Alkaline phosphatase (ALP; EC 3.1.3.1) is distributed in wide range of tissues, including the intestine, liver, kidney, bone and leukocytes( Reference McComb, Bower and Posen 1 ). In humans, ALP is divided into four types: tissue non-specific ALP (TNSALP, liver/bone/kidney), intestinal ALP, placental ALP and germ cell ALP( Reference Goseki-Sone, Orimo and Iimura 2 ). Rat ALPs are classified into TNSALP and intestinal ALP( Reference Haraikawa, Sogabe and Tanabe 3 ). Tissue non-specific ALP plays a role in bone mineralisation( Reference Goseki-Sone, Orimo and Iimura 2 ). Recently, several reports showed that small-intestinal ALP had a protective effect against inflammatory diseases( Reference Goldberg, Austen and Zhang 4 , Reference Kaliannan, Hamarneh and Economopoulos 5 ). Goldberg et al. ( Reference Goldberg, Austen and Zhang 4 ) reported that intestinal ALP detoxified endotoxins (lipopolysaccharides) and protected from bacterial invasion. Oral intestinal ALP supplementation was demonstrated to prevent the metabolic syndrome in mice fed a high-fat (HF) diet( Reference Goldberg, Austen and Zhang 4 ). It has been shown that several nutrients, such as vitamins A, K and D and lactose, enhanced small-intestinal ALP activity(3, Reference Nikawa, Rokutan and Nanba 6 –8).
Recently, our study has demonstrated for the first time that dietary fermentable fibre, glucomannan, elevates colonic ALP activity and the expression of intestinal alkaline phosphatase (IAP-I), an ALP gene expressed throughout the intestine, without affecting the small-intestinal ALP in rats fed an HF diet(9). In researching anti-colitis effect, Morita et al. Reference Morita, Tanabe and Sugiyama (10) showed that dietary-resistant starch, a fermentable carbohydrate, increased colonic ALP activity in rats, although the mechanism and the physiological significance were not investigated. Until now, the available data on the effects of dietary composition or nutrients on colonic ALP activity are much less extensive than on small-intestinal ALP activity, and little information exists on whether food ingredients affect colonic ALP gene expression.
We have recently reported that colonic ALP activity was correlated with faecal mucins and caecal organic acidsReference Okazaki and Katayama (9) . Mucins are the primary components of the mucus gel and play an important role in the maintenance of gut barrier function. Mucins protect mucosal surface against pathogenic micro-organisms and antigensReference Deplancke and Gaskins (11) . The high production of organic acids has been associated with a lower risk of colon diseasesReference Nyman (12) . Taken together, it is possible that the fermentable non-digestible carbohydrates may commonly elevate colonic ALP activity and the gene expression with increasing mucins and microbial fermentation, which is associated with increased production of protective factors for gut epithelial homoeostasis.
Dietary non-digestible oligosaccharides, which are some kinds of fermentable carbohydrate, increase intestinal mucins, organic acid (n-butyrate) and Bifidobacterium, suggesting being beneficial for intestinal barrier and colon health( Reference Kudoh, Shimizu and Ishiyama 13 – Reference Ehara, Izumi and Tsuda 17 ). Therefore, we hypothesised that dietary non-digestible oligosaccharides would increase colonic ALP activity and gene expression as well as improve intestinal barrier function, fermentation and microbiota of HF-fed rats. The objective of this study was to evaluate the effects of dietary oligosaccharides on colonic ALP activity and the gene expression and to investigate the relationship between its activity and production or status of protective factors for gut epithelial homoeostasis (faecal mucins, caecal organic acids: index of gut fermentation and faecal microbiota) in rats fed an HF diet. HF diet alters intestinal environment and is generally known to be a risk factor for colon disease. Sprague–Dawley rats are widely used in HF diet studies. Thus, we selected this strain to investigate colonic luminal environment under the HF diet condition.
Methods
Animals
Experimental procedures were reviewed and approved by the ethics committee for Animal experimentation of the Fuji Women’s University (approved no. 2016-1). All animal experiments were conducted according to the Guidelines for Animal experiments of the Fuji Women’s University, ‘Japanese Act on Welfare and Management of Animals’ (law no. 105 of 1 October 1973; recent revision: law no. 38 of 12 June 2013) and ‘Standards Relating to the Care and Management of Laboratory Animals and Relief of Pain’ (Ministry of the Environment in Japan, notification no. 88, 28 April 2006; recent revision: notification no. 84, 30 August 2013).
A total of 42 male Sprague–Dawley rats (4 weeks of age, weighing 90–100 g) were purchased from Japan SLC Inc. The rats were housed in individually in suspended stainless steel cages with wire mesh bottoms in a room with controlled temperature (23–24°C), relative humidity (55–65 %) and light/dark cycle (light, 08.00–20.00 hours) in the Fuji Women’s University animal facility.
Groups and treatments
Following ad libitum access to a non-purified commercial rodent powder diet (CE-2, CLEA Japan; containing 9·3 % moisture, 25·1 % crude protein, 4·8 % crude fat, 4·2 % crude fibre, 6·7 % crude ash and 50·0 % N-free extract; energy, 1·44 MJ/100 g) and water for 4 d, the rats were randomised by weight and assigned to four groups (Experiment 1, n 6/group): control, fructo-oligosaccharides (FOS), galacto-oligosaccharides (GOS) and isomalto-oligosaccharides (IMOS) or to three groups (Experiment 2, n 6/group): control, raffinose (RAF) and lactulose (LAC). Composition of the basal diet was as follows: lard, 30 %; casein, 20 %; l-cystine, 0·3 %; cellulose, 5·0 %; sucrose, 30 %; vitamin mixtureReference Reeves, Nielsen and Fahey (18) , 1·0 %; salt mixtureReference Reeves, Nielsen and Fahey (18) , 3·5 %; choline bitartrate, 0·25 % and maize starch, 9·95 % (Table 1). In the oligosaccharides diet, 4·0 % FOS, GOS or IMOS (Wako Pure Chemical Industries Ltd, respectively) was added in Experiment 1, and the same level of RAF (Wako Pure Chemical Industries Ltd) or LAC (Nichie Inc.) was added in Experiment 2 (Table 1). FOS, GOS, RAF and LAC are non-digestible oligosaccharides, and IMOS is some digestible oligosaccharide. The level of dietary fibre in the oligosaccharides-supplemented diets was adjusted by reducing dietary cellulose. The animals had free access to the experimental diets and deionised water. Food intake and body weight were measured daily. The welfare and general health status of the individual animals were checked every day throughout the experimental period. No adverse events were observed during this study.
Table 1 Composition of the experimental diets (%, w/w)

* Reeves et al. ( Reference Chua and Shrago 18 ).
† In Experiment 1, fructo-oligosaccharides, galacto-oligosaccharides or isomalto-oligosaccharides were used; in Experiment 2, raffinose or lactulose were used.
Procedures for collecting samples
Faecal pellets were collected during the last 3 d of feeding, stored at –20°C and then freeze-dried and milled. The powdered faeces were stored at –30°C until ALP, mucin, IgA and microbiota analyses. At the end of the feeding period, the rats were anaesthetised with 3·0 % isoflurane and euthanised. Whole blood was collected from the abdominal aorta. The serum was separated by centrifugation at 3000 g for 15 min and stored at –80°C. The caecum was removed, weighed, frozen immediately with liquid N2, and stored at –80°C until organic acid analysis. The small intestine and colon were removed, opened longitudinally, washed with saline to remove residual luminal contents, and weighed. The small intestine was divided into three regions. We took 3 cm from the pylorus as the duodenum and then separated the remaining part into jejunum and ileum. Portions of the duodenum, jejunum, ileum and colon were immediately immersed in RNA later (Ambion), stored at 4°C overnight and then stored at –80°C until total RNA was isolated. The remaining portions of the duodenum, jejunum, ileum and colon were frozen immediately with liquid N2 and stored at –80°C until ALP analysis.
Alkaline phoshatase activity
The mucosa of the duodenum, jejunum, ileum and colon and faeces were homogenised with 10 mm TRIS-buffered saline containing 1 % Triton X-100 (pH 7·3) and 1 mm phenylmethylsulfonylfluoride. The homogenate was centrifuged 7000 g at 4°C for 15 min( Reference Sogabe, Maruyama and Goseki-Sone 7 ). The supernatant was used as an enzyme-enriched extract. ALP activity was measured using a Lab Assay ALP Kit (Wako Pure Chemicals).
Alkaline phoshatase inhibitor assay
To investigate the enzymatic properties of ALP in the colon, an inhibition experiment was performed with l-phenylalanine, l-homoarginine and levamisole. l-Phenylalanine is a strong inhibitor of intestinal-type ALP, l-homoarginine inhibits bone and liver-derived ALP and levamisole inhibits TNSALPReference Chua and Shrago (19) . The ALP activity of pooled colonic mucosa was determined in the presence of l-phenylalanine (20 mm), l-homoarginine (20 mm) and levamisole (1 mm)( Reference Chua and Shrago 19 ). Protein concentration was determined using a Qubit Protein Assay Kit and Qubit 2.0 fluorometer (Life Technologies).
RNA extraction and gene expression assays
Total RNA was extracted from the preserved experimental samples using the Nucleospin RNA Kit (Macherey-Nagel), which included the elimination of genomic DNA with DNAse. Complementary DNA (cDNA) was synthesised from purified RNA using PrimeScript RT Master Mix (Takara Bio Inc.). In rats, two kinds of ALP isozymes were identified: intestinal ALP and TNSALP. The alkaline phosphatase, liver/bone/kidney (Alpl) gene encodes TNSALP, and the IAP-I and intestinal alkaline phosphatase 3 (Akp3) genes encode intestinal ALP. The IAP-I gene is expressed throughout the intestine, and the Akp3 gene is expressed specifically in the duodenumReference Narisawa, Hoylaerts and Doctor (20) . Therefore, the cDNA for Akp3, IAP-I and Alpl was quantified. The primer sets for Akp3 (primer ID: RA042710) and Alpl (primer ID: RA041418) were purchased from Takara Bio Inc. The primers set for IAP-I included the following two primers: 5′-CCTGGAGCCCTACACCGACT-3′ (forward) and 5′-GCCAGCGTTGAGACCCTTGG-3′ (reverse)Reference Harada, Koyama and Kasahara (21) . In this study, the cDNA for mucin 2 (Muc2) and mucin 3 (Muc3), both of which are mucin genes, was also quantified. The primer sets for Muc2 were 5′-AAGCCAGATCCCGAAACCAT-3′ (forward) and 5′-ATGGCCCCATTCACAACTGCC-3′ (reverse) and for Muc3 were 5′-GGTACAGCGGTGAAAACT-3′ (forward) and 5′-CATGGGGAAATCTCAACG-3′ (reverse)Reference Tsuboi, Kim and Paparella (22) . Real-time PCR was completed using SYBR Premix Ex Taq II (Takara Bio Inc.), and samples were amplified in a Light Cycler 480 System II (Roche Applied Science) with the following cycle conditions: 95°C for 30 s followed by forty-five cycles of 95°C for 5 s and 60°C for 30 s. Melting curve analysis was performed after amplification to distinguish the targeted PCR product from the non-targeted PCR product. The expression of the target genes IAP-I, Akp3, Alpl, Muc2 and Muc3 were normalised to glyceraldehyde-3-phosphate dehydrogenase (GAPDH) (primer ID: RA015380; Takara Bio Inc.), which was used as an endogenous control geneReference Dharmani Poonam Strauss, Ambrose and Allen-Vercoe (23) .
Faecal mucins and IgA
Mucins were extracted by the methods of Bovee-Oudenhoven et al. Reference Bovee-Oudenhoven, Termont and Heidt (24) with some modificationsReference Morita, Tanabe and Sugiyama (10) . Faecal sample was suspended in 20 volumes of PBS. The suspension was immediately heated to 95°C for 10 min and incubated for 90 min at 37°C. After centrifugation at 20 000 g for 15 min at 4°C, an equal volume of 0·4 m acetate buffer (pH 4·75) was added to the supernatant and incubated with 10 μl of amyloglucosidase for 20 min at 50°C. After the mixture was cooled, ice-cooled absolute ethanol was added to a final concentration of 60 % by volume. The samples were allowed to precipitate overnight at –30°C and centrifuged at 2000 g for 10 min at 4°C and finally dissolved in 2 ml of PBS. Mucins were determined using a fluorimetric assay according to the method of Crowther & WetmoreReference Crowther and Wetmore (25) with N-acetylgalactosamine (Sigma) as a standard.
Faeces were suspended in 40 volumes of PBS containing 50 mmol/l EDTA, 100 mg/l trypsin inhibitor and 1 mmol/l phenylmethylsulfonyl and incubated for 2 h at 4°C. The suspensions were vigourously mixed and centrifuged at 9000 g for 10 min at 4°C, and the supernatants were frozen at –80°C until IgA quantitation by ELISA technique. The total IgA concentration in the faeces was measured using an ELISA quantitation kit (Bethyl Laboratories)Reference Okazaki, Tomotake and Tsujimoto (26) .
Caecal organic acids
The pH of caecal digesta was measured directly using a compact pH metre (B-712; Horiba). Caecal organic acids were quantified using the internal standard method and HPLC (L-2130; Hitachi) system equipped with an Aminex HPX-87H ion exclusion column (7·8 mm inside diameter (i.d.)×30 cm; Bio-Rad) attached to a micro-guard column (Cation H Cartridge, 4·6 mm i.d.×3 cm; Bio-Rad)Reference Fernandes, Rao and Wolever (27) . Briefly, 500 mg of caecal digesta was homogenised in 5 ml of 50 mm H2SO4 containing 10 mm 2,2-dimethyl butyric acid (Wako Pure Chemicals Co. Ltd) as an internal standard. Next, the mixture was centrifuged at 17 000 g for 20 min at 2°C. The supernatant was ultrafiltered using an Amicon Ultra-4 Centrifugal Filter Device with a 3-kDa cut-off (Merck-Millipore Ltd), and the filtrate was analysed by HPLC (column at 60°C)Reference Chen and Lifschitz (28) . The mobile phase (5 mm H2SO4) was delivered at a flow rate of 0·7 ml/min using a Hitachi pump L-2130 (Hitachi). Organic acids were detected at 210 nm with a variable wavelength detector (L-2400; Hitachi)Reference Fernandes, Rao and Wolever (27) .
Faecal microbiota analysis using quantitative PCR
Bacterial genomic DNA was isolated from faeces using the QIAamp DNA Stool Mini Kit (Qiagen). Bacterial species were quantified by real-time quantitative PCR using a Light Cycler 480 System II (Roche Applied Sciences) as described previouslyReference Yang, Sitanggang and Kato (29) . After initial denaturation at 95°C for 30 s, 40 PCR cycles were done with denaturation at 95°C for 5 s, annealing at 55°C (total bacteria, Lactobacillus spp. Bifidobacterium spp., Clostridium coccoides, Clostridium leptum and Bacteroides) for 30 s and extension at 72°C for 15 s (total bacteria, Lactobacillus spp., Bifidobacterium spp. and Bacteroides) or 1 min (C. coccoides and C. leptum). Melting curve analysis was performed after amplification to distinguish the targeted PCR product from the non-targeted PCR product. Data were analysed by the second derivative maximum method using LightCycler 480 Basic Software. The relative abundances of the microbial populations are expressed as the proportions of the total bacterial 16S ribosomal DNA (rDNA) gene.
Statistical analyses
Power analysis was performed by using G*Power 3.1.9.2 (Franz Faul, Universität Kiel) software to determine the sample size needed to detect significant differences among the groups. In Experiment 1, statistical power of 0·80 (80 %) was obtained by estimating twenty-four rats (six animals per group) when the effect size was 0·74 and the significant level was 0·05. In Experiment 2, statistical power of 0·80 (80 %) was obtained by estimating eighteen rats (six animals per group) when the effect size was 0·81 and the significance level was 0·05. The sample size was similar to those in our previous studyReference Okazaki and Katayama (9) . Data were expressed as means with their standard errors. For data with a normal distribution, we used one-way ANOVA. Data without a normal distribution were analysed using the non-parametric Kruskal–Wallis test. Tukey’s post hoc tests were performed when a significant effect was detected using a one-way ANOVA. The Steel–Dwass post hoc test was performed when a significant effect was detected using the Kruskal–Wallis test. Some data were subjected to a Spearman rank correlation analysis (R s; Spearman rank correlation coefficient). The data analysis was performed using BellCurve for Excel software (Social Survey Research Information Co. Ltd). P values <0·05 were considered statistically significant.
Results
Body weight and food intake
In Experiment 1, the final body weights and food intake did not differ between the four groups (Table 2). The FOS diet, GOS diet and IMOS diet had no significant effect on the weights of colon and small-intestinal tissue. Faecal dry weight was significantly decreased in the FOS, GOS and IMOS groups (P<0·05).
Table 2 Body weight, food intake, colon and small-intestinal weight and faecal dry weight in rats fed a high-fat diet containing oligosaccharides (Mean values with their standard errors; n 6)

FOS, fructo-oligosaccharides; GOS, galacto-oligosaccharides; IMOS, isomalto-oligosaccharides; RAF, raffinose; LAC, lactulose.
a,b In each experiment, mean values within a row with unlike superscript letters were significantly different (P<0·05; Tukey post hoc test).
In Experiment 2, the final body weight, food intake, colonic and small-intestinal tissue weights were not different between the three groups (Table 2). When compared with the control group, faecal dry weight was significantly lower in the RAF and LAC groups. In each experiment, there were no adverse events in any group.
Colonic and faecal alkaline phoshatase activity
In Experiment 1, colonic ALP activity was markedly elevated in the FOS group (P<0·05, Fig. 1(a)) compared with the control group, with a similar trend observed in the GOS group. Faecal ALP activity was significantly elevated by FOS and GOS intake (P<0·05, Fig. 1(c)). On the other hand, the IMOS group did not affect the colonic and faecal ALP activity. As shown in Table 3, the colonic ALP preparations were strongly inhibited by l-phenylalanine but not l-homoarginine or levamisole. The duodenum and jejunum activities of ALP did not differ among the four groups (Table 4). The ileum ALP activity was significantly increased in the GOS group (P<0·05), and similar trend was observed in the FOS and IMOS groups. Serum ALP activity did not differ among the four groups (data not shown).

Fig. 1 Colon and faecal alkaline phosphatase (ALP) activities in rats fed a high-fat diet containing oligosaccharides in Experiment 1 (a and c) and Experiment 2 (b and d). Values are means (n 6), with their standard errors represented by vertical bars. (a, c) Control (), fructo-oligosaccharides (
), galacto-oligosaccharides (
) and isomalto-oligosaccharides (
). (b, d) Control (
), raffinose (
) and lactulose (
). a,b Mean values with unlike letters were significantly different (P<0·05; Steel–Dwass post hoc test).
Table 3 Inhibitory effects of l-phenylalanine, l-homoarginine and levamisole on colonic alkaline phosphatase (ALP) activity in rats fed a high-fat diet containing oligosaccharidesFootnote *

FOS, fructo-oligosaccharides; GOS, galacto-oligosaccharides; IMOS, isomalto-oligosaccharides; RAF, raffinose; LAC, lactulose.
* Values are pooled data from six animals in each group.
† Remaining ALP activity with inhibitors is expressed as a percentage of non-treated controls (non-inhibitor).
Table 4 Alkaline phosphatase (ALP) activities and relative gene expression of ALP (IAP-I, Akp3, Alpl) of duodenum, jejunum and ileum in rats fed a high-fat diet containing oligosaccharides (Mean values with their standard errors; n 6)

IAP-I, intestinal alkaline phosphatase; Akp3, intestinal alkaline phosphatase 3; Alpl, alkaline phosphatase, liver/bone/kidney; FOS, fructo-oligosaccharides; GOS, galacto-oligosaccharides; IMOS, isomalto-oligosaccharides; RAF, raffinose; LAC, lactulose.
a,b In each experiment, mean values within a row with unlike superscript letters were significantly different (P<0·05; Tukey post hoc test).
In Experiment 2, colonic and faecal ALP activity was significantly increased in the RAF and LAC groups (P<0·05, Fig. 1(b) and (d)). The colonic ALP preparations were strongly inhibited by only l-phenylalanine (Table 3). The ALP activity of small intestine (duodenum, jejunum and ileum) was not affected by dietary RAF and LAC (Table 4). Serum ALP activity did not differ among the three groups (data not shown).
Colonic gene expression
In Experiment 1, colonic IAP-I gene expression of FOS and GOS was 3·7- and 3·2-fold, respectively, of that in the control group (P<0·05, Fig. 2(a)). Dietary IMOS did not affect the expression of IAP-I. The FOS diet significantly elevated the colonic expression on Akp3 (P<0·05). The expression of Alpl gene did not differ among the four groups. In the duodenum, jejunum and ileum, the expression of IAP-I, Akp3 and Alpl was not affected by the dietary oligosaccharides (Table 4).
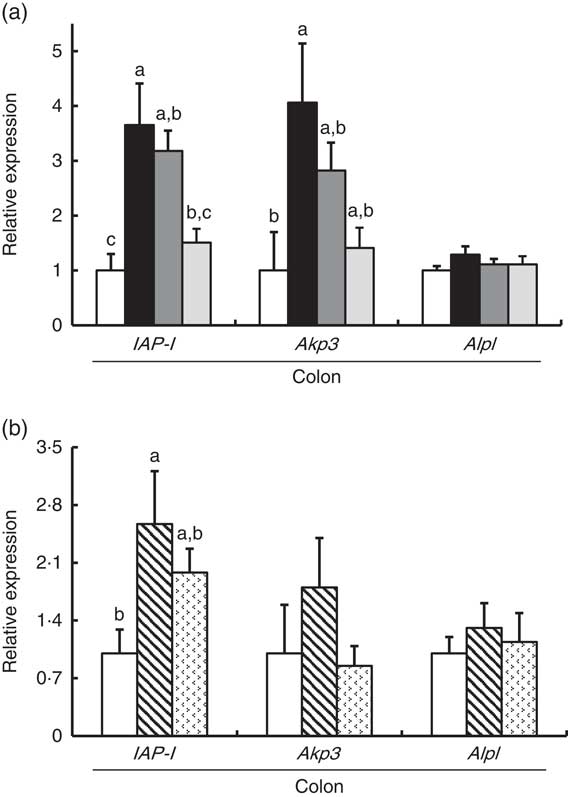
Fig. 2 Colonic gene expression of intestinal alkaline phosphatase (IAP-I), intestinal alkaline phosphatase 3 (Akp3) and alkaline phosphatase, liver/bone/kidney (Alpl) in rats fed a high-fat diet containing oligosaccharides in Experiment 1 (a) and Experiment 2 (b). Values are means (n 6), with their standard errors represented by vertical bars. (a) Control (), fructo-oligosaccharides (
), galacto-oligosaccharides (
) and isomalto-oligosaccharides (
). (b) Control (
), raffinose (
) and lactulose (
). a,b,c Mean values with unlike letters were significantly different (P<0·05; Tukey post hoc test).
In Experiment 2, colonic IAP-I was significantly elevated in the RAF group, and a similar trend was observed in the LAC group (P<0·05, Fig. 2(b)). The colonic expression of Akp3 and Alpl and the small-intestinal (duodenum, jejunum and ileum) expression of IAP-I, Akp3 and Alpl did not differ among the three groups (Fig. 2(b) and Table 4).
Faecal mucins and caecal organic acids
In Experiment 1, faecal mucins in the FOS and GOS diet groups were significantly higher than the control group (P<0·05, Fig. 3(a)). Although the data for faecal mucins were expressed as per gram faecal dry weight, the same results were obtained when expressed as per 3 d. The IMOS diet also increased faecal mucins, although this effect was smaller than that of the FOS and GOS diets (Fig. 3(a)). When the data were expressed per 3 d, IMOS diet had no effect on faecal mucin levels. The expression of Muc2 and Muc3 genes in the duodenum, jejunum, ileum and colon did not differ among the four groups (data not shown). Faecal IgA was significantly elevated in the FOS and GOS groups when the data were expressed as per gram faecal dry weight but not when expressed as per 3 d (data not shown). The pH of caecal digesta was significantly lower in both the FOS and GOS groups (5·32 (sem 0·04) and 5·32 (sem 0·09), respectively) when compared with the control group (6·98 (sem 0·12)) (P<0·05). The FOS and GOS diets significantly increased the caecal levels of lactate and n-butyrate when compared with the control diet (P<0·05) (Fig. 4(a)). The caecal propionate was elevated by the FOS diet. On the other hand, the IMOS group did not affect the pH of caecal digesta (7·30 (sem 0·03)) and the organic acids.

Fig. 3 Faecal mucins in rats fed a high-fat diet containing oligosaccharides in Experiment 1 (a) and Experiment 2 (b). Values are means (n 6), with their standard errors represented by vertical bars. (a) Control (), fructo-oligosaccharides (
), galacto-oligosaccharides (
) and isomalto-oligosaccharides (
). (b) Control (
), raffinose (
) and lactulose (
). a,b,c Mean values with unlike letters were significantly different (P<0·05; Steel–Dwass post hoc test).

Fig. 4 Caecal organic acids in rats fed an high-fat diet containing oligosaccharides in Experiment 1 (a) and Experiment 2 (b). Values are means (n 6), with their standard errors represented by vertical bars. (a) Control (), fructo-oligosaccharides (
), galacto-oligosaccharides (
) and isomalto-oligosaccharides (
). (b) Control (
), raffinose (
) and lactulose (
). a,b Mean values with unlike letters were significantly different (P<0·05; Tukey post hoc test for acetate and n-butyrate, Steel–Dwass post hoc test for succinate, lactate and propionate).
In Experiment 2, faecal mucins were significantly increased in the RAF and LAC groups (P<0·05, Fig. 3(b)), although the expression of Muc2 and Muc3 genes in the duodenum, jejunum, ileum and colon did not differ among the three groups (data not shown). The pH of caecal digesta was significantly lower in both the RAF and LAC groups (5·43 (sem 0·15) and 5·27 (sem 0·11), respectively) when compared with the control group (7·30 (sem 0·13)) (P<0·05). The caecal levels of lactate, n-butyrate, propionate and acetate were significantly increased by dietary RAF and LAC (Fig. 4(b)).
Faecal microbiota
In Experiment 1, faecal abundance of Bifidobacterium spp. was significantly higher in the FOS and GOS groups than in the control group (P<0·05; Table 5). The FOS diet markedly increased the abundance of the Lactobacillus spp. (P<0·05). The FOS and GOS groups showed a significant decrease in the abundance of the Clostridium coccoides when compared with the control group (P<0·05). A similar effect of dietary FOS and GOS was observed on Clostridium leptum. The IMOS diet did not modulate faecal microbiota.
Table 5 Faecal microbiota in rats fed a high-fat diet containing oligosaccharides (Mean values with their standard errors; n 6)
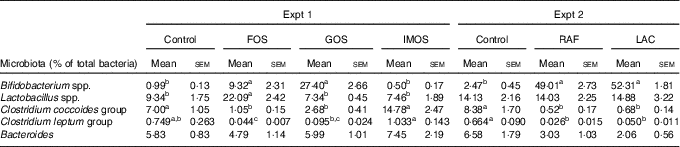
FOS, fructo-oligosaccharides; GOS, galacto-oligosaccharides; IMOS, isomalto-oligosaccharides; RAF, raffinose; LAC, lactulose.
a,b,c In each experiment, mean values within a row with unlike superscript letters were significantly different (P<0·05; Tukey post hoc test for Bacteroides, Steel–Dwass post hoc test for Bifidobacterium spp., Lactobacillus spp., Clostridium coccoides group and Clostridium leptum group).
In Experiment 2, dietary RAF and LAC significantly increased faecal abundance of Bifidobacterium spp. and decreased the abundance of C. coccoides and C. leptum when compared with the control diet (P<0·05, Table 5).
Relationship between colonic alkaline phoshatase and gut epithelial homoeostasis, mucin, organic acids and microbiota
In Experiments 1 and 2, colonic ALP activity significantly correlated with faecal mucins (R s 0·644, P<0·001 and R s 0·759, P<0·001, respectively) caecal lactate (R s 0·613, P<0·005 and R s 0·717, P<0·001, respectively) and n-butyrate (R s 0·584, P<0·005 and R s 0·701, P<0·005, respectively) and faecal ratio of Bifidobacterium spp. (R s 0·566, P<0·005 and R s 0·639, P<0·005, respectively) and inversely correlated with faecal ratio of C. coccoides (R s –0·730, P<0·001 and R s –0·705, P<0·005, respectively) and C. leptum (R s –0·684, P<0·001 and R s –0·649, P<0·005, respectively).
Discussion
It has been previously demonstrated that soluble dietary fibre, glucomannan, elevates colonic ALP without affecting the small-intestinal ALP activityReference Okazaki and Katayama (9) . In the present study, FOS, GOS, RAF and LAC increased colonic ALP activity, whereas IMOS which is partially digestible oligosaccharides did not. The ALP activities in the duodenum, jejunum and serum were not affected by dietary oligosaccharides, although ileum ALP was slightly increased only by GOS. These results suggest that consumption of non-digestible oligosaccharides specifically enhances the colonic ALP activity of rats fed an HF diet and imply that increased colonic fermentation with oligosaccharides intake may associate with the stimulation of colonic ALP.
The inhibitory effects of l-phenylalanine, l-homoarginine and levamisole on the oligosaccharides groups demonstrated that colonic ALP was a typical intestinal ALP. Therefore, the elevation of colonic ALP activity in the FOS, GOS, RAF and LAC groups is due to an increase in intestinal-type ALP. Our study revealed that dietary non-digestible oligosaccharides (FOS, GOS, RAF and LAC) commonly increased the colonic expression of IAP-I without affecting the expression of ALP genes in duodenum, jejunum and ileum, whereas some digestible oligosaccharides (IMOS) did not. The IAP-I gene is expressed throughout the intestine, whereas the Akp3 gene is expressed specifically in the duodenumReference Narisawa, Hoylaerts and Doctor (20) . In the present study, although colonic Akp3 expression was elevated by FOS, the expression was approximately 1/470 less than that of IAP-I. The results suggest that the Akp3 gene does not significantly contribute to colon ALP activity. Therefore, the increase in colonic ALP activity in rats fed non-digestible oligosaccharides may be associated with elevated colonic IAP-I expression.
The present results demonstrate that dietary non-digestible oligosaccharides increased faecal ALP activity and the activity was relatively high (1000-fold) compared with that in colon. Therefore, it is difficult to explain that the elevation in colonic ALP by non-digestible oligosaccharides contributes to faecal ALP activity. On the other hand, faecal ALP was highly correlated with faecal mucin (R s 0·934, P<0·001 in Experiment 1 and R s 0·893, P<0·001 in Experiment 2). Faecal ALP reflects the production, digestion and degradation of ALP in the intestine, and these processes can be modulated by dietsReference Lalles (30) . In the present study, colon and small-intestinal gene expression of mucin was not changed by dietary manipulations. Hino et al. Reference Hino, Takemura and Sonoyama (31) have suggested that increased mucin secretion by indigestible carbohydrate may be accompanied by accelerated epithelial cell turnover. Therefore, increased faecal ALP by non-digestible oligosaccharides may be mainly mediated by increased turnover of intestinal cells.
Small-intestinal ALP is recently reported to improve gut barrier function by regulating tight junction protein levelsReference Liu, Hu and Huo (32) , although the effect of colonic ALP is remains unknown. This study showed that dietary non-digestible oligosaccharides commonly elevated faecal mucins and that the mucin level was highly associated with colonic ALP activity. Mucins play an important role in the protection of gut barrier functionReference Deplancke and Gaskins (11) . Gomes et al. Reference Gomes, Ayub and Dos Reis (33) have reported that the distribution of mucin-secreting goblet cell and IAP activity occurs during the development of the intestine in suckling and weaning mice. It has been demonstrated that mucin-deficient mice had impaired intestinal ALP expression and lipopolysaccharide detoxification activityReference Zarepour, Bhullar and Montero (34) . Taken together, these studies suggest that elevated colonic ALP activity induced by non-digestible oligosaccharides has a strong relationship with increased gut mucin secretion, which may be associated with colonic barrier function.
In accordance with a previous reportReference Tanabe, Ito and Sugiyama (35) , dietary FOS, GOS, RAF and LAC elevated caecal n-butyrate. n-Butyrate is utilised as an energy source in colon epithelia and modulates the proliferation, apoptosis and activity of immune cells in the epithelial layer( Reference Scheppach 36 , Reference Tang, Chen and Jiang 37 ), which results in a lower risk of colon disease. ALP was recently shown to be induced by butyrate in colon cancer cell linesReference Joongho, Azadeh and Georgia (38) . Higher butyrate production in the rat caecum has been reported to stimulate mucin production and immune cells in the gut( Reference Shimotoyodome, Meguro and Hase 39 – Reference Ishizuka, Tanaka and Xu 41 ). Therefore, we assessed the relationship between caecal butyrate and colon ALP activity. The results indicated that caecal butyrate was significantly correlated with colon ALP activity. Thus, the elevated production of butyrate, a fermentation product, in the FOS, GOS, RAF and LAC groups may relate to the increased activity of colon ALP.
Lactate is absorbed more slowly in the gut than other organic acidsReference Hoshi, Sakata and Mikuni (42) , and the pH of caecal digesta is considerably lower, which favours acid-resistant bacteria such as bifidobacteria. Similar to the previous reports( Reference Bornet and Brouns 15 , Reference Watzl, Girrbach and Roller 16 ), the consumption of FOS, GOS, RAF and LAC modulated microbiota by increasing bifidobacteria. Several studies indicated that consumption of fermentable oligosaccharides increased intestinal Bifidobacterium as well as n-butyrate( Reference Bornet and Brouns 15 , Reference Watzl, Girrbach and Roller 16 , Reference Tanabe, Ito and Sugiyama 35 , Reference Komura, Fukuta and Genda 43 ). The novelty of this study is that a correlation was made between faecal Bifidobacterium spp. and colon ALP activity. Further study is necessary to test whether the elevated Bifidobacterium by non-digestible oligosaccharides is related to the induction of colonic ALP.
Ling et al. Reference Ling, Linglong and Weixia (44) reported a protective effect of mucins, n-butyrate and Bifidobacterium on intestinal barrier function. Our results revealed a significant correlation of colon ALP activity with mucins, n-butyrate and Bifidobacterium. Taken together, these studies imply that elevated colonic ALP activity induced by non-digestible oligosaccharides may have a relationship with colonic defense systems. However, the physiological significance of increased colonic ALP activity remains unclear. Additional studies are needed to investigate the relationship between non-digestible oligosaccharides-induced increases in colon ALP and colonic barrier functions.
In conclusion, our study provides evidence for a novel effect of dietary non-digestible oligosaccharides on colonic ALP activity and the gene expression of IAP-I in rats fed an HF diet and demonstrates that elevated colon ALP by non-digestible oligosaccharides has a relationship with increased production of mucin secretion, n-butyrate and Bifidobacterium. These data, including our previous results, support the hypothesis that fermentable non-digestible carbohydrate commonly elevates colonic ALP activity as well as improves intestinal barrier function, fermentation and microbiota. We speculate that these effects on colonic ALP might be important for protection of gut epithelial homoeostasis. Our study was limited by the lack of investigation of the molecular mechanisms associated with our findings. These will be investigated in further studies, and additional research is now in progress to examine whether the elevated colonic ALP by dietary non-digestible oligosaccharides promote the detoxification of colonic lipopolysaccharides.
Acknowledgements
The authors thank Hazuki Kubo and Reika Honma for their technical assistance.
This research was supported by JSPS KAKENHI grant number 15K00836.
Y. O. and T. K. conceived, designed and performed the experiments. Y. O. analysed the data. Y. O. and T. K. wrote the paper. Both authors read and approved the final manuscript.
The authors declare that there are no conflicts of interest.