Introduction
Corynosoma enhydri (Acanthocephala) is the most common parasite of sea otters (Enhydra lutris) and the only one known to use them as their main definitive host (Hennessey & Morejohn, Reference Helle and Valtonen1977; Margolis et al., Reference Margolis, Groff, Johnson, McDonald, Kent and Blaylock1997; Mayer et al., Reference Mayer, Dailey and Miller2003). Members of the genus Corynosoma are obligate marine parasites, infecting the intestines of marine mammals and birds worldwide, most commonly in pinnipeds (Aznar et al., Reference Aznar, Pérez-Ponce de León and Raga2006). Knowledge about the ecological relevance and sub-lethal effects of these parasites for marine mammals is limited (Shanebeck & Lagrue, Reference Shanebeck and Lagrue2019). Corynosoma enhydri infections in otters are reported to not cause peritonitis and to induce limited localized tissue reactions (Rausch, Reference Rausch1953; Kreuder et al., Reference Kreuder, Miller, Jessup, Lowenstine, Harris, Ames, Carpenter, Conrad and Mazet2003; Mayer et al., Reference Mayer, Dailey and Miller2003). In seals, C. strumosum infections can cause enteritis, damage to the villi and blockage of the lumen (Lehnert et al., Reference Lehnert, Raga and Siebert2007; Amin et al., Reference Amin2011; Lakemeyer et al., Reference Lakemeyer, Lehnert, Woelfing, Pawliczka, Silts, Daehne, von Vietinghoff, Wohlsein and Siebert2020). It is unclear how large-scale infections may affect hosts, and how immune suppression or metabolic disruption may influence health impacts; though infections with Corynosoma semerme have been associated with colonic ulcers in Baltic grey seals (Lakemeyer et al., Reference Lakemeyer, Lehnert, Woelfing, Pawliczka, Silts, Daehne, von Vietinghoff, Wohlsein and Siebert2020).
The genus is a wide-reaching group; C. strumosum and C. enhydri are the closest related members of Corynosoma and share a recent common ancestor, likely first evolving in seals as they predate otter evolution in the ocean (García-Varela et al., Reference García-Varela, Aznar, Pérez-Ponce de León, Piñero and Laclette2005; Rybczynski et al., Reference Rybczynski, Dawson and Tedford2009). In Europe, another member of the genus, C. semerme, also commonly infects seals (Nickol et al., Reference Nickol, Helle and Valtonen2002). Although closely related, C. enhydri, C. strumosum and C. semerme vary significantly in size, body shape and proboscis morphology (Hennessey & Morejohn, Reference Helle and Valtonen1977; Nickol et al., Reference Nickol, Helle and Valtonen2002). Corynosoma enhydri is the largest, with a maximum size of around 29 mm (Hennessey & Morejohn, Reference Helle and Valtonen1977), while C. strumosum peaks at 9 mm and C. semerme at 2 mm (Nickol et al., Reference Nickol, Helle and Valtonen2002), and infect different portions of the intestine (Nickol et al., Reference Nickol, Helle and Valtonen2002; fig. 1). Prevalence of infection with C. enhydri in southern sea otters has been seen at 90% (Hennessey & Morejohn, Reference Helle and Valtonen1977) and 94.4% (Mayer et al., Reference Mayer, Dailey and Miller2003), while northern sea otters were observed to have a prevalence of 51% (Margolis et al., Reference MacArthur and Wilson1977). Prevalence of Corynosoma infections in seals showed strong variability in geographic location and, over time, measured 56% in the Baltic (n = 26) (Nickol et al., Reference Nickol, Helle and Valtonen2002), 23% in the Wadden Sea (n = 23) (Lehnert et al., Reference Lehnert, Raga and Siebert2007), 87.5% in Japan (n = 40) (Kaimoto et al., Reference Kaimoto, Hirazawa, Masubuchi, Morohoshi, Katahira and Kobayashi2018), 100% in Ireland (n = 26) (O'Neill & Whelan, Reference O'Neill and Whelan2002) and 29.5% in Alaska (n = 105) (Kuzmina et al., Reference Kuzmina, Lisitsyna, Lyons, Spraker and Tollive2012). This difference may be due to host species location, diet and availability of fish intermediate hosts (Nickol et al., Reference Nickol, Helle and Valtonen2002).
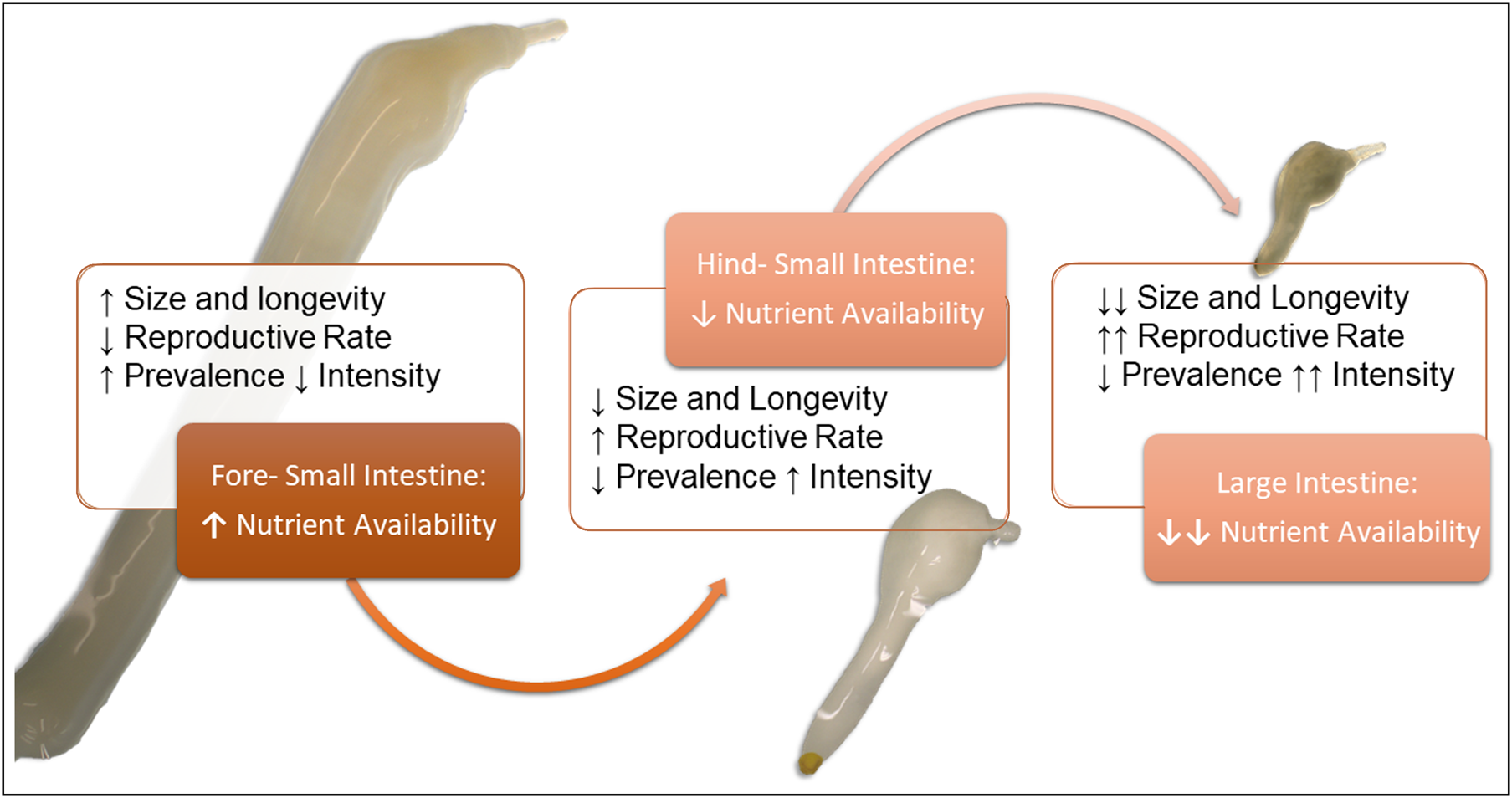
Fig. 1. Visual hypothesis of Corynosoma spp. site selection in the gastrointestinal tract. We hypothesize the differentiation in size and site selection seen previously in Corynosoma strumosum and Corynosoma semerme may be related to a life-history trade-off between longevity and reproduction (MacArthur & Wilson, Reference Margolis, Groff, Johnson, McDonald, Kent and Blaylock1967). Since simple carbohydrate availability decreases along the intestine, parasite longevity (in terms of size and lifespan) will be reduced. Conversely, reproductive rates should increase, as parasites in nutrient-poorer habitats emphasize reproductive output at the cost of adult growth. If this is the case, parasites in low-nutrient environments should show increased intensity associated with seasonality (MacArthur & Wilson, Reference Margolis, Groff, Johnson, McDonald, Kent and Blaylock1967), which has been reported for C. semerme (Helle &Valtonen, Reference Helle and Valtonen1981). Parasites in the beginning of the small intestine, where nutrients are richer, will show increased size, longevity and stable populations (MacArthur & Wilson, Reference Margolis, Groff, Johnson, McDonald, Kent and Blaylock1967), which should be related with higher prevalence but lower intensities in the host population.
Clustering of Corynosoma species within microhabitats of the intestine has been reported (Helle & Valtonen, Reference Helle and Valtonen1981; Valtonen & Helle, Reference Valtonen and Helle1988; Nickol et al., Reference Nickol, Helle and Valtonen2002; Kaimoto et al., Reference Kaimoto, Hirazawa, Masubuchi, Morohoshi, Katahira and Kobayashi2018). The gastrointestinal (GI) tract offers a range of physiochemical gradients, microbiota, immune factors and intraspecific parasite competition for niche differentiation (Berrilli et al., Reference Berrilli, Cave, Cavallero and De'Amelio2012). Microclimates can be created due to availability of nutrients at different stages of digestion, the metabolic processes of gut microbes and their resulting waste products, or even localized immune responses to bacteria or helminth infection (Hooper et al., Reference Hooper, Bry, Falk and Gordan1998; Berrilli et al., Reference Berrilli, Cave, Cavallero and De'Amelio2012). As helminths tend to clump in smaller assemblages due to low numbers in the host, and the intestine is not homologous, site selection may be a significant behaviour for Corynosoma spp. (Holmes, Reference Holmes1990).
Corynosoma strumosum in seals tend to congregate just past the midgut, and C. semerme has adapted to life in the nutrient-poor colon of seals (Helle & Valtonen, Reference Helle and Valtonen1981; Nickol et al., Reference Nickol, Helle and Valtonen2002; Kaimoto et al., Reference Kaimoto, Hirazawa, Masubuchi, Morohoshi, Katahira and Kobayashi2018). These two species differ in size and proportion, with C. semerme being smaller and stouter, with a shorter trunk than C. strumosum (Valtonen & Helle, Reference Valtonen and Helle1988). Corynosoma strumosum is also reported to have increased longevity in comparison to C. semerme (Valtonen & Helle, Reference Valtonen and Helle1988), which may be related to this size differential and location higher in the intestinal tract. Previous research has not conclusively explained observed differences in site selection (Valtonen & Helle, Reference Valtonen and Helle1988; Nickol et al., Reference Nickol, Helle and Valtonen2002; Kaimoto et al., Reference Kaimoto, Hirazawa, Masubuchi, Morohoshi, Katahira and Kobayashi2018); however, due to the differences in size, it is possible that site specificity may be related to available nutrients. Acanthocephalans are absorptive feeders, with carbohydrates as their main source of energy (Kennedy, Reference Kennedy2006). Easily accessible monosaccharides are predominantly absorbed in the upper GI tract of mammals; complex polysaccharides are fermented by commensal bacteria and absorbed downstream, often in the large intestine (Hill, Reference Hill1986). Pinnipeds and sea otters have very short large intestines – in seals, less than 1% of the entire intestinal length on average (Horstmann, Reference Horstmann, Würsig, Thewissen and Kovacs2018). Both groups secrete digestive enzymes to break down macromolecules like carbohydrates in the duodenum, but have remarkably fast digestive transit times (from ingestion to excretion) – three hours for sea otters (Costa & Kooyman, Reference Costa and Kooyman1984) and 5–12 hours for pinnipeds (Horstmann, Reference Horstmann, Würsig, Thewissen and Kovacs2018). This may limit opportunity for the breakdown of complex carbohydrates by commensal bacteria.
This study aims to compare infections of C. enhydri in otters from Alaska and California (isolated otter host populations), with C. strumosum infections of seals from Germany. Distribution in the intestinal tract and sexual success were investigated to better understand the ecology and life-history trade-offs for the genus. Due to previous observations of differences in site selection, size and longevity between C. strumosum and C. semerme (Nickol et al., Reference Nickol, Helle and Valtonen2002), we suspect differences in site selection by this parasite may be related to a life-history strategy of optimization between size (k-selection, longevity) and reproduction (r-selection, transmission) (McArthur & Wilson, Reference Margolis, Groff, Johnson, McDonald, Kent and Blaylock1967; fig. 1). We hypothesize that C. enhydri, as the largest of the Corynosoma spp., would prefer anterior sections of the intestinal tract where simple carbohydrates are more abundant; this trade-off from proximity to the colon for ease of transmission versus nutrients and growth would then also be related to a reduction in reproductive rates.
Materials and methods
Parasite removal and identification
The GI tracts of 19 stranded otters in good condition (dead between zero and four days, with intact intestines) were dissected at the Marine Wildlife Veterinary Care and Research Center in Santa Cruz, California, part of the California Department of Fish and Wildlife (CDFW). Otters were collected through a collaborative stranding network and were part of CDFW's ongoing monitoring of sea otter health, from three different counties (Shanebeck et al., Reference Shanebeck, Lakemeyer, Siebert and Lehnert2020). In Alaska, 12 otters in good condition were collected by a volunteer stranding network through the US Fish and Wildlife Service, Alaska Region, from the Nelson Lagoon (Alaskan Peninsula) and Homer, in the Kachemak Bay (Kenai Peninsula). Alaskan and Californian sea otters were necropsied by staff biologists or veterinarians, the entire intestines removed and frozen for later analysis, except in four cases in California where intestines were investigated directly at necropsy. The collaborating institutions recorded health, estimated age (determined by physical characteristics and dental analysis) (Kreuder et al., Reference Kreuder, Miller, Jessup, Lowenstine, Harris, Ames, Carpenter, Conrad and Mazet2003), sex and location of stranding, except for one animal in Alaska.
To compare the differences between C. enhydri in otters and C. strumosum in seals, the intestines of 22 seals were analysed in Schleswig-Holstein, Germany, from the Baltic (8) and North Seas (14). These included 15 harbour seals (Phoca vitulina) and seven grey seals (Halichoerus grypus). They were necropsied at the Institute for Terrestrial and Aquatic Wildlife Research, Büsum, University of Veterinary Medicine Hannover, Foundation, either freshly or frozen, and age, sex and health were determined (Siebert et al., Reference Siebert, Wohlsein, Lehnert and Baumgärtner2007).
GI tracts were thawed overnight if frozen, then cleaned of the mesentery and omentum by careful removal with a scalpel. The intestine was laid out in full; the small intestine was measured for total length, and cut into five equal sections, the large intestine left intact as the sixth (Nickol et al., Reference Nickol, Helle and Valtonen2002; Sinisalo et al., Reference Sinisalo, Poulin, Högmander, Juuti and Valtonen2003; Aznar et al., Reference Aznar2004; Kaimoto et al., Reference Kaimoto, Hirazawa, Masubuchi, Morohoshi, Katahira and Kobayashi2018). Each section was then opened, and parasites were removed with soft forceps. Individuals were stored in tap water for one to two hours to secure the protuberance of the proboscis, then in 70% ethanol for preservation. Parasites were not always attached to the intestinal wall but found floating in the lumen. This is a possible source of error in our calculations of distribution and conclusion about the preferred niche. However, due to the broad sectioning of the intestine and viscous nature of the intestinal chyme, post-mortem movement between sections would be limited.
Acanthocephalan parasites were identified according to the morphological information outlined by Neiland (Reference Neiland1962), Hennessy (Reference Hennessy1972) and Nickol et al. (Reference Nickol, Helle and Valtonen2002). Prevalence (percent of total otters infected), intensity (total parasites per infected otter) and abundance (average intensity of parasites in infected otters) of acanthocephalan infection were recorded (Bush et al., Reference Bush, Lafferty, Lotz and Shostak1997). Demographic information for the sea otters can be found in Shanebeck et al. (Reference Shanebeck, Lakemeyer, Siebert and Lehnert2020). Total counts of C. enhydri and C. strumosum were recorded, including sex and number of mated females, identified by the presence of a copulatory plug (Violante-Gonzalez et al., Reference Violante-González, Villalba-Vásquez, Monks, García-Ibáñez, Rojas-Herrera and Flores-Garza2017), or if the female was gravid (with fully formed embryonated eggs). Total numbers were recorded for each intestinal section. The average proportion of females to the whole Corynosoma infrapopulation within one otter, as well as mated females to total females, were calculated, excluding cases where there were less than five parasites, or no males were present.
Statistical analysis
Distribution and site selection were analysed statistically using RStudio version 3.5 (Rstudio Team, 2016), with the packages ‘geepack’ (Højsgaard et al., Reference Højsgaard, Halekoh and Yan2006) for use of the generalized estimating equation generalized linear model (GEEGLM), ‘ggplot2’ (Wickham, Reference Wickham2009) and ‘sciplot’ (Morales, Reference Morales2017) for graphical representation. Distribution was based on the total number of parasites per section of intestine, weighed as a dependent variable against the effect of intestinal section, the host's geographic location, intensity of the infrapopulation and the presence/absence of co-infecting parasites per section for otters; and intestinal section, seal species and presence/absence of co-infecting parasites per section for seals. A Poisson distribution with a loglink function was used, and an ‘AR-1’ autoregressive correlation structure which accounts for the correlation of adjacent sites, which we used due to the continuous nature of the intestine (AR-1 assumes the correlation between section 1 and 2 will be α, between section 1 and 3 α2, between section 1 and 4 α3, and so on), individual otter ID defining the clustering and corrected for zero-inflation (Zuur et al., Reference Zuur, Ieno, Walker, Saveliev and Smith2009).
All models at first included all factors and interactions as covariates, then were reduced to the minimum adequate model via stepwise deletion of those that were insignificant. ANOVA and the resulting Wald Statistic table confirmed significance of the regression analyses. GEEGLM was chosen to account for the longitudinal nature of an intestine when determining significance of distribution, and also because this model includes an estimated general mean for the population, helping to avoid bad standard error (SE) estimation because of the limited sample size (Ballinger, Reference Ballinger2004; Zuur et al., Reference Zuur, Ieno, Walker, Saveliev and Smith2009); it was also chosen instead of a generalized linear mixed model due to its better performance in analyses with many individuals (parasites sometimes in the hundreds) but low observations (limited otter sample size) (Zuur et al., Reference Zuur, Ieno, Walker, Saveliev and Smith2009).
Results
Corynosoma enhydri in sea otters
Prevalence of infection in California was 100% of otters infected, and in Alaska 83% of animals were infected. Females had an average abundance of 23, while males had an average abundance of five. Proportionally, females made up 80.7% (±12.5) of southern sea otter intestinal parasite infrapopulations, with 16.9% (±6.7) of females mated (table 1). Northern sea otters showed higher intensities of infection, females making up the majority again, with an average abundance of 244, while males had an average abundance of 33. Proportionally, females made up an average of 84.1% (±9.3) of infrapopulations, with 18.4% (±17.1) mated (table 1). Coinfection with another acanthocephalan species (Profilicollis sp.) occurred in nine of the southern and two of the northern sea otters.
Table 1. Average female composition and mating success of Corynosoma enhydri. Mean percent composition of adult female parasites per infected sea otter including standard deviation (SD) and geographic location (Alaska and California) of the host otters, including the mean percent of mated females by total females, and excluding cases where there were less than five parasites or males were not present.
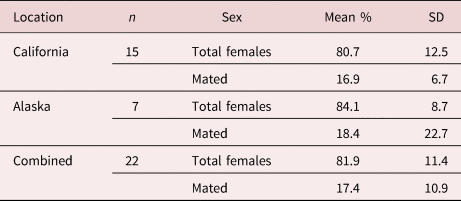
Corynosoma strumosum in seals
Prevalence of Corynosoma infections in grey seals (n = 7) was 71%, intensities ranged from two to 78 parasites, with a mean abundance of 19. Prevalence of Corynosoma infections in harbour seals (n = 15) was 67%, intensity ranging from nine to 130 parasites, with an average abundance of 65. Females made up 74.0% (±6.8) of C. strumosum infrapopulations, with 31.8% (±24.9) females mated in grey seals. In harbour seals, females made up 64.4% (±17.6) of parasite infrapopulations, with 43.1% (±17.5) mated (table 2). Two cases of co-infection with C. semerme were observed. Prevalence of C. semerme infections in the Baltic grey seals was 80% (n = 5). A single individual of Corynosoma magdaleni was found in the colon of one adult male grey seal from the Baltic Sea, along with C. semerme. Another C. strumosum infected a sub-adult Baltic male grey seal, which was found with co-infection of C. semerme in its colon. Two grey seals not infected with C. strumosum were infected with C. semerme in their colons. Co-infections with tapeworm (Diphyllobothrium spp.) were seen in three harbour seals from the North Sea and one grey seal from the Baltic Sea. In all four tapeworm co-infected animals, tapeworms were not found in the fourth or fifth section of the intestine. Tapeworms were also recovered from another harbour seal that was not infected with C. strumosum and, in this individual, they were found throughout the small intestine.
Table 2. Average female composition and mating success of Corynosoma strumosum. Mean percent composition of female adult parasites by total parasites including standard deviation (SD), as well as the mean percent of mated females by total females. Measured in infected seals in Germany, including harbour seals (Phoca vitulina) and grey seals (Halichoerus grypus); excluding one grey seal, which had only two parasites (one male, one female).

Distribution of Corynosoma
In sea otter intestines, the number of parasites discovered by section of intestine was analysed in order to determine the preferred habitat of C. enhydri. The interaction of intestinal section and total number of parasites was significant in determining the number of parasites present per section (df = 5, X 2 = 39, P < 0.001), the second and third sections were observed to have the largest averages (fig. 2), the third section having the strongest correlation (estimate = 1.75, SE = 0.768, z = 2.28). The interaction effect of geographic location with total parasite number was also significant in determining the number of parasites per section (df = 1, X 2 = 173, P < 0.001); southern sea otters had less parasites overall compared to northern sea otters. The interaction effect between intestinal section and geographic location was also significant (df = 5, X 2 = 12, P = 0.041), as seen in the differences in averages per section between sea otters from California and Alaska (fig. 2). Co-infection with Profilicollis sp. was not significant in the model. There was no significant difference in distribution between virgin females, mated females and males in the intestine, though it should be noted in otters from Alaska that males were more often found in the third and fourth sections.

Fig. 2. Distribution of Corynosoma enhydri in sea otters. Bar graph of the average number of C. enhydri counted per section of removal on the y-axis, with the intestinal section they were removed from (equal small intestine sections 1–5; C, colon) on the x-axis for (a) northern sea otters, Alaska, and (b) southern sea otters, California, with standard error given.
Corynosoma strumosum retrieved from seals was found most frequently in the fourth section of the intestine (fig. 3). The interaction effect of intestinal section and total number of parasites per host was significant in determining the number of parasites removed per section (df = 5, X 2 = 63.1, P < 0.001). Though on its own, the total number of parasites was not strongly correlated with the number of parasites per section (estimate = 0.005, SE = 0.006, z = 0.82). The fourth section had the largest average number of C. strumosum (fig. 3), though both the third (estimate = 2.14, SE = 0.464, z = 4.61) and fourth sections (estimate = 2.83, SE = 0.398, z = 7.10) were positively correlated with parasites per section. Distribution between the sexes of C. strumosum was not significantly different. Co-infections with other parasites and seal species were not significant in the model.

Fig. 3. Distribution of individuals of Corynosoma strumosum in seals. Bar graph of the average number of C. strumosum counted per section of removal on the y-axis, with the intestinal section they were removed from (equal small intestine sections 1–5; C, colon) on the x-axis, with standard error given.
Discussion
Corynosoma populations and reproduction
Knowledge about C. enhydri ecology, infection patterns and pathogenicity is limited, and only a few publications are available (Rausch, Reference Rausch1953; Hennessey, Reference Hennessy1972; Hennessey & Morejohn, Reference Hennessy and Morejohn1977; Margolis et al., Reference MacArthur and Wilson1997; Mayer et al., Reference Mayer, Dailey and Miller2003; Shanebeck & Lagrue, Reference Shanebeck and Lagrue2019; Shanebeck et al., Reference Shanebeck, Lakemeyer, Siebert and Lehnert2020). Previous prevalence (90%, Hennessey & Morejohn, Reference Hennessy and Morejohn1977; 94.4%, Mayer et al., Reference Mayer, Dailey and Miller2003), compared with the 100% observed in this study, shows consistent infections in southern sea otters that suggest a stable Corynosoma population. Mean abundance of 30, however, was markedly lower in this study when compared to the previous study by Hennessey & Morejohn (Reference Hennessy and Morejohn1977), which observed a mean abundance of 375. While Mayer et al. (Reference Mayer, Dailey and Miller2003) did not provide an overall abundance, they provided averages across all age classes; from that, the overall abundance would be approximately 400 (approximation is based upon graphical representation of abundance (‘mean density’) for C. enhydri across all age classes) (Mayer et al., Reference Mayer, Dailey and Miller2003). The difference between our observed mean abundance and previous work may be due to our lower host sample size, and the tendency of parasites to aggregate (Kennedy, Reference Kennedy2006; Perrot-Minnot et al., Reference Perrot-Minnot, Bollache and Lagrue2020), our survey perhaps missing the severe infections that may occur. Sample availability was low in Alaska due to logistical constraints, and it remains unclear if the observed difference in prevalence – 83.3% in this study versus 51% in a previous study (Margolis et al., Reference MacArthur and Wilson1997) – is due to increased infection, or low sample size.
This is the first study reporting on sexual dynamics and site selection for C. enhydri in sea otters. In both Alaska and California, females of C. enhydri made up the majority of the population. This is supported by previous research suggesting this dynamic exists because of increased male mortality rates due to higher activity and male–male competition (Poulin & Morand, Reference Poulin and Morand2000; Sasal et al., Reference Sasal, Jobet, Faliex and Morand2000). Interestingly, there was a limited number of females observed to be mated in both California (15.88%) and Alaska (18.29%) (table 1). The slight depression in mating success for Californian C. enhydri may be due to the low intensities of infection in the investigated otters. If infection intensities were higher, mating rates would increase as well. In Alaska, most infections were at similar intensities as southern sea otter cases, with two exceptions (872 and 1680 respectively).
This may explain the slight increase in mating success overall in Alaska, but with a larger variance (table 1). The proportion of mated females was low compared to the findings from C. strumosum in seals, in which females made up 68% of the population and 40% were mated (table 2). Prevalence was also lower in seals than otters, with 65% of seals infected. This is similar to previous observation of harbour and grey seals in the Baltic Sea, with a prevalence of 56% (1986–1987, n = 26; Nickol et al., Reference Nickol, Helle and Valtonen2002) and 70% in harbour seals along the Dutch coast (1988, n = 94; Borgsteede et al., Reference Borgsteede, Bus, Verplanke and Van der Burg1991), though others have seen prevalence as low as 23% (1997–2000, n = 107; Lehnert et al., Reference Lehnert, Raga and Siebert2007) and as high as 95% (1988–1989, n = 110; Strauss et al., Reference Strauss, Claussen, Jäger, Ising, Schneider and Stoye1991) in the German Wadden Sea, the high prevalence occurring concurrently with an epidemic of phocine distemper virus. Other studies of C. strumosum among various species of seals have confirmed that prevalence can be highly variable: 87.5% in Japan (Kaimoto et al., Reference Kaimoto, Hirazawa, Masubuchi, Morohoshi, Katahira and Kobayashi2018), 100% in Ireland (O'Neil & Whelan, Reference O'Neill and Whelan2002) and as low as 8.75% in Alaska (Kuzmina et al., Reference Kuzmina, Lisitsyna, Lyons, Spraker and Tollive2012). These variations are likely tied to fish distribution and abundance, as well as prey availability between localities, as C. strumosum relies on several different fish species as its transport host (Nickol et al., Reference Nickol, Helle and Valtonen2002). In the Baltic Sea, a previous study showed a strong correlation between infection rates and the availability of important transport fish hosts and environmental conditions (Valtonen et al., Reference Valtonen, Helle and Poulin2004).
The observed differences in prevalence and sexual success between C. strumosum and C. enhydri may be due to a variety of reasons. The higher prevalence of females in the population of C. enhydri may limit male access to females or heighten male–male competition (Poulin and Morand, Reference Poulin and Morand2000; Sasal et al., Reference Sasal, Jobet, Faliex and Morand2000). Or it is possible that size differentiation between these closely related species of Corynosoma (C. enhydri, C. strumosum and C. semerme) could be related to a life strategy trade-off between delegation of resources for growth and longevity or reproduction (Valtonen & Helle, Reference Valtonen and Helle1988; Nickol et al., Reference Nickol, Helle and Valtonen2002). Reproductive success may also be tied to intensity of infection and the clumping of parasites within the host, similar to the Allee effect (Greene, Reference Greene, Jørgensen and Fath2008). However, due to the low sample size available, the correlation between intensity of infection and reproductive success was not analysed; future projects should investigate infrapopulation differences in reproductive success and intensity of infection. Otters compared to seals have a high consumption/metabolic rate, healthy adults taking in 23–33% of their body weight daily (Yeates et al., Reference Yeates, Williams and Fink2007). Since C. enhydri are much larger than C. strumosum (Henessey, Reference Hennessy1972; Nickol et al., Reference Nickol, Helle and Valtonen2002), this may be a result of a richer environment. As sea otters have a high daily caloric intake, perhaps C. enhydri can afford to put more energy into growth before reproduction, and this may explain why C. enhydri have such lower proportions of mated females.
Corynosoma distribution
An important aspect of this study was to discover the ultimate realized intestinal niche of C. enhydri in sea otters and compare it between localities, and to its closest relative C. strumosum in seals. Analysis of distribution revealed a significant clumping by C. enhydri, found most frequently in the second and third section of the small intestine, and C. strumosum found most frequently in the fourth section of the small intestine. These closely related acanthocephalan species are not only differentiated in morphology, but also in site selection. Distribution of C. strumosum was not significantly different between grey and harbour seals, which agrees with previous analyses (Valtonen & Helle, Reference Valtonen and Helle1988; Nickol et al., Reference Nickol, Helle and Valtonen2002; Kaimoto et al., Reference Kaimoto, Hirazawa, Masubuchi, Morohoshi, Katahira and Kobayashi2018). There was no significant difference in the distribution between virgin females, mated females and males, even though in Alaskan otters, male C. enhydri were found more often in the third and fourth section; however, perhaps due to the lower sample size in Alaska, this was not significant. Future research should focus on the possible interaction between the number of males and intestinal location on the proportion of mated females.
Since co-infections with Profilicollis spp. were not significant in determining the distribution of C. enhydri in the intestine, interspecific competition is not likely a determining factor of their ultimate realized niche. In seals, co-infections with tapeworm and C. semerme had no significant effect on C. strumosum distribution. Our results and previous research reveal an interesting size-related pattern among Corynosoma. The largest, C. enhydri, was found in the second and third sections of the intestine; the much smaller C. strumosum was found in the fourth section; and C. semerme, the smallest, was found only in the nutrient-poor colon and caecum (Valtonen & Helle, Reference Valtonen and Helle1988; Nickol et al., Reference Nickol, Helle and Valtonen2002). This very specific localization within the intestine in relation to size, starting with the largest in the foregut and smallest in the colon, is a fascinating example of closely related species specializing in habitat (Valtonen & Helle, Reference Valtonen and Helle1988; Nickol et al., Reference Nickol, Helle and Valtonen2002; García-Varela et al., Reference García-Varela, Aznar, Pérez-Ponce de León, Piñero and Laclette2005).
This may be related to physiochemical gradients within the intestine, as acanthocephalans are absorptive feeders and carbohydrates are their main source of nutrition (Hill, Reference Hill1986; Kennedy, Reference Kennedy2006). The hindgut is also dominated by the highest concentration and diversity of bacteria in the intestinal tract, which may be a source of competition (Rerat, Reference Rerat1978). Seals and sea otters also have fast digestive transit times, and short large intestines, which may further limit access to carbohydrates downstream (Costa & Kooyman, Reference Costa and Kooyman1984; Horstmann, Reference Horstmann, Würsig, Thewissen and Kovacs2018). This could explain the size gradient observed between these three closely related Corynosoma species (Hennessey & Morejohn, Reference Hennessy and Morejohn1977; Nickol et al., Reference Nickol, Helle and Valtonen2002; García-Varela et al., Reference García-Varela, Aznar, Pérez-Ponce de León, Piñero and Laclette2005). This distribution may also be related to a life-history strategy between longevity and reproduction. Previous studies comparing Corynosoma in seals noted that C. semerme experience more seasonality in their prevalence, with periods of high abundance (Valtonen & Helle, Reference Valtonen and Helle1988). Corynosoma strumosum tends to have higher prevalence but lower abundance, suggesting longer longevity (Valtonen & Helle, Reference Valtonen and Helle1988). This trend continues in our observations of C. enhydri, which had an even higher prevalence than C. strumosum, with relatively low abundance and, most significantly, a lower mating rate. Previous reports of C. enhydri saw no significant changes in abundance by season, and prevalence was consistently over 90% (Mayer et al., Reference Mayer, Dailey and Miller2003). This suggests our broader hypothesis concerning the relationship between site selection and life-history strategies may be true (fig. 1).
It should be noted that, in addition to differences in site selection, there have also been observed differences in physical damage to the intestine between these species. Corynosoma semerme, the smallest Corynosoma, are more likely associated with colitis and lesions in the colons of grey seals (Lakemeyer et al., Reference Lakemeyer, Lehnert, Woelfing, Pawliczka, Silts, Daehne, von Vietinghoff, Wohlsein and Siebert2020), while C. enhydri have not been observed to be associated with serious physical damage in sea otters (Mayer et al., Reference Mayer, Dailey and Miller2003). It is possible that site selection and changes in the physiochemical gradient may be related to these observed differences. Alterations in nutrient availability can affect intestinal microbiota and influence prevalence and severity of enteritis (Tsukikawa et al., Reference Tsujikawa, Ohta and Nakamura2001; Mohr et al., Reference Mohr, Leisewitz, Jacobson, Steiner, Ruaux and Williams2008; Tsiouris et al., Reference Tsiouris, Georgopoulou, Batzios, Pappaioannou, Ducatelle and Fortomaris2014). Lowered resource availability and increased competition between parasites and microbes in the large intestine could be the cause of increased pathogenicity by C. semerme. We suggest further investigation into the possible relationship between physiochemical gradients and parasite-induced ulceration and inflammation.
Corynosoma enhydri tends to have a weaker penetrative attachment to the intestinal wall (Mayer et al., Reference Mayer, Dailey and Miller2003), and some were found floating in the lumen, which should be noted as a possible source of error in our analysis of habitat selection. However, given the size of the intestines, the limited handling after they were laid out in full and the broad sectioning, artificial movement between sections would be small and limited to the parasites already close to the next section, and should not change our general conclusions about the preferred niche. However, the majority of parasites were found attached to the intestinal wall.
Conclusion
While there were limitations to this study, both in time (samples were collected only in one year) and sample size (collecting from naturally stranded marine mammals greatly limits sample availability), this project gave an invaluable look into an underrepresented species. It was hypothesized that C. enhydri would be found predominantly in the upper intestinal tract due to their large size in relation to other species of Corynosoma, which may be related to a life-history trade-off between growth and reproduction. We observed both a significant clustering in the upper intestine as predicted, as well as lower mating rates than those observed in C. enhydri's smaller relative, C. strumosum, in seals. This discovery offers a fascinating exploration of the little-understood site selection of GI parasites in marine mammals, and suggests further research is needed to properly understand the mechanisms instructing habitat differentiation by parasites and microfauna in the digestive system.
Though still sporadically done, the integration of parasites into the study of ecology is essential for our understanding of marine ecosystems (Lafferty et al., Reference Lafferty, Allesina and Arim2008). Like many parasites, Corynosoma spp. rely on marine food webs to transmit to their final host, and as soft-bodied absorptive feeders, can be particularly helpful in the monitoring of abiotic changes in marine ecosystems (Kennedy, Reference Kennedy2006). Yet, though sea otters are such charismatic and vulnerable species, little knowledge exists about the only parasite that uses sea otters as its preferred definitive host (Mayer et al., Reference Mayer, Dailey and Miller2003; Shanebeck & Lagrue, Reference Shanebeck and Lagrue2019). This study investigated the demographics of C. enhydri in sea otters and established a baseline of their population structure, to better understand their ecology and microhabitat preferences. Acanthocephalans are ubiquitous wildlife parasites and may be useful bioindicators for health and ecosystem-wide effects in vulnerable mammals (Kennedy, Reference Kennedy2006; Shanebeck & Lagrue, Reference Shanebeck and Lagrue2019).
Supplementary material
Supplementary material is available online at https://doi.org/10.1017/S0022149X20000747.
Acknowledgements
The authors would like to thank all the volunteers for reporting and collecting seals – in particular, the national park rangers and seal hunters in Schleswig-Holstein. The health monitoring and collections of seals was partly supported by the Ministry of Energy, Agriculture, the Environment, Nature and Digitalization of Schleswig-Holstein, as well as the National Park Office Wadden Sea Schleswig-Holstein. Special thanks to Dr Melissa Miller, Katie Greenwald and the staff of the Marine Wildlife Veterinary Care and Research Center (a part of the California Department of Fish and Wildlife in Santa Cruz, California), and the volunteers who assisted in animal carcass collection. We also wish to thank Josh Lynn and the volunteers from the Marine Mammals Management Office at the US Fish and Wildlife Office, Alaska Region, Anchorage, Alaska, for provision of Alaskan otter intestines for necropsy, and the support of their facilities.
Financial support
This study was funded by the Institute for Terrestrial and Aquatic Wildlife Research, University of Veterinary Medicine Hannover, Foundation.
Conflicts of interest
None.
Ethical standards
None.