Introduction
Optimized agronomic management strategies – particularly cropping sequences and nutrient management – are the most important agronomic factors for maximizing the yield potential of wheat cropping systems. With regard to current and future agronomic challenges (e.g. climate change, sustainable crop production and food security for a growing world population), it is becoming increasingly important to evaluate the effects of different strategies on the stability of crop yields in the long term (Albers et al., Reference Albers, Gornott and Hüttel2017; Knapp and van der Heijden, Reference Knapp and van der Heijden2018) and help develop resilient crop production systems for the future (Ray et al., Reference Ray, Gerber, MacDonald and West2015; Berti et al., Reference Berti, Dalla Marta, Mazzoncini and Tei2016). Long-term experiments (LTEs) provide a valuable resource for investigating the long-term effects of different agronomic management, soil, annual weather and biotic factors – e.g. pests and diseases (Johnston and Poulton, Reference Johnston and Poulton2018). Ideal field experiments involve relatively large plots under constant management for 20 years or more because they provide more robust data to evaluate yield stability (Grosse and Hierold, Reference Grosse and Hierold2017). A valid statistical analysis fitting the experimental design is needed to accurately evaluate the yield stability, or yield risk, of crops over the years and explain how different agronomic factors affect yield (Piepho, Reference Piepho1996, Reference Piepho1998, Reference Piepho2000). Yield risk is defined here as the probability that the yield falls below a certain threshold (Eskridge, Reference Eskridge1990). The risk calculation is based on a combined analysis of the mean and inter-annual variability of crop yields and follows the cumulative distribution function. Payne (Reference Payne2015) and Onofri et al. (Reference Onofri, Seddaiu and Piepho2016) recommended using a mixed-model approach with residual (restricted) maximum likelihood (REML) for this kind of analysis.
The objective of the current study was to investigate the impact of cropping sequences (#1: continuous v. rotational), fertilization (#2: mineral v. organic) and straw management (#3: removal v. incorporation) on the yield stability and resilience of winter wheat.
Materials and methods
Experimental design and data
The current study was based on the Broadbalk Winter Wheat Experiment located at Rothamsted Research (Harpenden, UK, Herts, AL5 2JQ, UK; 51°48′N, 0°22′W, 128 m a.s.l.) and established by Lawes and Gilbert in 1843 (Lawes and Gilbert, Reference Lawes and Gilbert1864; Macdonald et al., Reference Macdonald, Poulton, Clark, Scott, Glendining, Perryman, Storkey, Bell, Shield, Mcmillan, Hawkins, Powlson, Smith and Smith2018), where wheat has been grown continuously or in rotation every year since.
The field slopes downward by 1 degree from West to East. According to the FAO (Food and Agriculture Organization of the United Nations), the soil was a Chromic Luvisol (FAO, 2015). The soil texture class was a clay loam to silty clay loam over clay-with-flints, and chalk was present below about 2 m. The top-soil (0–23 cm) contained about 250 g/kg sand, 500 g/kg silt and 250 g/kg clay (Gregory et al., Reference Gregory, Bird, Whalley, Matthews and Young2010). However, the clay content varied (190–390 g/kg) throughout the site (Watts et al., Reference Watts, Clark, Poulton, Powlson and Whitmore2006) and increased with soil depth (Jenkinson et al., Reference Jenkinson, Poulton and Bryant2008) 23–46 cm:300 g/kg; 46–69 cm:500 g/kg; but then decreased at 69–92 cm to 490 g/kg. The soil was drained with tile drains (5 cm in diameter and 60–75 cm deep) installed in the centre of each strip. The drains discharged into the main drain at the east side of the field. Soil organic carbon and total nitrogen contents were determined (Appendix A) by high-temperature combustion of finely ground soil in the presence of oxygen (LECO CNS) and subtraction of carbonate-C determined by the extraction of carbon dioxide with diluted acid. Starting in the 1950s, lime was applied when necessary to ensure that yields were not limited by soil acidity and that there was no more than one pH unit difference between plots. The pH value of the soil ranged from 7.0 to 8.0 (0–23 cm depth), which could be considered as a site pH (Macdonald et al., Reference Macdonald, Poulton, Clark, Scott, Glendining, Perryman, Storkey, Bell, Shield, Mcmillan, Hawkins, Powlson, Smith and Smith2018). The mean annual air temperature and rainfall at Rothamsted (30-year mean; 1981–2010) was 9.8°C and 733 mm, respectively (Rothamsted Research, 2019). Other data indicate that the annual mean air temperatures at Rothamsted have been on an upward trend in recent decades, between 1989 and 2018 about 1°C greater than in the previous period (1878–1988) (Macdonald et al., Reference Macdonald, Poulton, Clark, Scott, Glendining, Perryman, Storkey, Bell, Shield, Mcmillan, Hawkins, Powlson, Smith and Smith2018). Given that all treatments examined here experienced the same weather conditions, an analysis of the effects of weather was not included as part of this study. However, annual/monthly temperature and rainfall data for the experimental period 1986–2017 are available online at the electronic Rothamsted Archive (Rothamsted Research, 2020a, 2020b).
Since 1968, the Broadbalk experiment contained ten sections (section 0–9) and 20 treatments (18 or 19 per section). The experiment included five sections in rotation (sections 2, 3, 4, 5, 7), with each phase of the rotation present every year (see field plan; Appendix B). The other sections were in continuous winter wheat. The plot lengths within each section varied between 15.2 m (section 0), 28.0 m (section 1) and 23.2 m (sections 2, 3, 4, 5, 7), each with a plot width of 6 m (except treatment strips 2.1 and 2.2, which were each 4 m wide). Different fertilizer treatments were applied to strips within each section. The sections included different crop managements, with continuous wheat or wheat in rotation, and different straw management practices (incorporation v. removal). The experiment predated modern principals of statistical design, and consequently had no true spatial replication. A more detailed description of the current design of the experiment is available in the Rothamsted Guide to the Classical Experiments (Macdonald et al., Reference Macdonald, Poulton, Clark, Scott, Glendining, Perryman, Storkey, Bell, Shield, Mcmillan, Hawkins, Powlson, Smith and Smith2018).
For the analysis, a partial data set (1986–2017, marked as red frames in field plan; see Appendix B) with, in most cases, unchanged sections and treatments was used to ensure comparability and accurate estimates of yield stability. The partial data set was selected to provide a comparison between the yield stability of continuous wheat (sections 0–1), with or without straw incorporation, with those of the first wheat in rotation (sections 2, 3, 4, 5, 7), and between wheat treated with different mineral fertilizers and/or organic manure (fertilization treatments), as described in Table 1. Yield data from sections 6 (no fungicides), 8 (no herbicides) and 9 (soil differences) were not included in the analysis. The data set was obtained from the electronic Rothamsted Archive ‘e-RA’ (Perryman et al., Reference Perryman, Castells-Brooke, Glendining, Goulding, Hawkesford, Macdonald, Ostler, Poulton, Rawlings, Scott and Verrier2018), and included grain yields of winter wheat. The grain has been adjusted to 85% dry matter (15% moisture).
Table 1. Description of sections and fertilization treatments used for the analysis of yield stability (Broadbalk Wheat Experiment Rothamsted, 1986–2017)
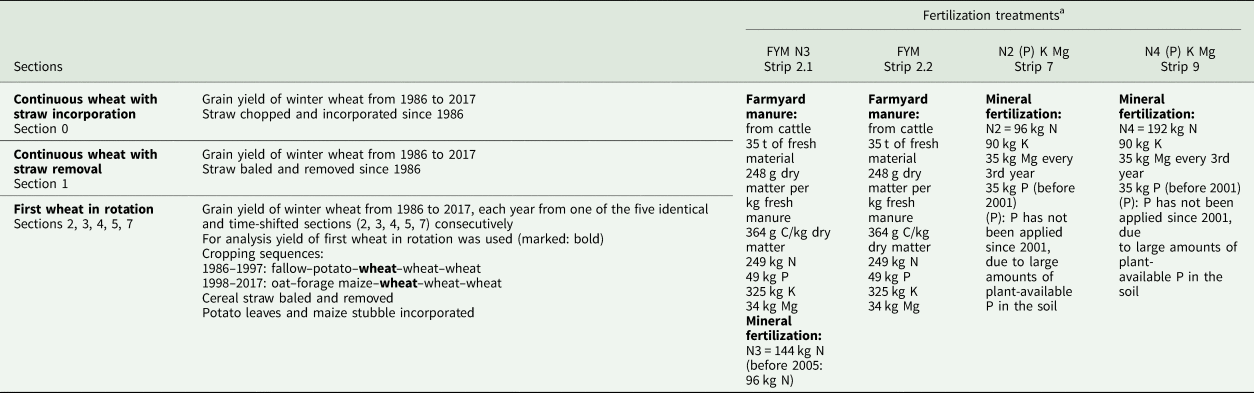
Notes: The numbering/naming of sections and fertilization treatments (strips) were kept identical according to the field plan (Appendix B) and official trial description of Rothamsted Research (www.era.rothamsted.ac.uk/Broadbalk).
a Values stated per hectare, applied annually, unless stated otherwise. N applied as a single dressing in all cases; N (=Nitrogen) applied as ammonium nitrate; P (=Phosphorus) as triple superphosphate; K (=Potassium) as potassium sulphate; Mg (=Magnesium) as Kieserite.
On most strips, N was applied in a single dose in spring (April). Farmyard manure (FYM), P fertilizer, K fertilizer and Mg fertilizer were applied in autumn, usually between the end of September and mid-October. Starting in 1968, FYM, P fertilizer, K fertilizer and Mg fertilizer (in autumn) were applied to the fallow sections of the rotational sections, but N fertilizer was not. Since 2001, P was withheld on many strips until levels of plant-available P decrease to optimal agronomic level. Oats in rotation did not receive mineral N or FYM; potato and maize received the same fertilizer rates as wheat. For the incorporated wheat straw (section 0), the following amounts of nutrients were used based on the analysis of straw yields (mean 5.4 t/ha) from the adjacent section 1: 480 g/kg C (≙ 2592 kg C/ha), 5 g/kg N (≙ 27 kg N/ha), 3 g/kg P (≙ 16 kg P/ha), 11.6 g/kg K (≙ 63 kg K/ha) and 1.2 g/kg Mg (≙ 6 kg Mg/ha). The wheat straw was normally removed by baling and had been incorporated into section 0 since autumn 1986; previously, the straw was removed at harvest. The stubble (10–15 cm tall) and part of the chaff remained for all sections.
There were management problems with field horsetail (Equisetum arvense L.), so no wheat was sown on section 0 in autumn 2002; instead, the section was used in 2003 and 2004 to test various combinations of herbicides. In autumn 2014, no winter wheat could be sown due to unfavourable weather conditions; instead, spring wheat was sown in March 2015. These three years (2003, 2004, 2015) were excluded from the data set.
After autumn ploughing, winter wheat was usually sown in mid-end October at a seed rate of about 350 seeds/m2. Along with the exception noted above when spring wheat was sown in March 2015, unfavourable autumn conditions also resulted in winter wheat being sown very late in January 2001 and February 2013; these were included in the statistical analysis. During the selected experimental period, the following short-strawed winter wheat cultivars (grain–straw ratio of 1:0.8) were grown: Brimstone (1985–1990), Apollo (1991–1995), Hereward (1996–2012) and Crusoe (2013–2018). Pesticides were applied where necessary, except in sections 6 (no fungicides) and 8 (no herbicides), which were not used in the analysis. No irrigation was used in the experiment. Winter wheat was harvested around mid-end August at full grain maturity (GS 92–94). At harvest, a yield strip (2.1 m wide and 15–28 m long: 32–59 m2) was cut from the centre of each plot by a small plot combine harvester, leaving a 10–15 cm stubble in all plots.
Statistical analysis
To account for the experimental design, a mixed model was fitted based on REML, as recommended by Raman et al. (Reference Raman, Ladha, Kumar, Sharma and Piepho2011) and Onofri et al. (Reference Onofri, Seddaiu and Piepho2016). Each plot had a different ‘section × treatment’ combination, with no repetition or randomization, so that plot errors and ‘section × treatment × year’ interactions (=residual) could not be separated. The main effects of ‘section’ and ‘treatment’ and interaction effect ‘section × treatment’ were modelled as fixed; the main effect for ‘year’ and interaction effect ‘section × treatment’ were modelled as random. Furthermore, an autoregressive model AR(1) was used for the residual effect to account for autocorrelation of yields on the same plot across years due to time-varying processes and experimental changes in fertilization, cropping sequence and cultivars (Piepho et al., Reference Piepho, Möhring, Pflugfelder, Hermann and Williams2015). Pairwise comparisons were performed (P < 0.05; all P values corrected by Sidak for multiple testing) between the mean yields of winter wheat for: (a) treatments within a section and (b) between sections within a treatment. The ‘section × treatment’ combinations were tested for a linear trend of wheat yields over the years (1986–2017), but there was no evidence of a temporal trend.
Each ‘section × treatment’ combination was assumed to have a specific variance component (stability variance) for its interaction with years. Specific variances $\lpar \sigma _i^2 \rpar$ were computed as the sum of stability variance of a ‘section × treatment’ combination and the variance of the year main effect. These REML-based estimates of specific variances were used as measures of inter-annual yield variability (year-to-year) in accordance with Shukla's ‘stability variance’ (Piepho, Reference Piepho1998, Reference Piepho1999), with lower values indicating fewer variable yields across years (=higher yield stability). The model allowed yield variability to be determined independently of yield level, as recommended by Shukla (Reference Shukla1972). Yield variability can be incorrectly interpreted if there is a systematic dependency of variation from the mean (Döring and Reckling, Reference Döring and Reckling2018). No such dependencies were found in this analysis.
Based on the model described above, the parameters ‘section × treatment’-specific means μi and standard deviations σi (based on the specific variances $\sigma _i^2$; see above) were used in the second step for the analysis of yield risk according to Eskridge (Reference Eskridge1990). As shown in Eqn (1), the yield risk described the probability p(i) that the wheat yield in a ‘section × treatment’ combination i falls below a certain threshold yield level (=threshold δ) during the analysed trial period (1986–2017):

with Φ as the cumulative density function of the standard normal distribution. The standard normal distribution of yields was verified by the Shapiro–Wilk Test of Normality (sig. 0.339; P < 0.05). The yield risk (probability of not achieving a threshold yield level) was calculated for a broad range of thresholds δ according to the range of observed yields between δ = 0–13 t/ha (in steps of 0.1 t/ha). These results were plotted as curve progressions for specific ‘section × treatment’ combinations (x-axis: thresholds; y-axis: risk values; Fig. 1). Additionally, yield risk comparisons of continuous wheat v. first wheat in rotation for the different fertilization treatments are presented in Fig. 2. These graphs were generated by plotting the yield risk, p(i) values per threshold δ, of one ‘section × treatment’ combination (x-axis) against the yield risk, p(i) values per threshold δ, of another ‘section × treatment’ combination (y-axis) to directly compare their yield risks over the full range of threshold yield levels (δ = 0–13 t/ha; in steps of 0.1 t/ha).
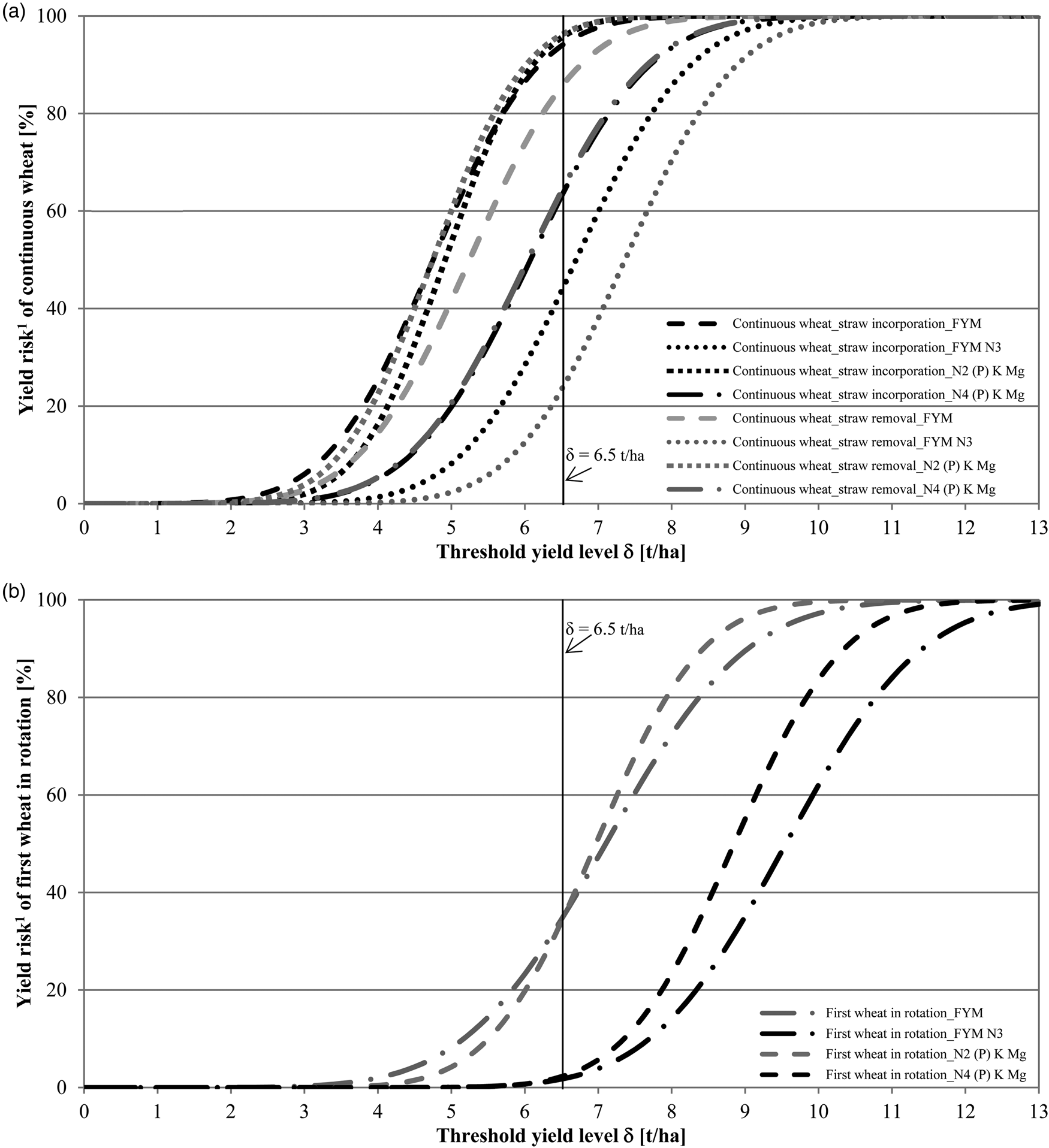
Fig. 1. (a-b). Yield risk comparison of fertilization treatments over a range of threshold yield levels for (a) continuous wheat (straw incorporation and straw removal) and (b) first wheat in rotation (Broadbalk Wheat Experiment Rothamsted, 1986–2017)

Fig. 2. (a-d). Yield risk comparison of continuous wheat (straw incorporation and straw removal) versus first wheat in rotation for fertilization treatments (a) FYM, (b) FYM N3, (c) N2 (P) K Mg, (d) N4 (P) K Mg (Broadbalk Wheat Experiment Rothamsted, 1986–2017)
For statistical analysis, the software SAS (Version 9.4; SAS Institute Inc.; Cary, North Carolina, USA) and SPSS (Version 24; IBM SPSS Statistics; Armonk, New York, USA) were used. The syntax for the model is presented in Appendix C.
Results
Yield
The mean yield over all sections and treatments during the trial period (1986–2017) was 6.53 t/ha (Fig. 3; range of yield values in Appendix D). In the FYM N3 treatment, straw removal led to a significantly higher mean yield for continuous wheat than when straw was incorporated; straw management had no significant effects on continuous wheat yields for any other treatments. The cropping sequence had a significant effect on mean yield, with significantly higher yields for first wheat in rotation compared with continuous wheat crops.

Fig. 3. Mean yield of winter wheat depending on fertilization treatment for continuous winter wheat (straw incorporation and straw removal) and first wheat in rotation (Broadbalk Wheat Experiment Rothamsted, 1986–2017)
Throughout all the sections, the application of FYM with mineral N (FYM N3) resulted in the highest mean yields (up to 9.6 t/ha), followed by mineral fertilizers with 192 kg N/ha (N4 (P) K Mg). In contrast, applying FYM alone resulted in a lower mean yield. The lowest mean yield (4.7 t/ha) was recorded with N2 (P) K Mg, which received 96 kg N/ha, in the continuous wheat section with straw removal (Fig. 3).
Yield risk
The yield risk – e.g. the risk of not achieving the threshold yield level depending on the fertilization treatment – was calculated for continuous wheat (Fig. 1(a)) and first wheat in rotation (Fig. 1(b)). The yield risk for all analysed sections was highest in the N2 (P) K Mg and FYM treatments, which is indicated by the left-hand side curve progressions (Figs 1(a) and (b)). At lower yield levels, there were higher risks of yield losses in the N2 (P) K Mg and FYM treatments (higher slope/gradient of curves at thresholds δ = 3–5 t/ha) compared to the FYM N3 and N4 (P) K Mg treatments. In contrast, the yield risks in treatment N4 (P) K Mg and in treatment FYM N3 were much lower, which is indicated by the right-hand side curve progressions (Figs 1(a) and (b)).
Continuous wheat and first wheat in rotation were compared (Figs 2(a)–(d)). The yield risks in diverse cropping sequences were much lower than in continuous wheat (indicated by convex curve progressions). Sections that underwent crop rotation had a lower risk of yield loss in all fertilization treatments, and this was most pronounced with N4 (P) K Mg, which received a larger amount of N fertilizer (Fig. 2(d)). Furthermore, straw removal had a positive effect on the continuous wheat sections with FYM supplied (Figs 2(a) and (b)); there was a higher yield risk under straw incorporation than removal, which is indicated by a more convex curve progression in the black curve than the grey curve. This effect was not evident on the minerally fertilized plots, N2 (P) K Mg and N4 (P) K Mg, where the yield risk of the two continuous wheat sections was similar (relatively equal progressions of black and grey curves; Figs 2(c) and (d)).
The ‘section × treatment’ combinations were compared by setting the threshold yield to an overall mean δ = 6.5 t/ha (grey guide lines in Figs 2(a) and (d)), and the lowest yield risks were found for first wheat in the rotation receiving FYM combined with N fertilizer (FYM N3: Fig. 2(b)), and for the treatment with high mineral N input (N4 (P) K Mg: Fig. 2(d)). In contrast, higher yield risks were found for continuous wheat with FYM application only (Fig. 2(a)), and the highest risk was found for continuous wheat with less N fertilizer (N2 (P) K Mg: Fig. 2(c)).
Inter-annual yield variability
In the continuous wheat section with straw incorporation, the highest inter-annual yield variability (Fig. 4) was found for low mineral N supply (N2 (P) K Mg). A lower variability (indicating more stable yields) was determined for higher mineral fertilization (N4 (P) K Mg), and the lowest variability was associated with FYM combined with mineral N fertilizer (FYM N3). In the continuous wheat section with straw removal, the yield variability was lowest for higher mineral N input (N4 (P) K Mg), and higher yield variability was obtained in the N2 (P) K Mg and FYM N3 treatments. The most unstable yields were found with FYM application only. The highest yield variabilities of first wheat in rotation were shown for FYM, especially when combined with mineral N (FYM N3). Mineral fertilization led to somewhat lower yield variability of first wheat in rotation, and variability was lowest in N2 (P) K Mg (Fig. 4).

Fig. 4. Inter-annual yield variability depending on fertilization treatment for continuous wheat (straw incorporation and straw removal) and first wheat in rotation (Broadbalk Wheat Experiment Rothamsted, 1986–2017).
Overall, the results of inter-annual yield variability differed across sections and treatments. Comparing all ‘section × treatment’ combinations, the lowest yield variability (=1.00; indicating the most stable yields) was found for continuous wheat with straw removal and the largest amount of N (N4 (P) K Mg). In contrast, the highest yield variability (=2.18) was obtained for the first wheat in rotation that received FYM plus mineral N (FYM N3; see Fig. 4).
System resilience
A high system resilience was found for first wheat in rotation receiving 192 kg/ha of mineral N fertilizer (N4 (P) K Mg) combining high mean yield level (8.8 t/ha) with greater yield stability, indicated by low inter-annual variability (filled black square in Fig. 5) and low yield risk (right-hand side curve progression in Fig. 1(b); convex curves in Fig. 2(d)). High system resilience was also observed for the rotational wheat with FYM N3; this treatment had the highest mean yield level (9.6 t/ha), low yield risk (right-hand side curve progression in Fig. 1(b)), but much higher inter-annual variability indicating less stable yields (square with stripes in Fig. 5).

Fig. 5. Comparison of mean yield v. inter-annual yield variability of winter wheat depending on fertilization treatment for continuous winter wheat (straw incorporation and straw removal) and first wheat in rotation (Broadbalk Wheat Experiment Rothamsted, 1986–2017).
In contrast, the continuous wheat section with straw removal and FYM N3 (circle with stripes in Fig. 5) showed a lower yield level, but with stable yields and relatively low yield risk (right-hand side curve progressions in Fig. 1(a)). Continuous wheat with straw removal receiving higher amounts of mineral N supply (N4 (P) K Mg) showed less system resilience, resulting in lower yield levels with slightly higher risk (Fig. 1(a)), but also slightly lower inter-annual yield variability (filled black circle in Fig. 5) compared to treatment FYM N3.
The poorest system resilience, combining high risk with low and varying yields, was found in both continuous wheat sections with less mineral N (96 kg N/ha; N2 (P) K Mg: left-hand side curve progressions in Fig. 1(a); patterned diamond and patterned circle in Fig. 5). Similarly, poor system resilience was shown by continuous wheat with FYM only (left-hand side curve progression in Fig. 1(a); filled white diamond and white circle in Fig. 5).
Discussion
Impact of cropping sequence (#1)
Cereal rotations with a large proportion of winter wheat are typical for large areas of northern Europe and other temperate or humid climates, particularly where crop diversity is constrained by physical or economic factors (Lithourgidis et al., Reference Lithourgidis, Damalas and Gagianas2006). Despite environmental concerns, growing winter wheat continuously or performing ‘break crop/wheat/wheat’ rotations might be recommended occasionally as the most profitable ‘cropping sequence’ (Sieling et al., Reference Sieling, Stahl, Winkelmann and Christen2005; Steinmann and Dobers, Reference Steinmann and Dobers2013). However, unfavourable preceding crops and continuous wheat inevitably cause yield losses (e.g. Engström and Lindén, Reference Engström and Lindén2009; Angus et al., Reference Angus, Kirkegaard, Hunt, Ryan, Ohlander and Peoples2015). Yields of wheat grown after a 2-year break (break crops: fallow-potato or oat-maize) were larger than yields of continuous wheat, almost certainly because the effects of soil-borne pests and diseases, particularly take-all root disease (Gaeumannomyces graminis var. tritici), are minimized (Macdonald et al., Reference Macdonald, Poulton, Clark, Scott, Glendining, Perryman, Storkey, Bell, Shield, Mcmillan, Hawkins, Powlson, Smith and Smith2018). This yield benefit clearly reduced the yield risk in this current study, which was lower (in all fertilization treatments) for the first wheat crop in the rotation than for continuous wheat crops.
In contrast, the inter-annual yield variability was higher for the first wheat in rotation, especially in cropping systems with FYM plus mineral N (FYM N3). A possible explanation for this could be that, although the higher yield potential of wheat grown in rotation compared to monoculture resulted in larger yields when environmental conditions (e.g. annual weather and/or soil conditions) were favourable, it also led to more variable yields when environmental conditions were unfavourable. Also, the effects of the preceding crops in the rotation and the additional FYM application should be considered, mainly because the specific amount of crop residues and its mineralization or immobilization will differ over the years, causing variability in subsequent wheat yields. Potatoes are shallow rooted and often leave large amounts of plant-available N in sub-soil (Macdonald et al., Reference Macdonald, Poulton, Powlson and Jenkinson1997). This may result in higher and perhaps less risky and variable yields in the subsequent wheat crop. Maize is deeper rooted and may thus leave fewer mineral N residues and less available water for subsequent crops than potatoes (Brouwer and Heibloem, Reference Brouwer and Heibloem1986). This could result in lower or more variable yields for the subsequent wheat crop.
These results stand in contrast to other LTE studies that have reported that wheat grown in rotations has lower inter-annual yield variability than monoculture wheat (e.g. Arshad et al., Reference Arshad, Soon and Azooz2002; Elen, Reference Elen2002; Babulicová, Reference Babulicová2008). Some other studies have found that wheat inter-annual yield variability was not affected by growing wheat continuously, or did not differ among cropping systems, as a consequence of the high production ability of wheat in the long-term (Procházková et al., Reference Procházková, Hrubý, Dovrtel and Dostál2003; St-Martin et al., Reference St-Martin, Vico, Bergkvist and Bommarco2017). The current results also showed no clear pattern of yield variability over all of the sections and treatments. Further research is needed to provide a definitive explanation of why wheat yields were partly more unstable in rotation than in monoculture (see FYM N3). A valid statistical analysis of the dependency between yield variability and available soil parameters (soil organic carbon and total N, see Appendix A) could not be performed due to insufficient data; however, one hypothesis was that lower yield variability is due to higher soil organic carbon content.
Overall, the sole goal should not be to reduce inter-annual yield variability, but to achieve a good compromise (e.g. favourable combination) between yield level, inter-annual yield variability and yield risk, as it has more practical relevance. Based on the Broadbalk Experiment, the first wheat in rotation receiving a higher mineral N supply (N4 (P) K Mg) presented a very good compromise between high and stable yields and low risk of yield reductions. There are relatively few long-term wheat experiments with different cropping systems that can be used to analyse the effects on inter-annual yield variability (e.g. Varvel, Reference Varvel2000; Nel et al., Reference Nel, Purchase and Barnard2003; Lithourgidis et al., Reference Lithourgidis, Damalas and Gagianas2006; Smith et al., Reference Smith, Menalled and Robertson2007; van der Bom et al., Reference Van der Bom, Magid and Stoumann Jensen2017), and even fewer on yield risk (e.g. Nielsen and Vigli, Reference Nielsen and Vigli2018; Macholdt et al., Reference Macholdt, Piepho and Honermeier2019a). Thus, additional studies that focus explicitly on these topics and incorporate a time period long enough to capture a greater range of climatic conditions are necessary to accurately quantify the long-term impact of different agronomic management treatments on the stability of crop yields.
Impact of mineral fertilizers and organic manure (#2)
Mineral N supply had the highest impact on the yield stability of winter wheat, here determined by yield risk and inter-annual yield variability. Häner and Barbant (Reference Häner and Barbant2006) showed that the impact of mineral N fertilizer on the yield variation (32%) of winter wheat was comparable to that for environmental conditions (35%). In the current study, larger amounts of mineral N fertilizer led to high and stable wheat yields with relatively low production risks, which was also observed in other comparable LTEs by Varvel (Reference Varvel2000), Chloupek et al. (Reference Chloupek, Hrstkova and Schweigert2004), Hao et al. (Reference Hao, Fan, Wang, Dang, Guo and Wang2007), Lollato et al. (Reference Lollato, Figueiredo, Dhillon and Arnall2019) and Macholdt et al. (Reference Macholdt, Piepho and Honermeier2019a). A possible reason for this is that the mineral N buffered against environmental change.
Under conditions of sufficient plant-available N supply and higher accumulated soil N content with related higher mineralization rates, wheat plants can exploit the prevailing growing conditions better and be more resilient to environmental stress (St-Martin et al., Reference St-Martin, Vico, Bergkvist and Bommarco2017). Therefore, a larger supply of plant-available N could be a very important factor in reducing inter-annual yield variability (Knapp and van der Heijden, Reference Knapp and van der Heijden2018), provided that there are no other constraints to plant growth – e.g. pests, diseases, soil acidity or compaction. Soil mineral N in Broadbalk is known to fluctuate annually, so it is not measured routinely (Glendining et al., Reference Glendining, Powlson, Poulton, Bradbury, Palazzo and Li1996). Weather conditions also affect crop development and yield. In a recent study by Addy et al. (Reference Addy, Ellis, Macdonald, Semenov and Mead2020), the wheat yield responses to applied N on Broadbalk were particularly sensitive to mean temperature in November, April and May, and to total rainfall in October.
In contrast, the highest inter-annual yield variabilities and yield risks were observed in treatments with lower levels of mineral N fertilizer or FYM only. These findings are in line with the long-term studies of Kravchenko et al. (Reference Kravchenko, Robertson, Thelen and Harwood2005), Smith et al. (Reference Smith, Menalled and Robertson2007) and Maltas et al. (Reference Maltas, Charles, Jeangros and Sinaj2013), in which yield and yield stability were lower in organically manured and low-input systems than in high-input mineral N systems. However, when N was limited, any form of organic manure (like treatment FYM only) had a positive long-term effect on wheat yields and soil sustainability parameters like organic carbon content (see Appendix A).
The combination of FYM and mineral N fertilizer (FYM N3) resulted in a very good combination of low yield risk with high and stable wheat yields compared to FYM only. A meta-analysis based on 20 LTEs in Europe by Hijbeek et al. (Reference Hijbeek, van Ittersum, ten Berge, Gort, Spiegel and Whitmore2017) showed similar results, where organic inputs led to increased wheat yields, even when sufficient mineral nutrients were provided. The supply of manure combined with mineral N fertilizer can improve not only wheat yield (Ellmer et al., Reference Ellmer, Erekul and Köhn2001; Barzegar et al., Reference Barzegar, Yousefi and Daryashenas2002; St-Martin et al., Reference St-Martin, Vico, Bergkvist and Bommarco2017), but also yield stability (Berzsenyi et al., Reference Berzsenyi, Györffy and Lap2000; Macholdt et al., Reference Macholdt, Piepho and Honermeier2019b). This stabilizing yield effect can be confirmed for the yield risk of continuous wheat in the Broadbalk Wheat Experiment, but regarding inter-annual yield variability not for rotational wheat.
There are several positive aspects of this fertilizer combination (FYM N3) on soil properties, such as: (1) accumulation of total and plant-available nutrients (Kulhánek et al., Reference Kulhánek, Balik, Cerný, Vasák and Shejbalová2014; Mazur and Mazur, Reference Mazur and Mazur2015); (2) physical improvements as a result of better aggregate stability, water infiltration rate, water holding capacity, structure quality and porosity (Barzegar et al., Reference Barzegar, Yousefi and Daryashenas2002; Pagliai et al., Reference Pagliai, Vignozzi and Pellegrini2004); (3) advantages in soil microbial biomass and activity (Clark and Hirsch, Reference Clark and Hirsch2008; Tlustos et al., Reference Tlustos, Hejcman, Kunzová, Hlisnikovský, Zámecníková and Száková2017); and (4) enhanced soil fertility (Edmeades, Reference Edmeades2003; Maltas et al., Reference Maltas, Charles, Jeangros and Sinaj2013; Johnston and Poulton, Reference Johnston and Poulton2018). All these positive aspects provide more favourable growing conditions for wheat plants and may improve the buffer function (resilience) of the soil, which reduces the negative impact of biotic and abiotic stressors on plants and finally results in higher yields and reduced yield risks in wheat, as observed in the Broadbalk Experiment at Rothamsted.
However, increased losses of inorganic N because of leaching from plots with higher N fertilizer rates and large annual applications of FYM have been reported (Powlson et al., Reference Powlson, Poulton, Addiscott, McCann, Hansen and Henriksen1989; Goulding et al., Reference Goulding, Poulton, Webster and Howe2000; Hawkesford, Reference Hawkesford2014). The annual FYM inputs on the Broadbalk Wheat Experiment are large and not typical of commercial agriculture in the UK. Consequently, they are probably environmentally unsustainable (especially nitrate leaching) (Macdonald et al., Reference Macdonald, Poulton, Glendining, Goulding, Perryman and Powlson2017). The FYM treatments are not intended to be representative of current farm practices, but they are useful from a scientific perspective, for example, to examine soil organic matter dynamics (Poulton et al., Reference Poulton, Johnston, Macdonald, White and Powlson2018).
Impact of straw management (#3)
The treatments with FYM and FYM N3 in the continuous wheat sections where the straw was baled and removed had a lower risk of yield loss, but higher inter-annual yield variability, compared to those where straw was incorporated. Straw incorporation (since autumn 1986) has had only a small, and variable, effect on soil C and N (Glendining et al., Reference Glendining, Powlson, Poulton, Bradbury, Palazzo and Li1996; Poulton et al., Reference Poulton, Johnston, Macdonald, White and Powlson2018). Based on soil analysis, the C:N ratio was around 10:1 and did not differ between the two continuous wheat sections with and without straw (Appendix A). The FYM and FYM N3 treatments have received high annual FYM applications (c. 248 kg N/ha; see Table 1) over a long period, increasing the soil N content substantially. This resulted in a narrow C:N ratio.
A possible explanation for the higher risk in the section with straw incorporation and additional FYM application (especially without mineral N supply) is that the mineral N availability is limited for a short period soon after straw incorporation because microbes temporarily immobilize N. This may influence subsequent crop establishment. Turley et al. (Reference Turley, Philips, Johnson, Jones and Chambers2003) also found wheat yield reductions caused by straw incorporation into the soil, which ranged from 5–8% on clay soils to 3–18% on silty clay loam soils (study based on six sites in England). Babulicová (Reference Babulicová2008) found that straw incorporation had significant negative yield effects on continuous winter wheat, which became more significant over the trial period of 32 years. In contrast, Jenkyn et al. (Reference Jenkyn, Christian, Bacon, Gutteridge and Todd2001) found in a 5-year experiment that straw incorporation decreased wheat yields only in the first year, mainly attributed to decreases in available N, but then no effect in the subsequent years due to demineralized N and thus available to support the decomposition of the straw.
However, lower inter-annual yield variability and potential benefits in terms of C sequestration of straw incorporation might need to be offset against possible yield losses (yield risk) or lower mean yield levels; this is another factor for policy makers to consider.
Earlier in the Broadbalk Wheat Experiment (1968–1985), straw was removed in both continuous wheat sections (sections 0 and 1). In 1969–1975, the mean wheat yields were higher in FYM N3-treated plots of section 1 (6.2 t/ha; straw removal) than those of section 0 (4.6 t/ha; later on straw incorporation starting in 1986). Both plots were fertilized and treated identically, and hence there might be other pre-existing differences between the plots, such as underlying soil differences. As already mentioned, the experiment was not properly randomized or replicated, so the existence of pre-existing differences cannot be ruled out. The differences were not evident in plots with FYM, N2 or N4 treatments, and yields were similar in the FYM N3 plots in the 10 years preceding this study (1977–1985). However, in terms of the earlier difference, the effects of straw management in the plots where wheat was grown continuously are inconclusive and require further investigation.
Straw incorporation did not appear to have an effect on yield risk when just inorganic N was applied (N2 and N4 treatments). Here, N availability and subsequent crop establishment did not seem to be affected, even in plots with straw incorporation. However, taking the inter-annual yield variability into account, stabilizing yield effects for continuous wheat as a consequence of straw removal and mineral N fertilization became evident; this was especially the case where more N was applied (N4). Possible reasons for why mineral N fertilization has stabilizing effects are discussed in the previous section.
The current study demonstrated that data from the Broadbalk Wheat Experiment can be used to characterize the long-term yield stability of different cropping systems and helped to better understand the impact of agronomic management strategies on crop yield responses. Further studies are needed to validate the robustness of the results and their applicability in different environments or under future climate predictions. A better understanding of how agronomic management practices can help carbon sequestration and guard against environmental variability is also needed. The risk assessment approach presented here, with the mixed-model analysis based on REML stability parameter allows deeper analysis of LTEs. The approach could be used by researchers in the wider field of agronomy, and also provides a valuable opportunity for farmers, advisors and policy makers to evaluate various farming practices affecting the yield risk of cropping systems (or rather production risk, including economic factors) with regard to climate change adaptation and sustainability.
Conclusion
Overall, the results highlight the scope for improving system resilience and stability in wheat yields through the use of crop rotations (#1) and sufficient N fertilizer with or without organic manure (#2). The impact of straw management (#3: removal v. incorporation) when wheat was grown continuously was not conclusive and warrants further investigation. When straw was incorporated and wheat received inputs of manure (FYM and FYM N3 treatments), the inter-annual yield variability was lower and yield risk was higher than when straw was removed. When inorganic N only was applied (N2 and N4 treatments), straw management did not appear to have an effect on yield risk, but did on inter-annual yield variability. Yields were more stable when straw was removed than when it was incorporated, especially when wheat received more mineral N (N4).
Acknowledgements
We thank the Lawes Agricultural Trust and Rothamsted Research, for data from the e-RA database. Further thanks are extended to Margaret Glendining from Rothamsted Research (e-RA) for data acquisition and to the anonymous reviewers. We would like to thank Editage for English language editing.
Financial support
The Rothamsted Long-term Experiments National Capability (LTE-NC) is supported by the UK Biotechnology and Biological Sciences Research Council under the National Capabilities programme grant (BBS/E/C/000J0300) and by the Lawes Agricultural Trust. The first and second authors acknowledge support by DFG (Deutsche Forschungsgemeinschaft) grants MA 7094/1-1 and PI 377/20-1, respectively.
Conflict of interest
The authors declare there are no conflicts of interest.
Ethical standards
Not applicable.
Appendix A
Soil analysis results of soil organic carbon and total nitrogen contents for the years 1987, 1992, 1997, 2000, 2005, 2010 in continuous wheat sections with straw removal depending on fertilization treatment (Broadbalk Wheat Experiment Rothamsted)

Appendix B
Colour online. Field plan of the Broadbalk Wheat Experiment Rothamsted (1998–2017).
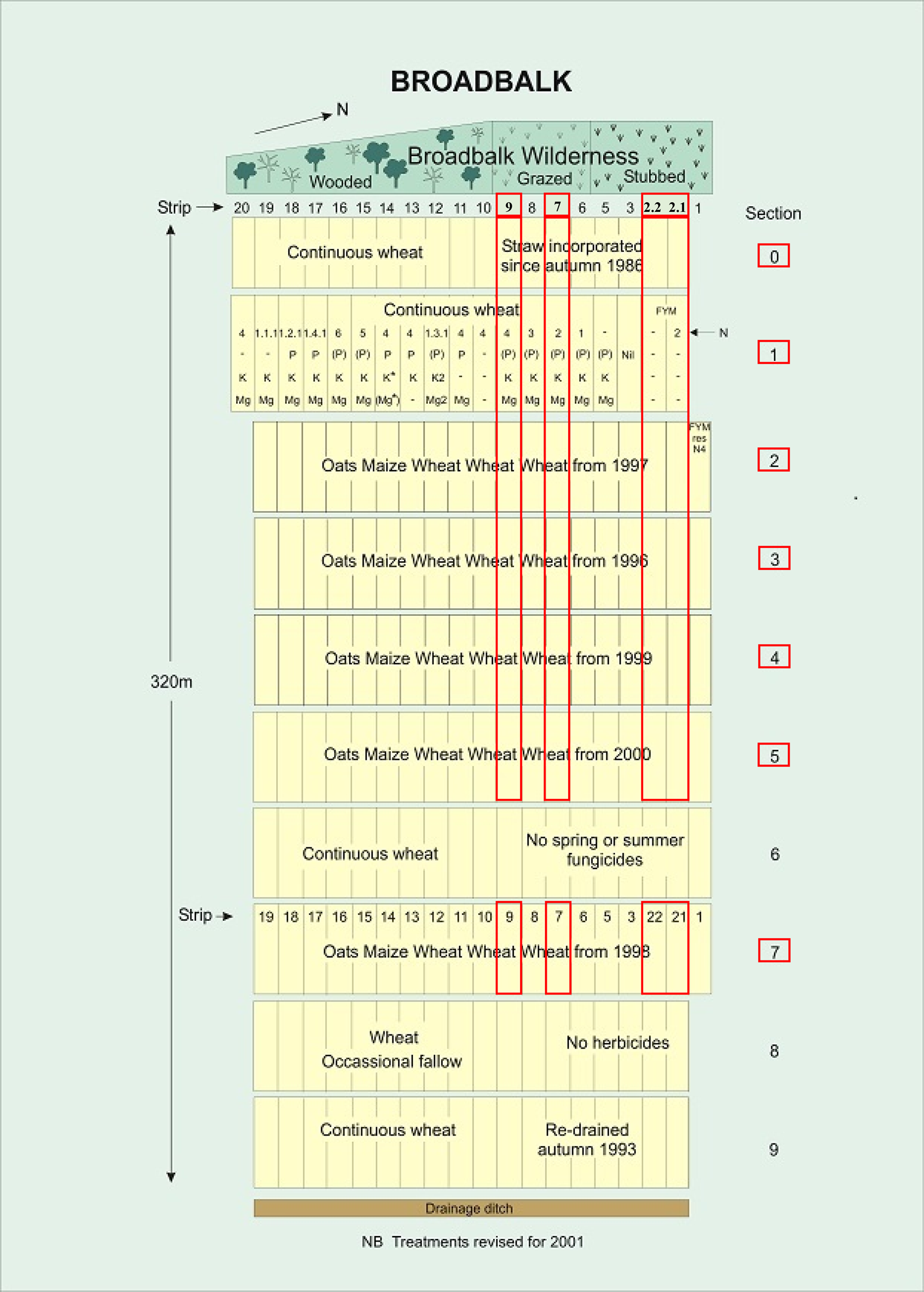
Appendix C
SAS syntax for REML-based yield and yield stability assessment model (Broadbalk Wheat Experiment Rothamsted, 1986–2017).
proc glimmix data = a lognote;
class section treatment year;
model yield = section|treatment;
random int/sub = year;
random year/group = section × treatment;
random year/sub = section × treatment type =ar(1) residual;
lsmeans section × treatment;
run;
Note: Akaike's Information Criterion (AIC) = 1006.21. Description of sections and fertilization treatments used for the analysis in Table 1; Broadbalk field plan in Appendix B.
Appendix D
Box and whisker plots for yield of winter wheat depending on fertilization treatment for continuous winter wheat (straw incorporation and straw removal) and first wheat in rotation (Broadbalk Wheat Experiment Rothamsted, 1986–2017).
