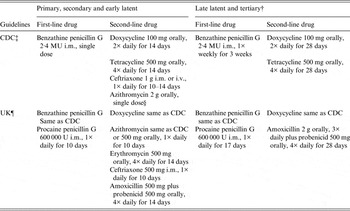
i.m., Intramuscular; i.v., intravenous; MU, million units.
* Recommended treatments for HIV-negative adults who are non-pregnant. For treatment of children, pregnant women, HIV-infected patients and patients with neurosyphilis, see notes ‡ and ¶ below.
† Tertiary syphilis refers to gummatous and cardiovascular syphilis. Treatment is the same for latent syphilis of unknown duration.
‡ See Workowski & Berman [Reference Workowski and Berman15].
§ Due to possible resistance, azithromycin must only be used when treatment with penicillin or doxycycline is not feasible. Azithromycin must not be used in MSM or pregnant women.
¶ See Kingston et al. [Reference Kingston12].
Data to support the use of second-line drugs for syphilis treatment are limited [Reference Ghanem and Workowski13]. According to CDC guidelines, men and non-pregnant women with early syphilis who are allergic to penicillin may be treated with doxycycline (100 mg orally, twice daily for 14 days); tetracycline (500 mg orally, four times daily for 14 days) or ceftriaxone [1 g intramuscularly (i.m.) or intravenously (i.v.), once daily for 10–14 days] [Reference Workowski and Berman15] (Table 1). Men and non-pregnant women with late latent and tertiary (i.e. gummatous and cardiovascular) syphilis who are allergic to penicillin or refuse parenteral treatment may be treated with doxycycline or tetracycline at the dosages used for early syphilis, but extended to 28 days [Reference Workowski and Berman15] (Table 1).
UK guidelines for the use of second-line drugs for treatment of early syphilis are similar to CDC guidelines except that (1) tetracycline is no longer recommended due to availability of doxycycline; (2) ceftriaxone is given as 500 mg i.m. once daily for 10 days; and (3) amoxicillin (500 mg with 500 mg probenicid given orally, four times daily for 14 days) is an additional alternative [Reference Kingston12] (Table 1). Although both the CDC and UK guidelines include azithromycin as a second-line drug for early syphilis, treatment failures have been documented in the USA due to emergence of macrolide-resistant T. pallidum [Reference Stamm6, Reference Ghanem and Workowski13, Reference Katz and Klausner16, Reference Stamm and Embers17]. Thus, azithromycin must be used with utmost caution and only when treatment with penicillin or doxycycline is not feasible. Azithromycin, should not be used to treat pregnant women or MSM [Reference Workowski and Berman15]. UK guidelines for second-line drugs for treatment of men and non-pregnant women with late latent and tertiary syphilis include doxycycline (same as CDC guidelines) and amoxicillin (2 g orally, three times daily plus 500 mg probenecid orally, four times daily for 28 days) [Reference Kingston12] (Table 1). Close follow-up of patients receiving second-line therapy is absolutely essential since treatment failure can extend the ‘window of opportunity’ for transmission of syphilis. (For guidelines for treatment of syphilis in children, pregnant women, HIV-infected patients and patients with neurosyphilis, see [Reference Kingston12, Reference Workowski and Berman15].)
Macrolide-resistant T. pallidum
The need for deep i.m. injection of benzathine penicillin, which is painful, and the possibility of a severe allergic reaction to penicillin has led some clinicians to use second-line oral antibiotics, including macrolides (e.g. erythromycin, azithromycin, clarithromycin, spiromycin) and tetracyclines (e.g. tetracycline, doxycycline) as first-line drugs for treatment of early syphilis. This usage is inconsistent with the CDC and UK treatment guidelines and is now further complicated by the emergence of T. pallidum with clinically significant, high-level resistance to macrolides [Reference Stamm6, Reference Katz and Klausner16–Reference Li20]. Macrolides are bacteriostatic antibiotics that inhibit protein synthesis by binding reversibly to the 23S rRNA of the 50S ribosomal subunit. Failure of erythromycin therapy for syphilis was first reported in 1964 and again in 1976 for pregnant women who subsequently gave birth to infants with congenital syphilis. However, it is unknown if these treatment failures were due to erythromycin-resistant T. pallidum since macrolides may not efficiently cross the placental barrier. In 1977, a T. pallidum strain (i.e. Street strain 14) was isolated by inoculation of rabbits with material from a US patient with secondary syphilis who had failed long-term erythromycin therapy. Stamm and co-workers later demonstrated, using an in vitro assay to evaluate the effect of antibiotics on treponemal protein synthesis, that Street strain 14 is resistant to high levels of erythromycin and cross-resistant to azithromycin [Reference Stamm6, Reference Stamm and Embers17]. Studies in the rabbit model of syphilis showed that the macrolide-resistant phenotype of Street strain 14 is highly stable, despite multiple passages in the absence of antibiotic pressure [Reference Stamm6, Reference Marra21].
In bacteria with only one or two 23S rRNA genes, macrolide resistance is often due to mutation or methylation of the peptidyl transferase region in domain V of 23S rRNA [Reference Stamm6, Reference Stamm and Embers17]. Using a combination of PCR amplification and DNA sequencing, Stamm & Bergen [Reference Stamm and Bergen22] showed that an adenine (A) to guanine (G) transition at the position cognate to A2058 in the E. coli 23S rRNA gene is present in both copies of the Street strain 14 23S rRNA gene. The A2058G mutation is not present in the Nichols type strain, which was isolated in 1912 and is sensitive to macrolides. Point mutations at position 2058 are present in the 23S rRNA genes of several macrolide-resistant bacteria including some other spirochaetes (e.g. Brachyspira spp. and T. denticola) [Reference Stamm6, Reference Stamm and Embers17].
Despite documented erythromycin resistance in T. pallidum Street strain 14, there was considerable enthusiasm early on for the use of azithromycin for treatment of syphilis, particularly for resource-limited settings where the burden of syphilis is high and the use of injectable drugs is problematic. Unlike erythromycin, azithromycin can be given as a single 2 g dose and has a long tissue half-life. Clinical efficacy of azithromycin for syphilis treatment was demonstrated in non-randomized studies and in randomized controlled trials in the USA, Africa, China, and Madagascar that compared cure rates for this drug and penicillin [Reference Stamm6, Reference Ghanem and Workowski13, Reference Kiddugavu23–Reference Hook26]. Azithromycin was used for syphilis treatment in Uganda (mid 1990s), the USA (1999 and 2000), and Canada (2000). However, during 2002–2003, treatment failures were observed in eight patients in San Francisco, California [Reference Katz and Klausner16]. Molecular analysis of T. pallidum in clinical specimens from two of these patients revealed a 23S rRNA gene mutation (A2058G) identical to that of Street strain 14. Retrospective analysis of clinical specimens from three US cities (Baltimore, San Francisco, Seattle) and Vancouver, Canada showed that while the prevalence of the mutation varied between the sites, it increased significantly over time within these sites [Reference Stamm6, Reference Katz and Klausner16, Reference Stamm and Embers17, Reference Lukehart27]. For example, the presence of T. pallidum with the A2058G mutation increased from 4% (2002) to 76·5% (2005) in specimens from syphilis patients in San Francisco and from 9% (2002) to 56% (2005) in specimens from syphilis patients in Seattle [Reference Katz and Klausner16, Reference Marra21].
Macrolide-resistant T. pallidum with the A2058G mutation is now present in several areas of the USA, Canada, Europe, and China and has been reported recently in Sydney, Australia [Reference Ghanem and Workowski13, Reference Katz and Klausner16–Reference Li20, Reference Chen28–Reference Read30]. Most syphilis outbreaks involve multiple T. pallidum strains in which macrolide resistance has emerged independently. Recent macrolide use for unrelated infections (e.g. skin, genital, or oral) is significantly associated with the increased prevalence of macrolide-resistant T. pallidum [Reference Grimes18, Reference Marra21, Reference Chen28]. The persistence of low levels of azithromycin in tissues for weeks after treatment for unrelated infections is likely to allow for selection of resistant mutants of T. pallidum that arise de novo. If treated inappropriately with azithromycin as a first-line drug, a patient who is infected with a resistant strain will remain infectious and may be capable of transmitting azithromycin-resistant T. pallidum. Based on studies in the rabbit model of syphilis, the A2058G mutation is stable in the absence of selective pressure [Reference Stamm6, Reference Marra21]. Thus, azithromycin-resistant T. pallidum strains may be able to persist in sexual networks [19]. Interestingly, macrolide-resistant T. pallidum appears to be uncommon in some countries (e.g. Madagascar, South Africa, Lesotho, Taiwan) where in certain cases the use of macrolides has been more limited [Reference Van31–Reference Wu34].
Until recently, the A2058G mutation was the only documented mutation associated with T. pallidum macrolide resistance. This mutation confers resistance to 14-member (e.g. erythromycin, roxithryomycin, clarithromycin) and 15-member (e.g. azithromycin) lactone ring macrolides, but not to 16-member lactone ring macrolides (e.g. spiramycin, tylosin), possibly due to differential binding of these drugs to Street strain 14 23S rRNA [Reference Stamm6, Reference Stamm and Embers17]. However, Matějková et al. [Reference Matějková35] identified a new mutation, A2059G, in both copies of the 23S rRNA gene of T. pallidum that was present in clinical specimens of a Czech Republic syphilis patient who had failed spiramycin therapy. The A2059G mutation is associated with resistance to 14-, 15-, and 16-member lactone ring macrolides in a number of bacteria and is presumably responsible for spiramycin resistance in T. pallidum. The prevalence of the A2059G or A2058G mutation in T. pallidum from syphilis patients in the Czech Republic was about 18% for either mutation. Because there was no direct epidemiological relationship among patients infected by T. pallidum with the A2059G mutation, it is unlikely that these patients were infected with a single, macrolide-resistant strain of T. pallidum.
Initially, there were no reports outside of the Czech Republic of T. pallidum with the A2059G mutation. However, Tipple et al. [Reference Tipple, McClure and Taylor36] identified T. pallidum with the A2059G mutation in one of 18 syphilis patients in a London, UK sexual health centre. Eleven of the remaining patients were infected by T. pallidum with the A2058G mutation. Recently, T. pallidum with the A2059G mutation was detected in syphilis patients in the USA and China [Reference Grimes18, Reference Li20, Reference Chen37]. These results indicate that molecular methods to assess T. pallidum macrolide resistance must be capable of identifying point mutations at both positions (i.e. 2058 and 2059) in the 23S rRNA genes. Chen et al. [Reference Chen37] has reported a TaqMan-based real-time triplex PCR assay that allows for rapid, detection of both point mutations. Thus far, T. pallidum with simultaneous mutations at positions 2058 and 2059 has not been described.
Conclusion
Syphilis continues to present challenges to global public health, particularly because it can increase the risk of acquiring and transmitting HIV infection. Current regimens for treatment of syphilis were developed before randomized controlled trials became standard. Differences in interpreting earlier studies have resulted in some variability in national guidelines. However, penicillin, which was first used for treatment of syphilis in the 1940s, has remained the recommended first-line drug for treatment of all stages of syphilis. Unlike most other bacteria, T. pallidum has remained highly sensitive to penicillin. Emergence of penicillin resistance appears unlikely since it would presumably require multi-step mutational changes in a bacterium that lacks horizontal gene transfer mechanisms [Reference Stamm6, Reference Stamm and Embers17, Reference Fraser38]. Nevertheless, T. pallidum has a capacity for endogenous genetic change as demonstrated by the emergence of clinically significant, high-level macrolide resistance due to point mutations (i.e. A2058G or A2059G) in its 23S rRNA genes. Although these point mutations are now frequently present in T. pallidum obtained from patients at several different geographical locations worldwide, their effect on the prevalence of syphilis is currently unknown. However, it is reasonable to surmise that macrolide treatment failure, which allows for persistence of infectious treponemes in host tissues, enables the spread of syphilis by extending the ‘window of opportunity’ for transmission.
At this juncture, macrolide resistance is the only clinically significant resistance that has been documented in T. pallidum. Owing to concerns regarding macrolide resistance, the use of doxycycline, as a second-line drug, is likely to increase for treatment of early adult syphilis in non-pregnant patients who are allergic to penicillin or who refuse parenteral treatment. Poor compliance to doxycycline treatment could result in subtherapeutic concentrations of this antibiotic in patient tissues, potentially acting as a selective pressure for emergence of doxycycline-resistant mutants. Single point mutations in the 16S rRNA gene of various bacterial species (i.e. at residues cognate to positions 965–967 or 1058 in the E. coli 16S rDNA) have been shown to confer resistance to doxycycline. Thus, point mutations at the relevant position in one or both copies of the T. pallidum 16S rRNA gene could result in doxycycline resistance [Reference Stamm6, Reference Stamm and Embers17].
Addressing the resurgence of syphilis and the emergence of macrolide-resistant T. pallidum will require effort on several fronts. First, because penicillin administration can be problematic, especially in developing countries, there is a need for new, single-dose, oral antibiotics to ensure patient compliance during treatment. Novel macrolides that show activity against bacteria that harbour 23S rDNA mutations merit investigation in the rabbit model of syphilis since it is possible that they could circumvent the current problem of azithromycin-resistant T. pallidum. Second, the availability of rapid point-of-care (POC) diagnostic tests and access to testing needs to be improved, especially in resource-poor settings [Reference Mabey39]. A dual POC test is commercially available that acts as both a screening and confirmatory test for syphilis and can use finger stick blood, serum or plasma under field conditions [Dual Path Platform (DPP) Syphilis Screen & Confirm test, Chembio Diagnostic Systems Inc., USA] [Reference Castro40]. The DPP test has the potential to interrupt transmission of syphilis by helping clinicians make prompt decisions concerning treatment. This is particularly important for patients who may not return for laboratory-based test results. Third, surveillance for detection of macrolide-resistant T. pallidum should be expanded to facilitate a better understanding of the prevalence and geographical distribution of this problem. The use of an enhanced molecular typing system for T. pallidum could aid in tracking movement of resistant strains [Reference Marra41]. Fourth, efforts to develop a vaccine to prevent syphilis, and thus limit dependence on antibiotics, should be renewed. The availability of novel methods to modulate the innate and adaptive immune response, coupled with an improved understanding of T. pallidum proteogenomics, has stimulated new approaches to syphilis vaccine development [Reference Stamm and Drapp42, Reference Cameron and Lukehart43]. Advances in syphilis diagnosis, treatment, molecular surveillance and vaccines, in combination with a rapid, multi-faceted strategy for outbreak containment, would significantly benefit global public health by accelerating syphilis control efforts.
DECLARATION OF INTEREST
None.