1. Introduction
Humanity's exponential rise in energy use and mobility, among other socio-economic trends since the 1950s, has led to similar rates of exponential anthropogenic impacts on Earth Systems (Hibbard et al., Reference Hibbard, Crutzen, Lambin, Liverman, Mantua, McNeill, Messerli, Steffen, Constanza, Graumlich and Steffen2006) such as carbon dioxide and methane atmospheric concentrations, surface temperatures, and forest loss (Ripple et al., Reference Ripple, Wolf, Newsome, Gregg, Lenton, Palomo, Eikelboom, Law, Huq, Duffy and Rockström2021; Steffen et al., Reference Steffen, Broadgate, Deutsch, Gaffney and Ludwig2015). This acceleration of both socio-economic trends and environmental degradation, and humanity's study of it (Rockström et al., Reference Rockström, Steffen, Noone, Persson, Chapin, Lambin, Lenton, Scheffer, Folke, Schellnhuber, Nykvist, de Wit, Hughes, van der Leeuw, Rodhe, Sörlin, Snyder, Costanza, Svedin, Falkenmark, Karlberg, Corell, Fabry, Hansen, Walker, Liverman, Richardson, Crutzen and Foley2009), marks humanity's awareness of our ability to undermine Earth's capacity to support human development (Raworth, Reference Raworth2017b), which demands societal transformation to avoid the worst impacts to humanity (Newell et al., Reference Newell, Daley and Twena2021).
These exponential socio-economic trends and associated environmental impacts have not been equally distributed, where the richest portions of the human population are causing a disproportionate amount of the impacts (e.g. Malm & Hornborg, Reference Malm and Hornborg2014). This is reflected in the updated ‘Great Acceleration’ graphs, where in 2010, OECD populations comprised only 18% of the global population and yet this population was responsible for 74% of global GDP (Steffen et al., Reference Steffen, Broadgate, Deutsch, Gaffney and Ludwig2015). Such inequity is occurring not just between countries but also within them, both of which can lead to significant social harm (Wilkinson & Pickett, Reference Wilkinson and Pickett2009). Economic inequalities also translate to unequal distribution of energy use and greenhouse gas (GHG) emissions, with a minor part of humanity being responsible for the major share of impacts (Hubacek et al., Reference Hubacek, Baiocchi, Feng, Castillo, Sun and Xue2017; Kartha et al., Reference Kartha, Kemp-Benedict, Ghosh, Nazareth and Gore2020).
Bringing together these environmental and social issues presents its own challenges, however, due to their interdependencies. The impact of ecological stress, for example, climate change, can actually exacerbate social problems, such as poverty (Leichenko & Silva, Reference Leichenko and Silva2014). This can occur vice versa as well, where if environmental policies are established based on analyses that fail to capture the multi-faceted complexity between environmental and social issues, these policies could potentially stagnate social equality (Raworth, Reference Raworth2017a). Lamb et al.'s (Reference Kartha, Kemp-Benedict, Ghosh, Nazareth and Gore2020) review of ex-post social outcomes associated with different implemented environmental policies highlights this potential, where the study found that environmental policies often fell short in delivering substantive positive social outcomes. Conceptualizing these interdependencies, Raworth's (Reference Raworth2012, Reference Raworth2017a) ‘A safe and just operating space for humanity’ detailed in her book Doughnut Economics (Raworth, Reference Raworth2017b) and the sustainable consumption corridor (SCC) frameworks (Fuchs et al., Reference Fuchs, Sahakian, Gumbert, Giulio, Maniates, Lorek and Graf2021) were established in an effort to bridge these environmental and social issues to describe a space where humanity can live good and healthy lives without an escalated level of consumption that endangers intergenerational sustainability (Brand-Correa et al., Reference Brand-Correa, Mattioli, Lamb and Steinberger2020). Both frameworks incorporate the conceptual frameworks of ecological ceilings (ECs), often based on the planetary boundaries (PBs) framework, and social foundations (SFs), where sustainability is visualized either as a doughnut or corridor, in which the SF describes the minimum social provisions that should be achieved to lead a good and dignified life, but that the resource use required to meet humanity's needs and wants don't surpass Earth's carrying capacity. The space between the defined EC and SFs is the space where sustainability can be said to have been achieved, in the sense of ‘strong sustainability’ (Ekins et al., Reference Ekins, Simon, Deutsch, Folke and Groot2003; Wiedmann et al., Reference Wiedmann, Lenzen, Keyßer and Steinberger2020).
There have been works that mobilize Raworth's doughnut framework and related concepts at a regional level (Dearing et al., Reference Dearing, Wang, Zhang, Dyke, Haberl, Hossain, Langdon, Lenton, Raworth, Brown and Carstensen2014), from the UK (Sayers & Trebeck, Reference Sayers and Trebeck2015) to South Africa (Cole, Reference Cole2015), as well as at a city level (e.g. City Think Space, 2012; Raworth et al., Reference Raworth, Krestyaninova, Eriksson, Feibusch, Sanz, Benyus, Dwyer, Miller, Douma, ter Laak, Raspail, Ehlers and Lipton2020). O'Neill et al. (Reference O'Neill, Done, Gettelman, Lawrence, Lehner, Lamarque, Lin, Monaghan, Oleson, Ren, Sanderson, Tebaldi, Weitzel, Xu, Anderson, Fix and Levis2018a, Reference O'Neill, Fanning, Lamb and Steinberger2018b) additionally identified shortcomings in the doughnut economy framework regarding the lack of linkages between social outcomes and resource use, thus risking an inability to determine if existing within a ‘safe and just’ space is achievable. In their study, they suggested the need to more effectively characterize physical and social provisioning systems in future works.
Further, there have been calls to develop sector-based consumption corridors (Fuchs et al., Reference Fuchs, Sahakian, Gumbert, Giulio, Maniates, Lorek and Graf2021). One example is the EAT-Lancet's work in developing what can be viewed as an international SCC for the nutrition sector (Willett et al., Reference Willett, Rockström, Loken, Springmann, Lang, Vermeulen, Garnett, Tilman, DeClerck, Wood and Jonell2019). Multiple studies have identified diets, mobility and housing as lifestyle sectors in which reductions would have the greatest impacts in terms of climate change (Akenji et al., Reference Akenji, Lettenmeier, Koide, Toivio and Amellina2019; Ivanova et al., Reference Ivanova, Wiedenhofer, Macura, Callaghan and Creutzig2020; Shigetomi et al., Reference Shigetomi, Kanemoto, Yamamoto and Kondo2021).
Of these three, mobility is the focus of this article. Transportation is responsible for 24% of global direct CO2 emissions (IEA, 2020) and 14% of total GHG emissions (Lamb et al., Reference Lamb, Wiedmann, Pongratz, Andrew, Crippa, Olivier, Wiedenhofer, Mattioli, Al Khourdajie and House2021). According to Lamb et al.'s (Reference Lamb, Wiedmann, Pongratz, Andrew, Crippa, Olivier, Wiedenhofer, Mattioli, Al Khourdajie and House2021) global decomposition of emissions by sector and sub-sector from 1990 to 2018 (thus without the impacts of the Covid-19 pandemic), emissions from the transportation sector and the road transport sub-sector have been growing steadily and faster than most of the other sectors, averaging a roughly 2% annual growth for both the sector and sub-sector. These growing environmental impacts associated with mobility are occurring while externalities associated with transport, social exclusion (Lucas & Jones, Reference Lucas and Jones2012), accessibility/mobility poverty (Simon, Reference Simon2016) and unequal levels of mobility (Czepkiewicz et al., Reference Czepkiewicz, Árnadóttir and Heinonen2019; Ivanova & Wood, Reference Ivanova and Wood2020; Oswald et al., Reference Oswald, Owen and Steinberger2020) continue to occur globally both in the Global South and Global North.
Considering these issues, it is clear that the mobility sector needs to transform. The field of sustainable urban mobility (SUM) is a complex domain, both underpinned by environmental impacts and social issues. This has led to the study of SUM to often be fragmented between approaches to improve environmental performance at the cost or lack of consideration to social equity, or vice versa. This omission or acknowledgement without further investigation of these interlinkages between these two pillars of sustainable development has led to what has been deemed an ‘intellectual dead-end’ in transport research (Grossmann et al., Reference Grossmann, Connolly, Dereniowska, Mattioli, Nitschke, Thomas and Varo2021).
Suites of sustainability indicators have been proposed to provide dimensions for the multiple domains of sustainability simultaneously. However, both in PB studies and SUM indicator studies, it has been identified that too often studies either develop indicators or discuss thresholds, but they rarely connect the two (Fang et al., Reference Fang, Heijungs and De Snoo2015; Holden et al., Reference Holden, Linnerud and Banister2013; O'Neill et al., Reference O'Neill, Done, Gettelman, Lawrence, Lehner, Lamarque, Lin, Monaghan, Oleson, Ren, Sanderson, Tebaldi, Weitzel, Xu, Anderson, Fix and Levis2018a, Reference O'Neill, Fanning, Lamb and Steinberger2018b). As suggested by Fang et al. (Reference Fang, Heijungs and De Snoo2015), an indicator without threshold tells us very little about the system's sustainability. This mutual lack of links between indicator and threshold studies has been a persistent gap in the literature, which if left unaddressed imbues the risk of sustainability assessments only considering movement from one unsustainable state to slightly less unsustainable states as opposed to a state of intergenerational ‘strong sustainability’. A lack of thresholds for indicators to be associated to and vice versa, where thresholds are determined but lacking measurements of performance and unclear definition of how systems impact them makes operationalization of SCC's difficult.
Therefore, to address this gap, in this article we take an initial step towards the development of a mobility sector-focused SCC, focusing particularly on the urban passenger ground transport sector as a case study. While the increasing prevalence of aviation emissions (Lamb et al., Reference Lamb, Wiedmann, Pongratz, Andrew, Crippa, Olivier, Wiedenhofer, Mattioli, Al Khourdajie and House2021) and the oft-underrepresented emissions from freight (Savy & Burnham, Reference Savy and Burnham2013) are important factors to incorporate when considering the transport sector, the focus on passenger ground transport allows for a higher specificity which could potentially be overlooked with the inclusion of these other sub-sectors. The focus on urban settings is connected to our interest in taking a social provisioning perspective for urban living as well as to allow for greater actionability of the framework.
In this paper, we have attempted to establish a framework to develop and visualize an SCC within an urban context, provide commentary on an established set of SUM indicators to illustrate some of the challenges of associating indicators and thresholds whilst simultaneously considering social and environmental aspects, and lastly, provide examples of indicators and thresholds for an urban mobility sector SCC. The value proposition of this work can be seen as similar to the ‘doughnut economy’ model, at a more granular level for the urban mobility sector, where the existing sector-relevant environmental and social issues and their relation to an SCC framework can be visualized simultaneously with their interrelations considered. This work, therefore, aims to provide a first iteration of an academic contribution towards developing a framework assessing the progress of the transformation to a ‘safe and just space for the urban mobility sector’ bound by an integrated EC and a SF. It is the hope of this first iteration and its future developments can be used to assist policy makers, urban transport researchers, sustainability-focused city-level initiatives (such as C40 or the Covenant of Mayors) and urban planners to incorporate the SCC/‘safe and just’ concepts into their work at the urban level, such as developing SUM plans (with target setting performed with PBs in mind), urban decarbonization plans (where our framework would allow for disaggregated by sector assessments), and sustainability assessments in which both environmental and social aspects could be considered simultaneously, whilst connecting them, similarly as in O'Neill et al. (Reference O'Neill, Done, Gettelman, Lawrence, Lehner, Lamarque, Lin, Monaghan, Oleson, Ren, Sanderson, Tebaldi, Weitzel, Xu, Anderson, Fix and Levis2018a, Reference O'Neill, Fanning, Lamb and Steinberger2018b).
Such perspectives and assessments could potentially be used by researchers to avoid O'Neill et al.'s (Reference O'Neill, Done, Gettelman, Lawrence, Lehner, Lamarque, Lin, Monaghan, Oleson, Ren, Sanderson, Tebaldi, Weitzel, Xu, Anderson, Fix and Levis2018a, Reference O'Neill, Fanning, Lamb and Steinberger2018b) fear of a ‘vanishingly thin ring’, in which living within an SCC becomes unattainable due to the escalation of materials and energy required by provisioning systems to satisfy human needs and wants, by allowing for more granular perspectives of sectoral system's need satisfaction and associated ecological impacts. Further, this integrated perspective allows for commentary on existing SUM indicators and their general lack of connection to thresholds and at times conflicting social and ecological objectives (often dependent on context). The framework developed in this work can also provide guidance for other sector-based works, which has been suggested as an area for further research in the literature (Fuchs et al., Reference Fuchs, Sahakian, Gumbert, Giulio, Maniates, Lorek and Graf2021).
2. Safe and just sustainable consumption corridors
Figure 1 illustrates a simplified version of the process followed in developing the mobility-focused SCC and is the basis of this initial framework we are recommending as a starting point for the operationalization of SCCs.

Figure 1. Framework for the development of a sector-based sustainability consumption corridor.
Following Figure 1, in the first step in developing a sector-oriented SCC, we suggest that a review of the key sustainability issues related to the sector be performed. Next, the EC and SF domains need defining, where following previous ‘safe and just’ and SCC works (Fuchs et al., Reference Fuchs, Sahakian, Gumbert, Giulio, Maniates, Lorek and Graf2021; Raworth, Reference Raworth2012), we suggest the use of the PBs to develop the EC and needs/need-satisfaction theory for SF development (though there could be other viable approaches to EC and SF development). With the understanding of the key sustainability issues associated with the sector provided by the initial review, how these issues relate to the domains of the EC and SF should then be mapped. Lastly, a review of sector-relevant indicator literature should be performed through the perspective of their association and impacts on the EC and SF domains. The review should follow the steps of a comprehensive and transparent literature review which allows for reproducibility. With the review completed, sector-relevant indicators can then be selected (with a variety of indicator subset selection methods available, e.g. Castillo and Pitfield, Reference Castillo and Pitfield2010; JRC-EC, 2008; Mazziotta and Pareto, Reference Mazziotta and Pareto2013) with additional criteria of having relevance to the ECs and SFs. With the indicators selected, they can then be associated with the ECs and SFs in an attempt to dimensionalize the SCC. The process of following these steps will be described in greater detail in the following sections.
Fuchs et al. (Reference Fuchs, Sahakian, Gumbert, Giulio, Maniates, Lorek and Graf2021) defined the ECs as the maximum consumption standards acceptable before the environmental impact of this consumption threatens the ability for others to live safe and good lives, and SFs are defined by the minimum consumption level that individuals now and in the future require to adequately satisfy their needs. The space between these two levels then defines the corridor, as shown in Figure 2 for SCCs in general. These definitions will be used as the basis for our development of ECs and SFs. Lastly, the set of indicators which can measure the progress of the sectors relevant for environmental and social impacts needed to be selected, with thresholds applied that describe a sustainable state of the system. This process will be described first generally, and then applied to the mobility sector, providing a case study of this framework.

Figure 2. Illustration of a sustainable consumption corridor (adapted from Fuchs et al., Reference Fuchs, Sahakian, Gumbert, Giulio, Maniates, Lorek and Graf2021).
2.1 Defining the ecological ceiling
In the construction of the sector-based SCC to promote a ‘safe and just operating space for humanity’, humanity's ‘safety’ in this context lies in its ability to avoid changes to the Earth System such that social-ecological resilience is challenged, affecting our ability to maintain humanity's current socio-economic level of development (Steffen et al., Reference Steffen, Broadgate, Deutsch, Gaffney and Ludwig2015). First established by Rockström et al. (Reference Rockström, Steffen, Noone, Persson, Chapin, Lambin, Lenton, Scheffer, Folke, Schellnhuber, Nykvist, de Wit, Hughes, van der Leeuw, Rodhe, Sörlin, Snyder, Costanza, Svedin, Falkenmark, Karlberg, Corell, Fabry, Hansen, Walker, Liverman, Richardson, Crutzen and Foley2009), the PBs provide a framework identifying the ecological thresholds which, if crossed, could cause non-linear, destabilizing changes to the Earth System which could undermine the ability for people to satisfy their needs and live a good life both now and into the future (e.g. Fuchs et al., Reference Fuchs, Sahakian, Gumbert, Giulio, Maniates, Lorek and Graf2021). Raworth (Reference Raworth2012, Reference Raworth2017a) was one of the first to use the PB framework as an illustrative EC that humanity should ensure does not get trespassed upon to avoid the worst effects of potential destabilization, while simultaneously providing for a SF for humanity. In our work to develop an SCC through the construction of an EC, we took an approach in which we considered the spatial, temporal and finally sectoral contexts of the PBs, as shown in Figure 1.
How PBs spatially differ has been covered in PB literature (Rockström et al., Reference Rockström, Steffen, Noone, Persson, Chapin, Lambin, Lenton, Scheffer, Folke, Schellnhuber, Nykvist, de Wit, Hughes, van der Leeuw, Rodhe, Sörlin, Snyder, Costanza, Svedin, Falkenmark, Karlberg, Corell, Fabry, Hansen, Walker, Liverman, Richardson, Crutzen and Foley2009; Steffen et al., Reference Steffen, Broadgate, Deutsch, Gaffney and Ludwig2015), where it has been explained that some PBs, such as climate change, are global thresholds (e.g. 350 ppm of CO2 in the atmosphere), while other boundaries, such as land-use change or freshwater use, are regional or local thresholds that in aggregate represent a global threshold, both of which types that should not be surpassed to ensure the ability to support human life within that region. The global thresholds and pressures from human systems (e.g. annual GHG emissions) exerted on these thresholds can be downscaled using different approaches.
Which approach to take when allocating the PBs' remaining capacity spatially is a cause of debate, both morally and mathematically. This is complicated by the fact that the relationship between the remaining PB capacity stock and the incoming (pressures) and outgoing flows is a dynamic process in which the state at any moment in time defines what can happen within the sustainable limits. In the simplest manner, it can be done by relating the region's share of global impacts to the impact's levels that are deemed sustainable, and to the current or projected population numbers, for example, yearly CO2eq. emissions (Akenji et al., Reference Akenji, Lettenmeier, Koide, Toivio and Amellina2019; O'Neill et al., Reference O'Neill, Done, Gettelman, Lawrence, Lehner, Lamarque, Lin, Monaghan, Oleson, Ren, Sanderson, Tebaldi, Weitzel, Xu, Anderson, Fix and Levis2018a, Reference O'Neill, Fanning, Lamb and Steinberger2018b) or other environmental footprints per capita (Hoekstra & Wiedmann, Reference Hoekstra and Wiedmann2014). It can be then nuanced in terms of responsibility or capacity, for example, by taking social and historical aspects into account, such as the level of affluence or development (e.g. Raupach et al., Reference Raupach, Davis, Peters, Andrew, Canadell, Ciais, Friedlingstein, Jotzo, Van Vuuren and Quere2014; UNFCC, 2021), by contribution to the impacts in the past (Hickel, Reference Hickel2020), or on a quota basis (Meyer & Newman, Reference Meyer and Newman2018). Another key issue in allocating the impacts to regions is taking either territorial or consumption-based approaches or their variants (Heinonen et al., Reference Heinonen, Ottelin, Ala-Mantila, Wiedmann, Clarke and Junnila2020; Ottelin et al., Reference Ottelin, Ala-Mantila, Heinonen, Wiedmann, Clarke and Junnila2019).
Temporally, as the remaining stock of natural capital assets declines, for example, as the CO2 concentration in the atmosphere grows towards the 350–450 ppm climate change threshold (Steffen et al., Reference Steffen, Broadgate, Deutsch, Gaffney and Ludwig2015), the permissible amount of GHG emissions declines. Models of how to keep global warming below 1.5 °C have many varying pathways of permissible emissions, some requiring negative global emissions (Allen et al., Reference Allen, de Coninck, Dube, Hoegh-Guldberg, Jacob, Jiang, Revi, Rogelj, Roy, Shindell, Solecki, Taylor, Tschakert and Waisman2018; IPCC, 2018). These varying pathways highlight the temporal dynamics associated with EC development and placing a ceiling on the pressures to the ECs.
2.1.1 Mobility sector ecological ceiling development
Using the urban mobility sector as a case study, Figure 3 illustrates the domains defined as a result of the process depicted in Figure 1. It defines three categorical levels using an adapted version of the DPSIR (Driver – Pressure – State – Impact – Response) framework (Smeets & Weterings, Reference Smeets and Weterings1999), in which we focus specifically on the D-P-S part. While we do not see the use of the DPSIR framework as mandatory or necessary in future SCC works, the partial application of the framework is useful for expressing the connection between the ecological thresholds and sustainability indicators and bridging the disciplinary boundaries of the two fields of study, where the PBs can clearly be seen as a state variable (the first level moving visually upward in Figure 3) and our transgressions against the PBs can be described as pressures, shown as the middle level in the figure. Lastly, the drivers can then be described as the independent, external forces which can lead to the pressures, shown as the highest level in Figure 3. For example, in the case of the climate change PB, for the mobility sector, travel demand could represent a driving force, which when powered by fossil fuels leads to GHG emissions – the climate change PB pressure.

Figure 3. Framework for developing indicators of the environmental ceiling in the sustainable consumption corridor for mobility. While presented in the figure as distinct, it is understood that overlaps between impacts across the domains exist.
Thus, in our EC development for the mobility sector and later assessment of SUM indicators, we applied a two-step conversion process (similar to Fang et al., Reference Fang, Heijungs and De Snoo2015), where with the PBs established in Rockström et al.'s (Reference Rockström, Steffen, Noone, Persson, Chapin, Lambin, Lenton, Scheffer, Folke, Schellnhuber, Nykvist, de Wit, Hughes, van der Leeuw, Rodhe, Sörlin, Snyder, Costanza, Svedin, Falkenmark, Karlberg, Corell, Fabry, Hansen, Walker, Liverman, Richardson, Crutzen and Foley2009) work, the functional unit which would impact the PB (pressure) was first determined, and then the relevant drivers of the pressure represented by indicators which describe the system could then be associated to the pressure. From the EC perspective, indicators which have no relation to a driver, pressure, or stateFootnote 1 were considered to be indirectly related to the system and thus excluded. Thus, in our assessment of SUM indicators, we have categorized each indicator as either a state, driver or pressure indicator.
Moving visually upwards in Figure 3, the PBs were separated into four primary domains (though we acknowledge the overlap that can occur between the domains) according to the mobility sector's relationship to each of the PBs, and a global vs local perspective for each. Climate change, biogeochemical flows, ocean acidification, atmospheric aerosol loading, stratospheric ozone depletion and air pollutionFootnote 2 were grouped as output-based thresholds since the mobility sector's impact upon these PBs can be most heavily related to the direct and indirect outputs (in many cases emissions) associated with urban transport.Footnote 3 For these output-based thresholds, we separated the mobility sector's impacts on the PBs into the direct (Tank-to-Wheel) outputs associated with transport, and indirect outputs associated with the embedded emissions in the different vehicle types and supporting infrastructure (such as roads, fuelling stations, Well-to-Tank emissions, etc.) (Dillman et al., Reference Dillman, Árnadóttir, Heinonen, Czepkiewicz and Davíðsdóttir2020; Hawkins et al., Reference Hawkins, Singh, Majeau-Bettez and Strømman2013). The second category was assumed to be land conversion where the land-use change, and associated impacts related to the transport system were connected (Gakenheimer, Reference Gakenheimer2011; Wegener, Reference Wegener2021). Biodiversity loss was additionally its own category, which was then associated with the transportation system's impact on biodiversity, such as collisions with wildlife and habitat fragmentation (Bruschi et al., Reference Bruschi, Garcia, Gugliermetti and Cum2015; Liu et al., Reference Liu, Coomes, Gibson, Hu, Liu, Luo, Wu and Yu2019). The final PB category considered freshwater use, which was then associated with the transportation system's impact on freshwater systems, such as distortion of the water table through the installation of impermeable infrastructure (Lee & Heaney, Reference Lee and Heaney2003).
2.2 Defining the social foundation
Equally important as the reductions of environmental impacts, is what Raworth (Reference Raworth2017a) calls the ‘social foundation’, that is, conditions for all people to live a good life and thrive. Studies on consumption corridors and poverty often rely on a eudaimonic view on wellbeing, including theories of human needs (Dover, Reference Dover2013; Gough, Reference Gough2015) and capabilities (Day et al., Reference Day, Walker and Simcock2016).
The concept of human needs has important advantages in considering well-being in the context of climate change and other ecological impacts (Gough, Reference Gough2015; Lamb & Steinberger, Reference Lamb and Steinberger2017). Firstly, needs are thought to be universal to all people and allow us to identify situations in which serious harm occurs when someone's needs are not met, which is important for inter- and intra-generational considerations of justice that are key to sustainability (O'Neill, Reference O'Neill and Held2011). Secondly, they define needs as something that can be satiated, which allows us to approximate levels of consumption sufficient for decent living (Gough, Reference Gough2017).
Importantly, basic needs (e.g. physical health, critical autonomy) are distinguished from intermediate needs or ‘universal satisfier characteristics’ (e.g. adequate housing, nutritional food and clean water, appropriate health care and education, significant primary relationships, physical and economic security). These are, in turn, distinct from need satisfiers (i.e. objects, activities or relationships that can satisfy basic human needs, such as modes of transport, jobs or friendships), which are context- and culture-specific (Doyal & Gough, Reference Doyal and Gough1991; Gough, Reference Gough2015). A similar distinction between needs and satisfiers has been developed by Max-Neef (Reference Max-Neef1991). What kinds of satisfiers are available and used, and how effective they are in satisfying people's needs largely depends on structural factors, such as the built environment, social relationships, infrastructures, institutions, etc. (Brand-Correa et al., Reference Brand-Correa, Mattioli, Lamb and Steinberger2020; Fanning et al., Reference Fanning, O'Neill and Büchs2020; Gough, Reference Gough2017; Mattioli, Reference Mattioli2016).
Sustainability of human needs allows us to formulate material and energy requirements for decent living within a given context (Gough, Reference Gough2017; Millward-Hopkins et al., Reference Millward-Hopkins, Steinberger, Rao and Oswald2020; Rao & Min, Reference Rao and Min2018). However, what is considered a good or decent life is also socially constructed. Therefore, consumption corridors and related approaches include methods to establish decent living standards with both expert knowledge and participatory processes (Fuchs et al., Reference Fuchs, Sahakian, Gumbert, Giulio, Maniates, Lorek and Graf2021; Gough, Reference Gough2017).
Researchers and practitioners working at the intersection of transportation and poverty have defined multiple, and often compound, aspects of transport poverty (Lucas et al., Reference Lucas, Mattioli, Verlinghieri and Guzman2016; Titheridge et al., Reference Titheridge, Oviedo, Hernandez, Ye and Mackett2014). The lowest income groups are typically the least mobile and are more likely to live in areas with low access to jobs, social networks, public services such as education or health. They are often forced to rely on walking and cycling, even for relatively long distances, or low-quality, inconvenient public transportation. In many cities, low-income communities are also more prone to transport externalities, such as road casualties and pollution. Taking care of the SF in the mobility sector can be thus understood as minimizing transport poverty. There are various ways through which it can be done, and Lucas et al. (Reference Lucas, Mattioli, Verlinghieri and Guzman2016) define transport poverty as encompassing four aspects: transport affordability, mobility poverty, accessibility poverty and exposure to transport externalities (see Figure 4).

Figure 4. Framework for developing indicators of the social foundation in sustainable consumption corridor for mobility. While presented in the figure as distinct, accessibility and mobility indicators overlap (Lucas et al., Reference Lucas, Mattioli, Verlinghieri and Guzman2016).
Socio-cultural construction of living standards points to temporal aspects of consumption corridors. Changes in provisioning systems [e.g. transport infrastructures, Fanning et al. (Reference Fanning, O'Neill and Büchs2020)] might make certain need satisfiers (e.g. a private car) essential for satisfying one's needs (e.g. earn money in a job located away from home, visit a healthcare professional) (Brand-Correa et al., Reference Brand-Correa, Mattioli, Lamb and Steinberger2020; Mattioli, Reference Mattioli2016). Changes in physical structures are often coupled with cultural changes, which means that they influence and are influenced by social expectations, conceptions of the good life and social norms. Changes in both physical and social structures may lead to ‘escalation’ or ‘de-escalation’ of need satisfiers, that is, satisfaction of needs requiring more or fewer resources or incurring higher or lower ecological impacts (Brand-Correa et al., Reference Brand-Correa, Mattioli, Lamb and Steinberger2020).
For example, private cars have been increasingly considered a necessity by members of the public in many countries (Gough, Reference Gough2017; Mattioli, Reference Mattioli2016). Historically created car dependence and a poor provision of public transportation in urban regions may lead to the private car being a practical necessity and a sign of being able to fully participate in society (e.g. Heinonen et al., Reference Heinonen, Czepkiewicz, Árnadóttir and Ottelin2021). These structural developments also produce geographical differences in the context and thresholds of mobility-related social indicators, for example, whether access to a private vehicle is considered essential. Consequently, the level of access to mobility that is considered ‘good’ depends very much on the built environment of a particular urban area, its mobility system and its ability to provide access to essential services, but also on the mobility culture of the area.
Sectoral approaches to defining the SF usually refer to systems that provide for one of the intermediate needs (societal-level satisfiers in Table 1), such as housing, employment or food provisioning, and have led to the formulation of minimum decent living standards in these sectors (Fuchs et al., Reference Fuchs, Sahakian, Gumbert, Giulio, Maniates, Lorek and Graf2021). Mobility is different in that it does not directly answer to any of the intermediate or basic needs (which can be considered ends of social provisioning) but rather provides means to participate in certain activities and social relationships or reach locations that allow for need satisfaction (Mattioli, Reference Mattioli2016, Table 1). This property underlines the multidimensional and contextual character of transport poverty and makes it challenging to define its singular indicator (Lucas et al., Reference Lucas, Mattioli, Verlinghieri and Guzman2016).
Table 1. The chains of need satisfiers including travel and travel modes (based on Gough, Reference Gough2017; Mattioli, Reference Mattioli2016)

Table 1 helps to illustrate the differences between mobility-enhancing and accessibility-enhancing approaches to transportation and land-use planning (Handy et al., Reference Handy, Boarnet, Ewing and Killingsworth2002). Mobility maximizes the potential for movement using one or more modes of transport and is often enhanced by improving the accessibility of certain travel modes (e.g. cars, buses) (Handy et al., Reference Handy, Boarnet, Ewing and Killingsworth2002). Enhancing accessibility thus focuses on de-escalating need satisfiers by reducing the amount of travel required to meet human needs (minimizing the 3rd order satisfier in Table 1). Enhancing mobility, in turn, may in some cases lead to the escalation of need satisfiers, for example, through providing car infrastructure, worsening conditions for walking or cycling, and thus increasing car dependence and externalities of traffic. In such conditions, car ownership and use may become a vital need satisfier while also being a ‘negative satisfier’ (cf. Max-Neef, Reference Max-Neef, Elizalde and Hopenhayn1989) of other needs (or the needs of others). Consequently, focusing on accessibility rather than mobility appears to be better suited to sufficiency-based approaches, such as consumption corridors.
Therefore, Figure 4 illustrates a framework for developing the domains of the SF in a mobility SCC. Following Mattioli's (Reference Mattioli2016) transportation needs framework, it starts with accessibility indicators, which are given higher priority than mobility indicators, due to the different level of need satisfiers they address. Following Lucas et al. (Reference Lucas, Mattioli, Verlinghieri and Guzman2016), we map the dimensions to the main approaches to transport poverty.
2.3 Indicator selection and association
With the sector-focused ECs and SFs defined, the next step in the process shown in Figure 1 was to attempt to provide dimensions for the SCC. SUM indicators have developed in the literature to act as a system of parameters that can be used to describe the many facets of SUM, and the field provides a broad set of indicators, with studies providing varying sets of indicators from a single holistic indicator to well over 100 indicators (Gillis et al., Reference Gillis, Semanjski and Lauwers2016; Gudmundsson, Reference Gudmundsson2004; Haghshenas & Vaziri, Reference Haghshenas and Vaziri2012). To root this initial SCC work in the published SUM literature as much as possible, we used an established SUM indicator set as our first step and associated the indicators to the EC and/or SF domains. The use of established SUM indicators allowed for commentary on the potential faults of traditional SUM indicators in the context of outlining a ‘safe and just operating space for humanity’ and SCCs, such as their lack of ties to thresholds, inability to define a sustainable system state, and their potential for conflicting environmental and social objectives and contextual limitations (Holden et al., Reference Holden, Linnerud and Banister2013).
Reviewing the field of SUM indicator literature, we elected to use the indicator set provided by Sdoukopoulos et al.'s (Reference Sdoukopoulos, Pitsiava-Latinopoulou, Basbas and Papaioannou2019) review, which reviewed 2264 indicators from 78 SUM initiatives, and through this work provided a set of the 47 most commonly cited SUM themes and indicators. This work was selected due to the robustness of the review and its assessment of the most common themes and indicators.
In the following tables, we have organized the indicators which we have determined to be relevant to the ECs and SFs into three categories, namely, those only related to the ECs, those only related to the SFs, and those related to both the ECs and to the SFs.
Table 2 shows the first category of indicators, which are related only to the ECs. The first two columns are the themes and indicators provided by Sdoukopoulos et al.'s (Reference Sdoukopoulos, Pitsiava-Latinopoulou, Basbas and Papaioannou2019) review. The following three columns describe the associated ECs, developed in Figure 3, that each indicator directly or indirectly relates to, the direction of the measurement that would describe sustainable progress of that indicator, and the indicators relation to the DPSIR framework.
Table 2. Sustainable urban mobility indicators from Sdoukopoulos et al. (Reference Sdoukopoulos, Pitsiava-Latinopoulou, Basbas and Papaioannou2019) associated with the derived ecological ceiling, in which the direct/indirect relation to the ecological ceiling, the sustainable direction (which describes the directional change to the indicator that would be considered ‘sustainable progress’ relative to an ecological or social goal), and DPSIR category were assessed
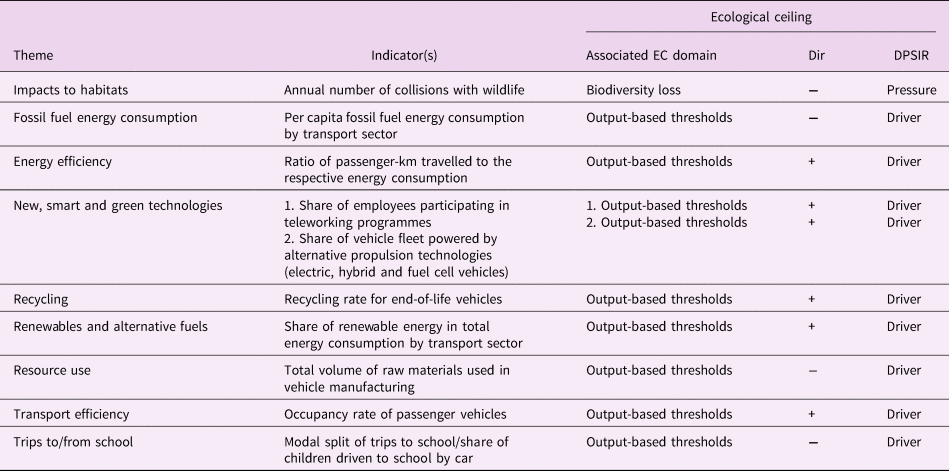
Table 3 follows a similar approach for the indicators which were assessed to be related to just the SF, with the exception that for the last column, rather than using the DPSIR framework, an order satisfier approach was taken, using a needs satisfaction approach (where applicable) (Gough, Reference Gough2017; Mattioli, Reference Mattioli2016). It is worth noting Table 3 saw a significant number of indicators which could be associated with the ‘Exposure to transport’ domain. This is likely due to the fact from the DPSIR framework, the impacts are largely seen as social and economic costs and are thus seen on the SF side of the SCC. Additionally, the accessibility/mobility indicators are more likely to be connected to the actual transportation system as they describe the state of the system, which largely determines the environmental impacts associated with the system.
Table 3. Sustainable urban mobility indicators from Sdoukopoulos et al. (Reference Sdoukopoulos, Pitsiava-Latinopoulou, Basbas and Papaioannou2019) associated with the derived social foundation, in which the direct/indirect relation to the social foundation, the sustainable direction (which describes the directional change to the indicator that would be considered ‘sustainable progress’ relative to an ecological or social goal), and need order satisfier level were assessed

Lastly, Table 4 displays the indicators which were determined to be connected to both the ECs and SFs using the same approach as in Tables 2 and 3. We additionally provide an interlinkage discussion to highlight indicators that are moving either in the same or opposite sustainability directions, signifying an occurrence of where environmental improvement could endanger well-being and vice versa (Arsenio et al., Reference Arsenio, Martens and Ciommo2016). The indicators which were determined to have opposite sustainable directions were organized and are highlighted in red and those with the same in green. These indicators pertain to goods, services or infrastructures at risk of becoming ‘negative satisfiers’ (cf. Brand-Correa et al., Reference Brand-Correa, Mattioli, Lamb and Steinberger2000; Max-Neef, Reference Max-Neef, Elizalde and Hopenhayn1989), which contribute to the satisfaction of some needs while undermining conditions for the satisfaction of others. For those indicators in which their relation to the EC or SF was determined to be contextually dependent, those rows were highlighted in yellow. Expanded upon in the discussion, this table highlights the most contentious mobility indicators with competing environmental and social sustainability directions.
Table 4. Sustainable urban mobility indicators from Sdoukopoulos et al. (Reference Sdoukopoulos, Pitsiava-Latinopoulou, Basbas and Papaioannou2019) associated with both the derived environmental ceiling and social foundation, in which the direct/indirect relation to the social foundation with similar commentary as Tables 2 and 3, as well as a commentary on the environmental and social impact interlinkage of the indicator (where those highlighted in red in yellow indicate potentially countering directional change to the indicator that would be considered ‘sustainable progress’ relative to an ecological or social goal)

2.3.1 Thresholds
After defining the dimensions of the corridor framework and aligning indicators, the next step is to define thresholds of the indicator values that provide the dimensions of a ‘safe and just space’ for a sector. This is a tall task and one outside the scope of this paper. However, using existing examples from the climate change context, we can illustrate how it can be done and highlight the importance of the thresholds and dynamic properties of the SCCs.
Akenji et al. (Reference Akenji, Lettenmeier, Koide, Toivio and Amellina2019) downscaled several climate change mitigation pathways compatible with 1.5 °C warming to per capita emission levels attributable to household consumption (i.e. lifestyle emissions). The resulting targets are 2.5, 1.4 and 0.7 tCO2eq for 2030, 2040 and 2050, respectively.Footnote 4 If the current internal structure of lifestyle carbon footprints were to remain as in five case study countries reported there (from 18% in Brazil to 33% in China), mobility footprints would need to be down to 0.45–0.83 tCO2eq in 2030, 0.25–0.46 in 2040 and 0.13–0.23 in 2050 to maintain a 1.5 °C friendly lifestyle. Similarly, Koide et al. (Reference Koide, Lettenmeier, Kojima, Toivio and Amellina2019) set targets for mobility in Japan at 0.29–0.39 tCO2eq in 2030 and 0.07–0.16 in 2050. Consumption maxima can also be established by downscaling sectoral reduction targets to per capita level. The reduction in transportation-related GHG emissions compatible with limiting warming to 1.5 °C has been proposed at 70–80% lower in 2050 than in 2010–2020 (de Blas et al., Reference de Blas, Mediavilla, Capellán-Pérez and Duce2020; Gota et al., Reference Gota, Huizenga, Peet, Medimorec and Bakker2019). Translated to per capita levels, it would mean downshifting passenger transportation emissions from 0.62 tCO2e in 2018 to 0.10–0.15 tCO2e per person by 2050.Footnote 5
The ranges of these example target estimates emphasize the difficulty in setting thresholds for the ECs. Furthermore, establishing the EC for regions depends on assumptions on the allocation of responsibility and capacity of regions to reduce emissions. Establishing the share of the sector or sub-sector (e.g. urban and long-distance mobility) is also laden with assumptions on its importance for human need satisfaction, and the rate of efficiency improvements and demand reductions that are deemed possible and desirable, among other things.
Regarding the SF, establishing thresholds is similarly challenging. Taking an example from accessibility, one could set a goal of providing all people living in a region access to all essential services and opportunities within their reasonable means. The challenge would then be in defining the essential services and opportunities and the reasonable means. The former could be based, for example, on the needs theory, and include access to intermediate needs and their satisfiers [e.g. healthcare facilities, jobs, meeting places, dwellings of significant relationships, education and childcare facilities (Gough, Reference Gough2015)], but the list would always be contextually- and culturally-specific. Reasonable means also need to be defined, such as a certain share of income spent on mobility, time spent on traveling daily or acceptable distances to certain services. The ‘reasonable means’ are defined in a normative manner and are subject to conventions in planning literature and practice (Páez et al., Reference Páez, Scott and Morency2012). Minimum standards of (the access to) mobility also rely on normative assumptions and are highly contextual, for example, whether the access to a private car is considered a necessity depends on the level of car dependence in a given locality and the popular notions of a decent life (Mattioli, Reference Mattioli2016). Defining thresholds for the SF in the mobility sector would thus require a wider public debate as suggested in previous literature (Fuchs et al., Reference Fuchs, Sahakian, Gumbert, Giulio, Maniates, Lorek and Graf2021; Gough, Reference Gough2017).
3. A safe and just space for urban mobility
Combining the developed ECs and SFs, Figure 5 shows the first illustration of a dimensionalized ‘safe and just space for urban mobility’ using example indicators in the form of a sector-based SCC. This figure provides the benefits of visualizing both the ecological and social dimensions in one image, such that rather than considering these aspects in a siloed manner.
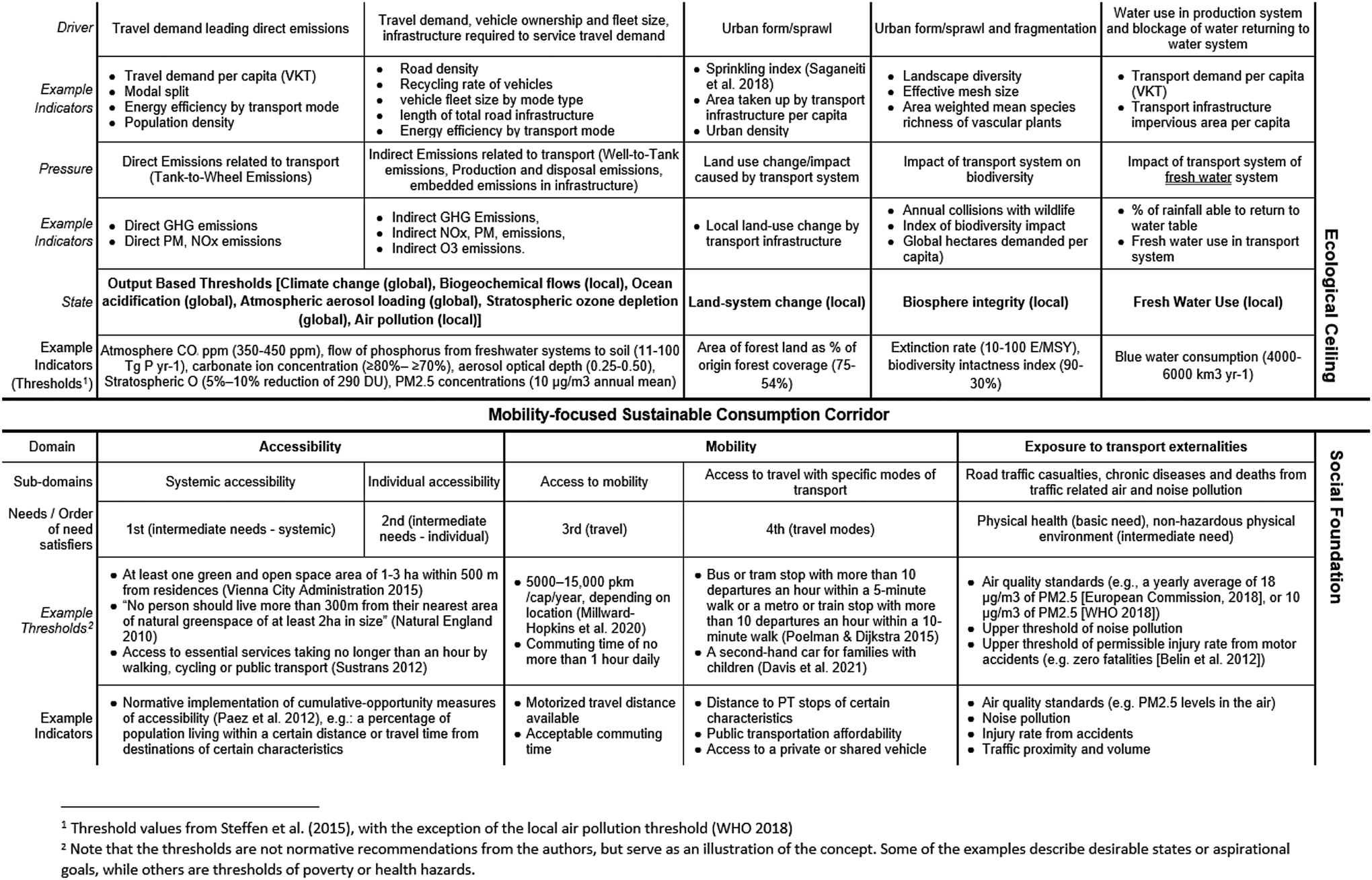
Figure 5. The mobility-focused sustainable consumption corridor with ecological ceilings, social foundations and associated themes, example indicator and example thresholds.
3.1 Integrating ecological ceilings and social foundations
So far, the indicators for the ECs and SFs have been presented separately. The ecological and social issues have also largely been considered separately, in the fields of sustainable mobility and transport poverty (Lucas et al., Reference Lucas, Mattioli, Verlinghieri and Guzman2016). This siloing of issues in transport studies has led to impasse in transport studies where studies in one sustainability domain rarely effectively consider the impacts on the other, and the need to integrate them has been identified (Grossman et al., Reference Grossmann, Connolly, Dereniowska, Mattioli, Nitschke, Thomas and Varo2021). Therefore, in an attempt to integrate them, we suggest incorporating elements of ecological justice directly into social justice indicators in mobility. Indicators of accessibility or mobility poverty often concentrate on the ability of all or particular social groups to reach essential services or have access to travel modes within their reasonable means, such as time (e.g. one-hour travel per day), effort or money (e.g. certain share of income). While these remain relevant, we suggest supplementing them with reasonable ecological means, defined by the EC of a consumption corridor (i.e. its consumption maxima, such as 0.13–0.23 tCO2eq).
Table 5 shows an example of how the definitions and measurements of accessibility and mobility could be potentially modified to integrate such perspectives through the inclusion of references to ECs or consumption maxima. Such integrated definitions can provide both normative and positive measurements of the level of resources necessary for different levels of social provisioning. The development of definitions and indicators through such a lens could provide greater insights into the ability of provisioning systems to provide for human needs whilst remaining within the PBs.
Table 5. Definitions and normative goals for the accessibility and mobility aspects of the social foundation with the ecological ceiling integrated into them

4. Discussion
At the highest level, the first major contribution of this work that we wish to highlight is the transformational perspective shift on the sustainability of the urban mobility sector that SCC/‘doughnut economy’ thinking provides. Too often in policies and studies aimed at improving and studying urban mobility, environmental and social issues are considered separately (Arsenio et al., Reference Arsenio, Martens and Ciommo2016) and without thresholds and vice versa (Fang et al., Reference Fang, Heijungs and De Snoo2015; Holden et al., Reference Holden, Linnerud and Banister2013; O'Neill et al., Reference O'Neill, Done, Gettelman, Lawrence, Lehner, Lamarque, Lin, Monaghan, Oleson, Ren, Sanderson, Tebaldi, Weitzel, Xu, Anderson, Fix and Levis2018a, Reference O'Neill, Fanning, Lamb and Steinberger2018b), which leaves little guidance towards a ‘safe and just space’ for the urban mobility sector (Schwanen, Reference Schwanen2020). With a more clear definition of a sustainable state through the use of ECs, SFs and indicators to measure performance, such thinking can help provide policymakers and other researchers a framework for the development of a ‘strong sustainability’ state (Ayres et al., Reference Ayres, Berrgh and Gowdy2001). This is of increasing importance in the mobility sector, where a rapid decoupling of need satisfaction and material and energy use and associated environmental impacts needs to occur (Brand-Correa et al., Reference Brand-Correa, Mattioli, Lamb and Steinberger2020; Gough, Reference Gough2017) and yet globally energy and material use is trending in the wrong direction (Lamb et al., Reference Lamb, Wiedmann, Pongratz, Andrew, Crippa, Olivier, Wiedenhofer, Mattioli, Al Khourdajie and House2021).
Specifically, within this contribution of key importance, is the identified lack of mutual connections between threshold and indicator sustainability studies. Holden et al. (Reference Holden, Linnerud and Banister2013) identified this issue specifically for SUM indicator studies, suggesting that simply suggesting ‘rate of change’ indicators is not sufficient, and yet to date the SUM indicator literature has seemingly not answered this call. O'Neill et al. (Reference O'Neill, Done, Gettelman, Lawrence, Lehner, Lamarque, Lin, Monaghan, Oleson, Ren, Sanderson, Tebaldi, Weitzel, Xu, Anderson, Fix and Levis2018a, Reference O'Neill, Fanning, Lamb and Steinberger2018b) further identified the need to better characterize social and physical provisioning systems for ‘a good life within planetary boundaries’. Thus, in this work, we thus propose a greater interdisciplinary integration of the fields of SUM indicators, transport poverty, and that of SCCs/doughnut economics and Earth systems studies. This provides a twofold development. First, through such an integration, indicator studies can avoid the pitfall of only measuring movement in a less unsustainable direction and can rather define the sustainable state of the system. Secondly, this integration can assist researchers and practitioners in taking the first step towards dimensionalizing SCCs by better characterizing the ECs and SFs through sector-specific knowledge, allowing for greater provisioning system insights, which has been called for in the literature (Fuchs et al., Reference Fuchs, Sahakian, Gumbert, Giulio, Maniates, Lorek and Graf2021; O'Neill et al., Reference O'Neill, Done, Gettelman, Lawrence, Lehner, Lamarque, Lin, Monaghan, Oleson, Ren, Sanderson, Tebaldi, Weitzel, Xu, Anderson, Fix and Levis2018a). This mutual benefit between threshold and indicator studies was also identified by Fang et al. (Reference Fang, Heijungs and De Snoo2015), who suggested associating PBs to ecological footprinting. Their proposition, while important, did not include social aspects. Thus, this work took a step towards providing a method to characterize both ECs and SFs for specific sectors.
The value in this can be seen in the commentary that this work was able to produce when attempting to apply the most common SUM indicators to an SCC. For example, the operationalization of the SCC was able to provide the context needed to provide feedback on indicators that could potentially be contextually ambiguous and/or have competing social and ecological goals, highlighted in Table 4. The clearest example of this is the vehicle ownership indicator, where it has been well documented that greater levels of private vehicle ownership leads to greater direct and embedded emissions (Dillman et al., Reference Dillman, Czepkiewicz, Heinonen, Fazeli, Árnadóttir, Davíðsdóttir and Shafiei2021), yet other studies use this indicator as a measurement of mobility accessibility (Johnson et al., Reference Johnson, Currie and Stanley2010). The environmental and social aspects of this indicator are thus contradictory, which could lead to potential misuse of the indicator if used in the wrong context.
At a practical and policy level, the authors of this study see multiple potential use cases of the framework proposed in this study and its future versions. First, building on the work of Fang et al. (Reference Fang, Heijungs and De Snoo2015) on connecting environmental footprinting to the PBs, this framework provides the ability to take a more granular step where sector-specific indicators could then be linked to PBs at the urban level (or, in other use cases, at a national or regional level). Utilizing this framework across primary consumption sectors, the results could be aggregated to get a granular sectoral view of the performance of a city against the PBs, similar to Akenji et al.'s (Reference Akenji, Lettenmeier, Koide, Toivio and Amellina2019) or Wiedmann and Allen's (Reference Wiedmann and Allen2021) work. The results of this work could be used as a means to provide improved policy guidance for target setting to align with the PBs in support of ‘strong’ as opposed to ‘weak’ sustainability.
From a social perspective, particularly through the use of the needs-based theories, policy makers and urban planners could use the accessibility perspective adapted in this research to take an alternative approach to mobility system development. Rather than the commonly placed focus on the mobility system itself or technological developments within the system [which in May's (Reference May2015) review of SUM plans, an overemphasis of supply-side solutions was identified], greater attention could be placed on accessibility, transport needs, and addressing the underlying reasons for changes in travel demand an approach which has increasingly been called for in the literature (Mattioli, Reference Mattioli2016; Simon, Reference Simon2016).
Lastly, this framework provides the ability to take an integrated social-ecological perspective where the resources necessary to meet different levels of social provisioning for a sector within the EC can be more granularly investigated. This can assist in determining which elements of the system require de-escalation or higher levels of provisioning to reach sufficiency. In the context of mobility studies, our work responds to an apparent underrepresentation of social issues in sustainable mobility literature and ecological issues in transport poverty literature (e.g. Grossmann et al., Reference Grossmann, Connolly, Dereniowska, Mattioli, Nitschke, Thomas and Varo2021) by considering ecological and social aspects on equal ground.
4.1 Limitations and further study
As the first step of an iterative work, this study was not without limitations, hopefully addressed by future studies. At the broadest level, this work is limited by the PBs themselves, where many unknowns still exist and the thresholds provide a normative range estimation of the gap between a stable ecological state and an unstable one. These are further complicated by the character of these limits which are collectively agreed by people and laden with values and assumptions regarding the desirable state of socio-ecological systems or possible rate of reductions (e.g. Kallis, Reference Kallis2019). This ambiguity extends as one attempts to scale the PBs spatially (Raupach et al., Reference Raupach, Davis, Peters, Andrew, Canadell, Ciais, Friedlingstein, Jotzo, Van Vuuren and Quere2014), sectorally (Akenji et al., Reference Akenji, Lettenmeier, Koide, Toivio and Amellina2019) and temporally (Meyer & Newman, Reference Meyer and Newman2018), especially when considering shared responsibility and equitable distribution (Hickel, Reference Hickel2018).
Establishing SFs presents similar challenges of ambiguity as discussed in the Thresholds section, particularly so for transport, where transport represents more the means to an end than a need itself, thus making generalizations regarding the need for transport in different contexts difficult (Mattioli et al., Reference Mattioli, Lucas and Marsden2017). Furthermore, establishing SF thresholds is inherently normative and laden with assumptions regarding a ‘good’ or ‘decent’ life. Consider examples provided in Figure 5. Some of them describe aspirational goals (e.g. the high levels of public transportation or greenspace accessibility), while others signify the state of poverty or deprivation (e.g. a long commute), or a health hazard (e.g. air pollution standards). What is an aspirational goal in one setting can be a minimum standard in different circumstances, depending on the level of ambition, available resources, the initial situation, etc. Setting such thresholds in any given context requires a political process of some kind. In the context of SCC, various deliberative methods involving experts, citizens and political actors have been proposed to guide corridor development (Büchs & Koch, Reference Büchs and Koch2019; Fuchs et al., Reference Fuchs, Sahakian, Gumbert, Giulio, Maniates, Lorek and Graf2021; Gough, Reference Gough2017). The framework presented in this article can potentially guide experts working in such processes but does not involve deliberation. Future work should focus more on participatory and deliberative methods used for defining SCCs in various contexts.
A potential pitfall of the sectoral approach taken within this work is the capacity for rebound effects, where the externalities of a change in one sector on other sectors may not be captured. For example, if digital technologies or good local accessibility allow for reduced travel costs and emissions, only to be displaced to another sector or intra-sector, this presents a potential limitation of sector-based approaches to consumption corridors (Lettenmeier et al., Reference Lettenmeier, Liedtke and Rohn2014).
Such an example of an intra-sector rebound effect could be long-distance travel, and particularly aviation, for the efforts to reduce emissions in the mobility sector. This sub-sector has been largely overlooked in the SUM literature, despite its high share (>50%) in the travel-related GHG emissions in wealthy countries and cities (Aamaas & Peters, Reference Aamaas and Peters2017; Czepkiewicz et al., Reference Czepkiewicz, Árnadóttir and Heinonen2019) and a high growth rate in recent decades (Lamb et al., Reference Lamb, Wiedmann, Pongratz, Andrew, Crippa, Olivier, Wiedenhofer, Mattioli, Al Khourdajie and House2021). Aviation emissions are often excluded from urban policies (e.g. climate action plans, sustainable energy action plans, SUM plans) and emission inventories (Elofsson et al., Reference Elofsson, Smedby, Larsson and Nässén2018). A more comprehensive take on urban-level consumption corridors would need to include aviation and other long-distance travel of residents and visitors. More work is also needed to scrutinize aviation's role in contributing to the SF and human need satisfaction.
Another limitation which could be considered is the use of an established SUM indicator set, which provided a shortlist of the most common themes and indicator per theme. This was intentionally done to root the work to the literature but limited the capacity to provide commentary on the many existing SUM indicators in the literature. The commentary that was provided, however, does present an opportunity for future developments. For example, the ECs and SFs provided in this work, or newly adopted ones using the framework, could be used for the development of threshold relevant indicators and the development of SCCs for other sectors. Lastly, future studies could work on defining the ECs and SFs and associated indicators for different sectors and provide case studies for the implementation of the suggested framework. With the mobility sector's continued role in global emissions (Lamb et al., Reference Lamb, Wiedmann, Pongratz, Andrew, Crippa, Olivier, Wiedenhofer, Mattioli, Al Khourdajie and House2021) and the potential for 1.5 °C warming to occur in less than a decade (WMO, 2021), the transformation towards ‘a safe and just space’ for mobility and humanity is as important and relevant as ever, and operationalizing this philosophy will be an important evolving field (IEA, 2020).
Supplementary Material
The supplementary material for this article can be found at https://doi.org/10.1017/sus.2021.28.
Author contributions
Conceptualization, K.D.; Data curation, K.D.; Conceptual development, K.D., M.C. and J.H.; Methodology, K.D., M.C. and J.H.; Visualization, K.D. and M.C.; Writing – original draft, K.D. and M.C.; Writing – review and editing, K.D., M.C., B.D. and J.H.; Project administration, K.D., M.C. and J.H.; Supervision, J.H. and B.D.; Funding acquisition, B.D. and J.H.
Financial support
This work was supported by the Icelandic research council (RANNIS) [grant numbers 185497-051, 2019]; The Icelandic Road Authority (Vegagerðin).
Conflict of interest
The authors declare that they have no known competing financial interests or personal relationships that could have appeared to influence the work reported in this paper.
Research transparency and reproducibility
All data used within this research can be found within the cited works, and the appendix was included for full transparency of the work.