Oxidative stress induced by a high-fat diet (HFD) plays a pivotal role in the aetiology of several conditions associated with hyperlipidaemia including CVD, cancer and failures in metabolism and endocrine functions(Reference Du, Zhao and You1, Reference Odermatt2). The ability of natural products to minimise or prevent such adverse conditions has raised the interest in searching for new lipid-lowering and antioxidant agents from fruits and vegetables(Reference Asdaq and Inamdar3, Reference González-Castejón and Rodriguez-Casado4). Phytochemicals (e.g. polyphenols, terpenoids and organosulphurs) have been proposed as the major compounds responsible for the health benefits associated with a diet rich in plant foods, due to their antioxidant, anti-carcinogenic, and anti-inflammatory effects(Reference González-Castejón and Rodriguez-Casado4, Reference Bahramikia and Yazdanparast5).
Glucosinolates (GL), and their breakdown products isothiocyanates (ITC), largely present in Brassicaceae have been reported to modulate phase II enzymes and antioxidant machinery, through the activation of the Kelch-like ECH-associated protein 1–NF-erythroid-2-related factor-antioxidant responsive element signalling pathway(Reference Vasanthi, Mukherjee and Das6–Reference Herr and Büchler8). The property of directly scavenging reactive oxygen species (ROS) has also been reported for a number of these sulphur-containing compounds(Reference Valgimigli and Iori9). The cellular defence mechanism against radicals consists primarily of direct antioxidant agents (e.g. glutathione (GSH), vitamins and polyphenols), and of various enzymatic activities, such as superoxide dismutase (SOD), catalase (CAT), glutathione peroxidase, NAD(P)H:quinone reductase (NQO1) and glutathione reductase(Reference Valgimigli and Iori9, Reference Yazdanparast, Bahramikia and Ardestani10). Hypercholesterolaemia and obesity have been associated with decreased antioxidant capacity, culminating in an oxidative stress condition(Reference Yazdanparast, Bahramikia and Ardestani10, Reference Bhandari, Kumar and Khanna11). A HFD has also been reported to affect phase II enzymes and GSH levels in the rodent liver(Reference Yoshinari, Takagi and Yoshimasa12, Reference Ghose, Omoluabi and Gandhi13), which can be counteracted by natural products.
Broccoli sprout extract has shown hypocholesterolaemic effects and to reduce body-weight (BW) gain in Syrian hamsters(Reference Rodríguez-Cantú, Gutiérrez-Uribe and Arriola-Vucovich14) and in rats fed a HFD(Reference Lee, Shin and Lee15), respectively. A phase 1 study has reported improved cholesterol metabolism and decreased oxidative stress markers, in healthy subjects who consumed broccoli sprouts for 1 week(Reference Murashima, Watanabe and Zhuo16). Moreover, the potential medicinal effects of Brassicaceae, concerning lipid metabolism and antioxidant properties, have also been documented(Reference Komatsu, Miura and Yagasaki17–Reference Kataya and Hamza19).
Overall, it is still unclear whether these preventive effects are due to single classes of molecules (e.g. GL or ITC) or to the synergistic interactions of several thousand compounds within the whole vegetable(Reference Holst and Williamson20–Reference Heinen, Verhage and Goldbohm22). Interestingly, a stronger cholesterol-lowering activity of broccoli sprouts, compared with that of individual phytochemicals (i.e. ITC sulforaphane), has been shown recently(Reference Rodríguez-Cantú, Gutiérrez-Uribe and Arriola-Vucovich14).
Brassicaceae comprise a complex mixture of bioactive sulphur-containing compounds, flavonoids, minerals and vitamins, and are particularly rich in GL, which may be hydrolysed to ITC by β-thioglucosidase EC 3.2.1.147 (myrosinase, MYR), present in the subcellular plant cell compartments or in the mammalian intestine(Reference Vasanthi, Mukherjee and Das6, Reference Latté, Appel and Lampen23). It is believed that the health effects of Brassica vegetables are mediated by the breakdown products ITC, and that the parent compounds are biologically inert, although a direct activity of intact GL might not be completely excluded(Reference Abdull Razis, Bagatta and De Nicola24, Reference Abdull Razis, Bagatta and De Nicola25). In the case of plant MYR inactivation, the intestinal hydrolysis of GL to ITC, which occurs with efficiency less than 20–30 %(Reference Herr and Büchler8, Reference Perocco, Bronzetti and Canistro26), represents the rate-limiting step affecting the bioavailability of ITC. The co-ingestion of a MYR source, or a pre-digestion with an exogenous MYR, has therefore been assumed as a strategy to increase GL conversion and the resultant potential benefits(Reference Clarke, Hsu and Riedl27). More efforts are needed to identify the mechanisms of action of Brassicaceae in counteracting the deleterious consequences of a diet rich in fat. Moreover, there is a paucity of literature on the deregulation of phase II enzymes by a HFD, thus it is worth investigating how to suppress these alterations, since therapeutic drugs may become ineffective or even toxic in hyperlipidaemic condition(Reference Sugatani, Sadamitsu and Wada28).
The aim of the present study was to disclose the influence of Brassica oleracea L. var. acephala (DC) subvar. laciniata L. (a variety of kale generally known as Tuscan black cabbage or Cavolo nero) sprout extract (TBCSE) on antioxidant as well as phase II enzymes, in the livers of rats fed a HFD. The effect on serum lipid profile (total cholesterol (TC), TAG and NEFA) was also assessed. To enhance the protective effect of TBCSE against the HFD, the ‘pre-digestion’ of the extract with an exogenous MYR source, which improves ITC bioavailability, was also performed.
Materials and methods
Chemicals
NADP+ and NADPH, GSH, oxidised glutathione (GSSG), GSSG reductase (GSSG-red), tert-butylhydroperoxide, dichlorophenolindophenol (DCPIP), diethylenetriaminepentaacetic acid pentasodium salt (DETAPAC), nitrotetrazolium blue chloride (NBT), xanthine, xanthine oxidase, bathocuproinedisulfonic acid, defatted bovine serum albumin and SOD were all purchased from Sigma Chemical Company. Glucose 6-phosphate, glucose 6-phosphate dehydrogenase and cytochrome c were from Boehringer–Mannheim. The enzyme MYR was extracted and isolated from the seeds of Sinapis alba L. as described previously(Reference Pessina, Thomas and Palmieri29) and modified by Bernardi et al. (Reference Bernardi, Finiguerra and Rossi30). The method was based on three chromatographic steps: affinity chromatography on Con-A-Sepharose; ion-exchange chromatography on SP-Sepharose FF; gel filtration chromatography on prepacked Superdex 200 Hiload 26/60. The chromatographic materials were supplied by GE Healthcare. The specific activity of the stock solution used in the present study was 60 U/mg soluble protein. Enzymatic activity was 30 U/ml and the solution was stored at 4°C in sterile distilled water until use. One MYR unit was defined as the amount of enzyme able to hydrolyse 1 μmol sinigrin/min at pH 6·5 and 37°C(Reference Palmieri, Leoni and Iori31).
All other chemicals were of the highest purity commercially available.
Plant source and extract preparation
Brassica oleracea L. var. acephala (DC) subvar. laciniata L. (Tuscan black cabbage) cv. 0D74 seeds were supplied by Suba & Unico, and stored in a dry and dark place at room temperature. Seeds were identified by a lot number and guaranteed by the producer for the quality and homogeneity of the product. Seeds were surface sterilised by soaking for 30 min in 1 % sodium hypochlorite and rinsed with tap water. Sprouting was allowed to occur by using an automatic sprouter Vita Seed (Suba & Unico) at room temperature in the dark with regular water supply. Thereafter, 7 d sprouts were gently washed with tap water, whole frozen and freeze-dried. Fine powdered freeze-dried sprouts (20 g) were extracted with boiling 70 % (v/v) ethanol (800 ml) for 5 min at 80°C using an Ultra-Turrax T25 homogeniser (IKA-Werk), and then centrifuged with a J2-MC centrifuge (Beckman) at 17 000 g for 30 min at 10°C. The supernatant volume was reduced 3-fold in a rotary evaporator, centrifuged and, finally, the extract was lyophilised (DLAB 500; Italian Vacuum Technology).
Determination of glucosinolate content
GL profile and quantification was assessed after desulfation as described previously(Reference De Nicola, Nyegue and Montaut32). Briefly, the fine powdered lyophilised TBCSE was suitably dissolved in ultra-pure water, and 1 ml volume containing 2–2·5 mg extract was loaded onto a mini-column filled with 0·6 ml of DEAE-Sephadex A-25 anion-exchange resin (GE Healthcare) conditioned with 25 mm-acetate buffer (pH 5·6). After washing with 3 ml of the same buffer, 200 μl (0·35 U/ml) of purified sulfatase(Reference Leoni, Iori and Haddoum33) were loaded onto the mini-column. After overnight incubation, desulfoglucosinolates were eluted with 4 ml ultra-pure water and injected into an Agilent 1100 HPLC system equipped with a photodiode array detector and an Inertsil ODS-3 column (250 × 3·0 mm, 5 μm particle size) thermostated at 30°C(Reference De Nicola, Nyegue and Montaut32). Identification of the peaks was performed on the basis of retention times and UV spectra of desulfoglucosinolate standard available in our laboratory. GL were quantified by using a calibration curve of pure desulfosinigrin solution (range 0·14–1·4 mm) and taking into account the reported relative proportionality factor for each individual desulfoglucosinolate(Reference Wathelet, Iori and Leoni34). In total, four independent HPLC determinations were performed.
Pretreatment of Tuscan black cabbage sprout extract solution with myrosinase and chemical analysis
TBCSE was dissolved in 0·01 m-PBS (pH 7·4) at 15 mg/ml, and ITC were produced by enzymatic hydrolysis of GL present in the extract, incubating at 37°C in the presence of 100 μl MYR (30 U/ml). After 10 min, the resulting ITC were analysed via GC–MS for profile determination and quantified as total ITC via HPLC analysis.
GC–MS analysis of isothiocyanates
The buffered solution containing ITC (1 ml) was withdrawn and extracted with dichloromethane (2 ml). After drying with K2SO4, the organic phase was analysed by GC–MS. GC–MS analyses of ITC were carried out using a Bruker Scion SQ Premium (Bruker Daltonics) equipped with a 30 m × 0·25 mm capillary column (HP-5MS). The flow rate of the carrier gas He was 1 ml/min. Temperature programming was from 60°C (hold 4 min) to 200°C at 10°C/min (hold 1 min). The temperature of the injector and of the detector was 180 and 280°C, respectively. All MS analyses were conducted in the electron impact (EI+) mode at 70 eV, the mass range was from 40 to 650 m/z and the chromatogram acquired in total ion current (TIC). The identification of ITC was assigned on the basis of retention times and mass spectra of ITC obtained by MYR-catalysed degradation of pure GL available in our laboratory.
HPLC analysis of total isothiocyanates
The buffered solution containing ITC was analysed as reported by Matera et al. (Reference Matera, Gabbanini and De Nicola35) by adding 40 μl of the sample to 600 μl of a solution of 1,2-benzenedithiol (10 mm) in i-PrOH and 500 μl of 0·1 m-phosphate buffer (pH 8·5). Solutions were incubated for 2 h at 65°C and then left to cool to room temperature, centrifuged 20 min at 13 000 g with a 5415C centrifuge (Eppendorf) and the supernatants (20 μl) were injected in HPLC. Analyses were performed with an Agilent 1100 system equipped with a photodiode array detector on a Zorbax SB-C18 column (150 × 4·6 mm, 3·5 μm) and thermostated at 30°C. Separation and quantification was achieved as described previously(Reference Matera, Gabbanini and De Nicola35). The pretreatment of TBCSE solution with MYR was performed twice and each cyclocondensation assay was done in triplicate.
Experimental design
Male Sprague–Dawley rats obtained from Harlan-Nossan, Italy, weighing 165 (sd 10) g, were housed under controlled conditions (12 h light–12 h dark cycle, 22°C, 60 % humidity). Throughout the study, they were treated in accordance with the National Academy of Sciences guidelines. After 1 week acclimatisation, all the animals were randomly divided into six groups of six animals each, and were treated as follows for twenty-one consecutive days: rats fed a regular diet (RD group; 18·7 % crude protein, 5·6 % crude fat, 4·5 % crude fibre, by Mucedola s.r.l.); rats fed a HFD (HFD group; 22·5 % crude protein, 23·1 % crude fat, 5·7 % crude fibre, 1·0 % cholesterol, by Charles River); rats fed a HFD and treated with TBCSE at 15 mg/kg BW without MYR pre-digestion (HFD+TBCSE min MYR − group); rats fed a HFD and treated with TBCSE at 150 mg/kg BW without MYR pre-digestion (HFD+TBCSE max MYR − group); rats fed a HFD and treated with TBCSE at 15 mg/kg BW with MYR pre-digestion (HFD+TBCSE min MYR+ group); rats fed a HFD and treated with TBCSE at 150 mg/kg BW with MYR pre-digestion (HFD+TBCSE max MYR+ group). The dose of 15 mg/kg BW contains an amount of GL comparable with the average daily GL intake in humans(Reference Steinbrecher, Nimptsch and Hüsing36). Before the treatment, TBCSE was dissolved in PBS at 1·5 and 15 mg/ml, and incubated at 37°C in the presence or absence of 0·3 or 3·0 units of MYR, respectively, for 10 min, in order to hydrolyse all present GL to ITC. Rats were treated orally, and the RD and HFD groups received an equal volume of vehicle (PBS) orally as well.
Weight gain and food intake measurements
BW gain and food intake were monitored daily. Food intake was estimated by subtracting the amount of food left in the cages from the total amount provided every day.
Tissue collection and preparation of subcellular fractions
Rats were fasted for 16 h before being killed in accordance with the approved Italian Ministerial procedures appropriate to the species. Liver was immediately removed from each rat, and processed separately. The S9 fraction (9000 g) was then prepared, and the post-mitochondrial supernatant was then centrifuged for 60 min at 105 000 g, after which the cytosolic fraction (supernatant) was collected and immediately frozen in liquid N2, and stored at − 80°C. The pellet was resuspended in 0·1 m-K2P2O7, 1 mm-EDTA (pH 7·4) and centrifuged again for 60 min at 105 000 g to give the final microsomal fraction. Washed microsomes were then suspended with a hand-driven Potter–Elvehjem homogeniser in a 10 mm-Tris–HCl buffer (pH 7·4) containing 1 mm-EDTA and 20 % (v/v) glycerol. The fractions were immediately frozen in liquid N2, stored at − 80°C and used within a week for enzymatic analyses. In this short period, the activities measured in conditions of V max and linearity of protein contents were stable(Reference Bauer, Corsi and Paolini37).
Protein concentration
Protein concentration was determined according to the method described by Lowry et al. (Reference Lowry, Rosenbrough and Farr38) as revised by Bailey(Reference Bailey39), using bovine serum albumin as a standard. Samples were diluted at least 200 times to provide a suitable protein concentration.
Phase II enzymes
Glutathione S-transferase
The incubation mixture for measuring glutathione S-transferase activity contained 1 mm-GSH, 1 mm-1-chloro-2,4-dinitrobenzene in methanol and 0·025 ml of sample in a final volume of 2·5 ml of 0·1 m-phosphate Na+/K+ buffer (pH 6·5). The product of the reaction of the thiol group of GSH with the electrophilic group of 1-chloro-2,4-dinitrobenzene was read at 340 nm (ɛ = 9·6 per mm× cm)(40).
Uridine diphosphate glucuronosyl transferase
The overall uridine diphosphate glucuronosyl transferase activity was determined kinetically using 1-naphthol as the substrate (final concentration 50 mm) by the continuous fluorimetric (excitation 293 nm, emission 335 nm) monitoring of 1-naphtholglucuronide production in the presence of 1 mm-uridine-5′ diphosphoglucuronic acid(Reference Mackenzie and Hänninen41).
Antioxidant enzymes
Catalase
The reaction was started in a quartz cuvette, containing 50 mm-potassium phosphate buffer and the cytosol sample, by adding 30 mm-H2O2. The decomposition of the substrate was measured at 240 nm, and CAT activity was expressed as μmol H2O2 consumed/min per mg protein, using a molar extinction coefficient of 43·6 per mm× cm(Reference Wheeler, Salzman and Elsayed42).
Oxidised glutathione reductase
GSSG-red activity was measured by adding 1·5 mm-NADPH to an assay cuvette containing 50 mm-potassium phosphate buffer, 1 mm-EDTA, cytosol sample and 20 mm-GSSG. The generation of NADP+ from NADPH, during the reduction of GSSG, was recorded at 340 nm for 5 min at 37°C. GSSG-red activity was calculated using the extinction coefficient of 6·22 per mm× cm, and expressed as μmol NADPH consumed/min per mg protein(Reference Wheeler, Salzman and Elsayed42).
Glutathione peroxidase
The incubation mixture for measuring the glutathione peroxidase (GSH-px) activity contained 50 mm-potassium phosphate, 1 mm-EDTA, cytosol sample, 10 mm-GSH, GSSG-red (2·4 U/ml) and 1·5 mm-NADPH. After incubation at 37°C for 5 min, and addition of tert-butylhydroperoxide, NADPH consumption was followed at 340 nm for 5 min at 37°C. Glutathione peroxidase activity was calculated using the extinction coefficient of 6·22 per mm× cm, and expressed as nmol NADPH consumed/min per mg protein(Reference Flohé and Günzler43).
NAD(P)H:quinone reductase
NQO1 activity was assayed spectrophotometrically at 600 nm by monitoring the reduction of the blue redox dye DCPIP to its colourless form, using NADPH as the hydrogen donor. The assay mixture contained 50 mm-Tris–HCI (pH 7·5), 1 mm-NADPH and 40 μm-DCPIP. Enzymatic activity was calculated using the extinction coefficient of DCPIP (22·1 per mm× cm), and expressed as nmol DCPIP reduced/min per mg protein(Reference Ernster44).
Superoxide dismutase
SOD activity was determined via the bathocuproinedisulfonic acid–NBT assay as described by Spitz & Oberley(Reference Spitz and Oberley45). Briefly, xanthine–xanthine oxidase was utilised to generate a superoxide flux. NBT reduction to blue formazan by O2− was monitored spectrophotometrically at 560 nm. The rate of NBT reduction in the absence of the sample was used as the reference rate. When cytosol samples (containing SOD activity) were added to the system, the rate of NBT reduction was gradually inhibited. The amount of inhibition was defined as a percentage of the reference rate of NBT reduction. One unit of activity was defined as that amount of protein necessary to decrease the reference rate to 50 % of maximum inhibition. The reaction mixture also contained CAT to remove H2O2 and DETAPAC to chelate metal ions interfering with the assay system, bathocuproinedisulfonic acid and defatted bovine serum albumin.
Serum biochemical analyses
Blood samples were collected from each animal during killing in heparinised tubes, and centrifuged for 15 min at 1500 g to obtain serum. The analyses of TC, TAG and NEFA were carried out on an automated analyser (Olympus AU 400) via colorimetric assays. TC was estimated using a cholesterol esterase enzymatic assay (OSR6116; Olympus). TAG were measured with an enzymatic mixture containing lipoprotein lipase, glycerol kinase and glycerol phosphate oxidase (OSR60118). NEFA were assayed by the ACS-ACOD method (NEFA-HR(2); Wako, Test Medical).
Statistical analysis
Statistical analysis was performed using Wilcoxon's rank method to evaluate significant differences between the experimental groups(Reference Box and Hunter46).
Results
Glucosinolate content in Tuscan black cabbage sprout extract
Individual and total contents of GL in TBCSE are reported in Table 1. The extract resulted to be highly enriched in GL, particularly in two aliphatic GL with a sulphur-containing side chain which accounted for 89 % on the base of the weight, with predominant glucoraphanin (4(R)-methylsulfinylbutyl GL), the precursor of sulforaphane, followed by glucoerucin (4-methylsulfanylbutyl GL), the precursor of erucin. 4-Hydroxy-glucobrassicin, glucobrassicin, 4-methoxy-glucobrassicin and neoglucobrassicin were also detected. It is worth noting that this local black cabbage, a kale type grown in Tuscany, differs from other kales since neither progroitin, nor sinigrin was detected, which is in agreement with the results recently reported for five domestic cultivars of black kale from Italy(Reference Sasaki, Neyazaki and Shindo47). This occurrence makes Tuscan black cabbage an ideal source of glucoraphanin; in fact, a gram-scale production of this GL has recently been set up starting from the seeds of this kale variety, according to a procedure developed at CRA-CIN of Bologna, Italy(Reference Abdull Razis, Bagatta and De Nicola24).
Table 1 Glucosinolate content of Tuscan black cabbage (Brassica oleracea L. var. acephala (DC) subvar. laciniata L.) sprout extract (Mean values and standard deviations of four independent HPLC determinations)
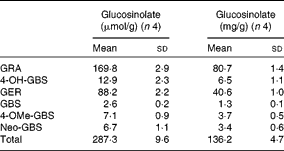
GRA, glucoraphanin; 4-OH-GBS, 4-hydroxy glucobrassicin; GER, glucoerucin; GBS, glucobrassicin; 4-OMe-GBS, 4-methoxy-glucobrassicin; Neo-GBS, 1-methoxy-glucobrassicin.
Pretreatment of Tuscan black cabbage sprout extract with myrosinase and chemical analysis
GL contained in TBCSE were hydrolysed by the exogenous MYR treatment, and the resulting ITC were identified and quantified. Identification was achieved by GC–MS analysis after dichloromethane extraction of the buffered hydrolysate. As a result, two ITC, erucin and sulforaphane, were detected (Fig. 1), confirming the presence of the precursors glucoraphanin and glucoerucin in the extract. The total ITC were determined according to the well-established cyclocondensation assay(Reference Zhang48), and quantified by HPLC. We treated 15 mg/ml of TBCSE containing 3·1 (sd 0·1) μmol/ml of GL (glucoraphanin and glucoerucin) and the results gave 3·3 (sd 0·1) μmol/ml of total ITC, showing a quantitative conversion of GL into ITC mediated by MYR in PBS (pH 7·4). Only aliphatic GL accounted for the level of conversion, thus indole GL are known to be hydrolysed into highly unstable ITC and are spontaneously transformed.
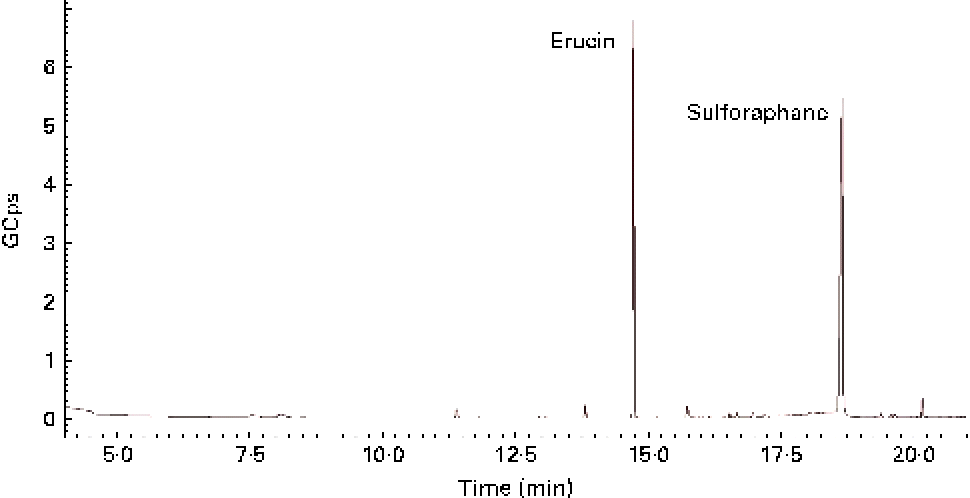
Fig. 1 GC–MS chromatogram of isothiocyanate profile in Tuscan black cabbage sprout extract, after incubation with exogenous myrosinase.
Body-weight gain, liver weight and food intake
In the present study, BW gain and liver weight were significantly higher in rats fed the HFD when compared with the controls (P< 0·01; Table 2). TBCSE lowered BW in all the treated groups with respect to the HFD group. At 15 mg/kg BW, both in the presence and absence of exogenous MYR, the extract was able to significantly counteract the BW gained by the HFD (P< 0·01). After 21 d of TBCSE administration, liver weight was also lowered, with respect to the HFD group, with a statistically significant reduction only by the lower dose of TBCSE with MYR (P< 0·01). Food intake was significantly lower in the HFD group with respect to the controls (P< 0·01), as well as in the HFD+TBCSE MYR − (150 mg/kg BW) and the HFD+TBCSE MYR+(15 mg/kg BW) groups, where a modest but statistically significant reduction (P< 0·01) was recorded (Table 2).
Table 2 Changes in body weight, liver weight and food intake of male Sprague–Dawley rats fed a regular diet (RD), a high-fat diet (HFD) or HFD+Tuscan black cabbage sprout extract (TBCSE) (Mean values and standard deviations of six measurements performed on six rat samples for each studied group)

MYR, myrosinase.
Mean values were significantly different compared with the HFD group (Wilcoxon's rank method): * P< 0·05, ** P< 0·01.
Mean values were significantly different compared with the RD group (Wilcoxon's rank method): † P< 0·05, †† P< 0·01.
Serum lipid profile
As shown in Table 3, feeding the HFD resulted in an increase of serum lipid profile: TC (P< 0·05), TAG (P< 0·05) and NEFA (P< 0·01) were significantly elevated compared with the RD group. On the contrary, the TBCSE treatment produced a generalised decrement in the measured serum lipid parameters (TC, TAG and NEFA) with respect to the control rats: TBCSE MYR − significantly reduced TC (P< 0·01) at 15 mg/kg BW and TAG at 150 mg/kg BW, while NEFA were reduced by both dosages of TBCSE MYR − (P< 0·01), and by the lower dosage with MYR (P< 0·05).
Table 3 Changes in lipid profiles in the serum of male Sprague–Dawley rats fed a regular diet (RD), a high-fat diet (HFD) or HFD+Tuscan black cabbage sprout extract (TBCSE) (Mean values and standard deviations of six measurements performed on six rat samples for each studied group)

MYR, myrosinase; BW, body weight.
Mean values were significantly different compared with the HFD group (Wilcoxon's rank method): * P< 0·05, ** P< 0·01.
Mean values were significantly different compared with the RD group (Wilcoxon's rank method): † P< 0·05, †† P< 0·01.
Hepatic antioxidant enzymes
The HFD decreased the activity of all the antioxidant enzymes tested (P< 0·01), except the GSH-px activity, compared with the RD group (Table 4). The activity of CAT was significantly increased by the extract at 15 mg/kg BW without MYR (4·2 %, P< 0·05) and with MYR (22·4 %, P< 0·01). NQO1 was significantly enhanced by TBCSE up to 141·7 % (P< 0·01) by the higher dosage without MYR, and up to 33·5 % with MYR (P< 0·01). The lower dosage without MYR also up-regulated the activity of NQO1 (up to 8·7 %, P< 0·01). A decrement (6·5 % loss, P< 0·05) in GSH-px activity was recorded in the HFD+TBCSE MYR − (15 mg/kg BW) group, whereas significant up-regulations were observed in both HFD+TBCSE MYR+ groups (up to 14·2 %, lower dosage, P< 0·01 and up to 9·9 %, higher dosage, P< 0·01). GSSG-red was also influenced by the treatment with TBCSE: up to 12·2 % (P< 0·01) by the higher dosage without MYR and up to 7·6 % (P< 0·01) by the lower dosage with MYR. In the HFD+TBCSE MYR − groups, the activity of SOD was induced by both the lower (up to 16·0 %, P< 0·01) and the higher (up to 47·0 %, P< 0·01) dosages. A decrease in SOD activity was observed (17·9 % loss, P< 0·05) in the HFD+TBCSE MYR+ group (150 mg/kg BW) as well.
Table 4 Changes in antioxidant enzymatic activities in the hepatic citosol of male Sprague–Dawley rats fed a regular diet (RD), a high-fat diet (HFD) or HFD+Tuscan black cabbage sprout extract (TBCSE) (Mean values and standard deviations of six measurements performed on six rat samples for each studied group)

MYR, myrosinase; BW, body weight; SOD, superoxide dismutase.
Mean values were significantly different compared with the HFD group (Wilcoxon's rank method): * P< 0·05, ** P< 0·01.
Mean values were significantly different compared with the RD group (Wilcoxon's rank method): † P< 0·01.
Hepatic phase II enzymes
The HFD reduced significantly (P< 0·01) the activities of the tested phase II enzymes, compared with the RD group, as indicated in Table 5. TBCSE MYR− at 150 mg/kg increased the activity of glutathione S-transferase up to 11·9 % (P< 0·01), while the treatment with MYR at 15 mg/kg up to 6·7 % (P< 0·05). Uridine diphosphate glucuronosyl transferase activity was up-regulated by the extract administration in a dose-dependent manner: TBCSE MYR− induced up to 17·4 % (P< 0·05), and 56·2 % (P< 0·01), at the lower and the higher dosages, respectively, whereas TBCSE MYR+ increased up to 52·6 % (P< 0·01) at 15 mg/kg and up to 72·3 % (P< 0·01) at 150 mg/kg.
Table 5 Changes in phase II enzymatic activities in hepatic microsomes of male Sprague–Dawley rats fed a regular diet (RD), a high-fat diet (HFD) or HFD+Tuscan black cabbage sprout extract (TBCSE) (Mean values and standard deviations of six measurements performed on six rat samples for each studied group)

UDP, uridine diphosphate; MYR, myrosinase; BW, body weight.
Mean values were significantly different compared with the HFD group (Wilcoxon's rank method): * P< 0·05, ** P< 0·01.
Mean values were significantly different compared with the RD group (Wilcoxon's rank method): † P< 0·01.
Discussion
Several lines of evidence suggest the presence of antioxidative and hypolipidaemic compounds in Brassica vegetables. In addition, it has been shown that a diet rich in fat increases ROS production and serum lipids, and diminishes the antioxidant defence system.
A potential mechanism for the generation of free radicals may be the activation of β-adrenergic receptors that in turn could mediate lipolysis and NEFA production, able to uncouple mitochondrial phosphorylation and further generate ROS(Reference Bhandari, Kumar and Khanna11). Moreover, a suppression of the mitochondrial respiratory chain may result in NEFA β-oxidation by acyl-CoA oxidase in peroxisome, and in ω-oxidation by cytochrome P450 2E1 in the endoplasmatic reticulum, with subsequent additional formation of ROS(Reference Matsuzawa-Nagata, Takamura and Ando49).
The present study aimed to explore principally the preventive role of TBCSE intake against alterations by a HFD in the rat. The influence on antioxidant as well as on phase II enzymes, and the lipid- and weight-lowering properties of this high-GL-containing TBCSE, were the main biological endpoints investigated.
Feeding a HFD to rats induced hyperlipidaemia, which was confirmed by the higher serum lipid profile compared with rats fed a RD. The findings of the present study revealed that TBCSE supplementation was able to lower serum lipids such as TC, TAG and NEFA, increased by the HFD, particularly at both the lower and higher dosages without MYR. Moreover, TBCSE was effective in reducing rat BW gain and liver weight increase, mostly at the lower dosages considered. Although a modest reduction of food intake was recorded in some experimental groups receiving TBCSE, this does not appear to be the principal responsible for the beneficial observations concerning the BW and lipid-lowering effects, rather due to the extract properties.
In the HFD group, liver antioxidant enzymes (CAT, NQO1, GSSG-red and SOD) were significantly down-regulated when compared with the control RD group. This is in accord with the literature, as feeding a HFD to experimental animals depresses the antioxidant system of cells(Reference Yoshinari, Takagi and Yoshimasa12, Reference Daniel, Mathew and Devi50, Reference Anila and Vijayalakshmi51). These decrements in antioxidant enzymes might be possibly ascribed to their increased exploitation to counteract the excessive liver ROS and lipid peroxidation production, which might further damage cellular membranes and induce changes in intracellular enzymes(Reference Bahramikia and Yazdanparast5, Reference Yazdanparast, Bahramikia and Ardestani10).
GSH-px was instead not affected significantly by the HFD with respect to the RD group. Accordingly, another study has detected no differences in the expression level of hepatic GSH-px between mice fed a HFD and controls. A different molecular mechanism of regulation in response to lipid excess may be thus supposed for this enzyme, in comparison with other antioxidant enzymes(Reference Matsuzawa-Nagata, Takamura and Ando49).
Administration of TBCSE to rat fed a HFD, either in the presence or absence of exogenous MYR, restored hepatic enzymatic antioxidant enzyme capability towards the basal level or even higher than the RD group. The most responsive enzymes were as follows: CAT by the lower dosage with MYR; NQO1 by the higher dosage without MYR; GSH-px by both MYR+ dosages; GSSG-red and SOD by the higher dosage without MYR. These recovering outcomes could be attributed to the high content of flavonoids, phenolics and organosulphur compounds within the extract, which act as the precursor of GSH, possess antioxidant and free-radical-scavenging properties and enhance the antioxidant status(Reference Bahramikia and Yazdanparast5, Reference Abdel-Wahhab and Aly18).
Changes in phase II enzymes have been described in genetically engineered or nutritionally induced animal models of obesity(Reference Bahramikia and Yazdanparast5). For example, a reduction in the activity of the hepatic phase II enzymes (e.g. Ugt1a1, Sult1a1 and Sultn) was described in HFD-fed mice(Reference Ghose, Omoluabi and Gandhi13). The activities of glutathione S-transferase and uridine diphosphate glucuronosyl transferase were significantly suppressed by the HFD, while TBCSE was efficient in counteracting this down-regulation, in most of the experimental groups, although without a clear distinction between the treatments with or without MYR pre-digestion. Phase II enzymes are primarily microsomal membrane proteins, therefore any changes in the fatty acid composition of membrane phospholipids may affect the fluidity of membranes and potentially disturb the activity of these embedded enzymes(Reference Chen, Yang and Tsai52). Dietary fatty acids might thus represent a cause of phase II enzyme deregulation, and ultimately of an altered pharmacological response to therapeutic drugs or even of drug toxicity(Reference Wilkinson53). TBCSE was shown to counteract phase II enzyme modifications by the HFD, thus potentially able to prevent potential hazardous perturbations of drug metabolism, which may occur in hyperlipidaemic or obese condition(Reference Ghose, Omoluabi and Gandhi13). This is the first scientific study to describe the promising efficacy of TBCSE in the prevention of HFD-related conditions. The possible underlying mechanisms by which Brassicaceae exert lipid-lowering properties are not yet completely elucidated. Previous studies have reported that the hypolipidaemic properties of certain herbal extracts are mediated by inhibiting fat intestinal absorption and by stimulating lipid catabolism(Reference Sankhari, Thounaojam and Jadeja54). In addition, it has been reported that broccoli sprout extract affects the expression of genes involved in lipid homeostasis in the liver(Reference Rodríguez-Cantú, Gutiérrez-Uribe and Arriola-Vucovich14, Reference Fimognari, Turrini and Ferruzzi55). It is reasonable therefore that similar events might also account for the hypolipidaemic effects of TBCSE described here. Yet, it is not completely clear which class of phytochemicals within Brassica plants is mainly responsible for the health effects. The total GL to ITC conversion, experimentally demonstrated, by an exogenous source of MYR does not appear to confer additional benefits, compared with the extract as such. Indeed, no substantial distinctions between the extract containing a mixture of GL and ITC (these latter yielded by intestinal microflora), or only ITC, were recorded. Overall, the total effect of TBCSE without preliminary GL hydrolysis appears to be as efficacious as the administration after incubation with MYR, and for some parameters even superior as observed in the analysis of glutathione S-transferase, NQO1, SOD activities and serum lipid profile. Thus, also the additive and/or synergistic interactions between GL and ITC are likely to confer significant health advantages against the deleterious alterations of a hyperlipidaemic diet.
Emerging data further suggest that GL as well as ITC interact synergistically with other elements of the food matrix to exert their biological functions and strengthen the significance of the food matrix on the bioactivity of phytochemicals(Reference Rodríguez-Cantú, Gutiérrez-Uribe and Arriola-Vucovich14).
Hence, the intake of vegetable extracts as a dietary supplement, better representing entire raw greens, seems to be a more health-protective alimentary strategy, compared with the use of a high dose of single phytochemicals. In this regard, it has recently been reported that exposure to high concentrations of phytochemicals from Brassica also does not necessarily lead to a high induction of detoxifying enzymes(Reference Veeranki, Bhattacharya and Marshall56).
In summary, TBCSE emerges to be an effective nutraceutical, capable of counteracting alterations associated with a diet rich in fat, even at relatively low dosages.
Acknowledgements
All sources of financial and material support were provided by a grant from the Italian Ministry of Education, University and Research. S. M., D. C., A. S. and M. P. designed the study. S. M. conducted the study in vivo and data analysis. G. R. D. N. and L. L. prepared and analysed the extract. S. M. wrote the manuscript, with the cooperation of D. C., A. S., M. P. and G. R. D. N. All authors discussed, edited and approved the final version. The authors declare that there are no conflicts of interest.