Introduction
Deep brain stimulation (DBS) is an established surgical therapy for movement disorders such as Parkinson’s disease (PD), essential tremor and dystonia.Reference Fasano and Lozano1 Although, rechargeable implantable pulse generators (IPGs) and those with constant current outputs are becoming available, most IPGs used to date are non-rechargeable and voltage controlled. Depending on their longevity, which correlates to the stimulation parameters and consumed energy,Reference Bin-Mahfoodh, Hamani, Sime and Lozano2 the non-rechargeable IPGs need to be replaced before end of life. The new IPG should ideally be programmed to deliver consistent energy to the target brain tissue to maintain appropriate neurological symptom control.
Although IPG replacement in the subcutaneous pocket is a minor surgical procedure with minimal expected complications, any electrophysiological inconsistency in the target brain tissue may result in neurological worsening or appearance of new side effects. Allert et al.Reference Allert, Kirsch, Weirich and Karbe3 reported lack of symptom stability in 47% and 35% of cases, after single- and double-channel IPG replacement, respectively. In this study, we evaluated the possibility of neurological worsening and associated electrophysiological correlates after IPG replacement in PD cases on chronic DBS therapy (CDT) along with the therapeutic interventions employed to alleviate them.
Materials and Methods
This study was a retrospective chart review of PD cases on CDT followed in the DBS outpatient clinic at the Movement Disorders Centre in London, Ontario, Canada from January 2010 to December 2016. After optimal initial programming, PD cases on CDT were assessed in the clinic every six months. As a routine, patients are advised to regularly check the IPG battery status and immediately contact the clinic staff on getting an elective replacement indicator (ERI) signal. IPG replacement is organized and done by the neurosurgeon who performed the initial DBS surgery. Three neurosurgeons perform IPG replacement at our center with approximately one replacement per month. The procedures are done under short general anesthesia using a bipolar cautery. Pre-replacement IPG parameters are readily available for post-operative programming of the new IPG. Neurological worsening after the IPG replacement in any patient is immediately communicated to the neurology team. After IPG replacement, the patients either contacted the clinic staff before a follow-up visit or discussed neurological worsening or appearance of a new side effect on a follow-up clinic visit.
The study was approved by the Western University Human Subjects Research Ethics Board (HSREB# 108576). Clinic charts of 91 PD cases on CDT were reviewed. For each case with IPG replacement, the following parameters were extracted: demographic profile, including age and sex, diagnosis, duration of illness, indication and date of DBS surgery, target brain nuclei for stimulation, laterality (unilateral or bilateral), indication and date of IPG replacement, type of implanted IPG, DBS stimulation parameters (active contacts, voltage, pulse width, frequency, impedance, and current) before and after IPG replacement and clinical complications appearing after IPG replacement. In patients with clinical complications after IPG replacement, information regarding the nature of clinical deterioration and adjustments made in the stimulation parameters or medications to alleviate them were reviewed.
The stimulation parameters, namely voltage, impedance, and total electrical energy delivered (TEED), in pre- and post-replacement state of the IPGs were compared and any deviation in the post-replacement state as compared to pre-replacement state was converted to a percentage of the pre-replacement value to attain the percentage change. The pulse width and frequency were not changed during any of the IPG replacements. The TEED values were calculated using the following formula:Reference Koss, Alterman, Tagliati and Shils4, Reference Moro, Esselink, Xie, Hommel, Benabid and Pollak5

IBM SPSS® statistics version 21 was used for analyzing the data. Shapiro–Wilk test was used to determine normal distribution of data. Data with normal distribution were analyzed using an independent-sample t test. Data not meeting the criteria of normal distribution were analyzed using nonparametric Mann–Whitney test. Statistical analysis was two sided, and a p value of <0.05 was considered statistically significant.
Results
A total of 62 IPGs involving 121 channels were replaced in 45 PD cases during the seven-year period. Bilateral subthalamic nucleus DBS (STN-DBS) was performed in 44 cases with right globus pallidus internus DBS (GPi-DBS) in a single case with prior left pallidotomy. Although 57 replaced IPGs were non-rechargeable constant-voltage devices, the remaining five were rechargeable devices including constant-current devices in two cases. Fifty-nine (95.2%) IPGs were dual-channel and three (4.8%) were single-channel devices (Supplementary table 1). Thirty-two cases had IPG replacement once, 11 had twice, 1 had thrice, and a single patient required five IPG replacements, with IPG site infection and hardware malfunction complicating two of the IPG replacements in this individual.
Fifty-two (83.8%) IPG replacements were elective and six (9.6%) followed end of service (EOS), that is, complete battery failure. Two (3.2%) IPGs were replaced following hardware malfunction including one with rapid drainage of battery from short circuit. Although a single case developed skin-erosion (without frank infection) requiring IPG replacement, another case developed IPG site infection necessitating replacement. The mean time to replace the 62 IPGs was 49.17 ± 18.17 months. Although the mean time to replace the 59 dual-channel IPGs was 49.52 ± 18.02 months, it was 42.3 ± 24.0 months for the three single-channel IPGs (Supplementary table 1).
Overall, 35.5% (16/45) cases developed neurological worsening involving 27.4% (17/62) IPG replacements. All complications involved dual-channel IPGs and appeared in the first week after replacement. Neurological worsening was observed in 26.9% (14/52) of IPGs replaced electively and 16.6% (1/6) IPGs replaced following EOS (Supplementary table 1). Although 70.6% (12/17) of the replaced IPGs associated with neurological worsening were the first IPG replacements for those PD cases, 29.4% (5/17) were repeated replacements with 17.6% (3/17) being second and 5.8% (1/17) each being third and fourth IPG replacements. The neurological worsening reported by patients including reduced DBS efficacy or appearance of new symptoms were corroborated on neurological examination using MDS-UPDRS scale part III and/or part IV (Supplementary table 2). Tremor worsened in 41.2% (7/17), speech in 35.3% (6/17), and gait in 23.5% (4/17) of the cases reporting neurological deterioration. Generalized troublesome dyskinesia was seen following 11.8% (2/17) IPG replacements. Worsening of bradykinesia (17.6%), rigidity (11.8%), and appearance of limb dystonia (5.9%) were the other neurological symptoms noticed.
Of the 121 channels in the 62 replaced IPGs, 96 had monopolar and 25 had bipolar stimulation. Neurological worsening was observed in 29.1% (28/96) monopolar and 24% (6/25) bipolar channels with a non-significant difference (p = 0.60). Considering the possibility of reduction in the impedance due to cleaning of lead contacts prior to reinsertion into the new IPG along with the new IPG potentially delivering a more steady current, the new IPGs were often programmed to a lower voltage. In three cases, the voltage was increased due to patient request of poor symptom control in the immediate post-operative period. Among the 117 channels of the new constant-voltage IPGs, the voltage matched the pre-replacement values in 40 channels, a reduction was observed in 59 and an increase in 18 channels. Deviation from pre-replacement voltage values was observed in 88.25% (15/17) IPGs developing neurological worsening. Four (23.5%) IPGs had additional obvious causes including switched laterality in a single case and hardware related complication in three cases. Of the three cases with hardware involvement, two had IPG site infection and the third developed eroded insulation in the DBS leads. Of the two dual-channel IPGs replaced with constant current devices, one case developed generalized dyskinesia resulting from the current being programmed to a value higher than the pre-replacement state. Thus, voltage inconsistency appeared primarily responsible for 58.8% (10/17) neurological worsening, thereby, accounting for neurological worsening in 16.1% (10/62) of all IPG replacements.
Of the available post-replacement impedance values in 115 channels, a reduction was observed in 49 channels, an increase in 62 channels and 4 channels had values similar to the pre IPG replacement. The median value of percentage change in post IPG replacement voltage from the pre-replacement one was statistically significant on comparing cases with and without neurological worsening. The deviation in voltage and impedance significantly affected TEED in cases with neurological worsening (p = 0.002) (Figure 1).

Figure 1: Box plots showing the median along with the first and third quartiles of the percentage change in voltage (a), impedance (b), and TEED values (c) after IPG replacement in channels with and without neurological worsening, both pre- and post-stabilization. Abbreviations: IPG: implantable pulse generator; n = number of IPG channels; NW: neurological worsening; TEED: total electrical energy delivered. Pulse width and frequency remained unchanged throughout for all patients. No changes from monopolar to bipolar or vice versa were needed.
Adjustment in voltage contributed to neurological stabilization in 76.4% (13/17) of the affected IPGs. Although 44.1% channels in these IPGs required an increase, 26.5% channels required a reduction in voltage to achieve neurological stabilization. In addition to adjustment in voltage, change in active contacts was required in 11.7% (2/17) of cases. Although switching of laterality affected two dual-channel IPG replacements, only one developed neurological worsening, which improved on switching the stimulation parameters to match the laterality. Since the second IPG had symmetric stimulation parameters, neurological worsening was not observed. Of the two cases developing dyskinesia after IPG replacement, one improved with bilateral reduction in voltage. The second patient was on levodopa and a 66% levodopa dose reduction provided levodopa sparing and stabilized the neurological status.
Of the two cases with IPG site infection, one was successfully treated with intravenous antibiotics, while a repeat IPG replacement was required in the second case. After a subsequent IPG replacement, the second case developed worsening of tremor and rapid drainage of IPG due to short circuit resulting from eroded insulation of the DBS leads. It required repeat IPG replacement along with repair of the insulation.
The deviations in stimulation parameters in cases with neurological worsening along with the therapeutic interventions employed for neurological stabilization is summarized in Supplementary table 2. The cases with neurological worsening after IPG replacement required an average of 2.2 (range = 1–4) follow-up visits in the DBS clinic with an average duration of 4.4 months (range = 1–6 months) to stabilize neurologically. These cases remained neurologically stable over a subsequent six months follow-up. After applying various interventions to achieve neurological stabilization in cases with worsening, the statistical significance disappeared while comparing median values of percentage change in voltage and TEED (Figure 1).
Discussion
This study suggests a proactive manipulation in the voltage after IPG replacement done with an aim to maintain a consistent TEED at the target brain tissue may significantly reduce the possibility of neurological worsening after IPG replacement. Surgeons often preferred reducing the voltage while programming the new IPG and patients continued to experience a stable improvement in their symptoms. Reduction in contact impedance and possibly a more stable current delivery with the new battery could be the potential reasons, not directly explored in this study. However, this strategy did not work in everyone with a significant number of patients requiring re-manipulation of their voltage to achieve a consistent TEED for neurological stabilization. The best predictor of this manipulation however was not the absolute voltage but the TEED value in the pre-replacement state.
The possibility of neurological worsening observed following 27.4% of the replaced IPGs in this study is lower than that reported by Allert et al.Reference Allert, Kirsch, Weirich and Karbe3 who described neurological worsening in 43% of their cases. They reported a slightly higher possibility of neurological worsening in EOS cases as compared to elective replacements but the latter had a higher complication rate in our series, probably related to a low number of EOS cases. Since sudden discontinuation of DBS therapy may result in acute neurological worsening,Reference Blahak, Capelle, Baezner, Kinfe, Hennerici and Krauss6– Reference Chou, Siderowf, Jaggi, Liang and Baltuch8 elective IPG replacement is preferred. Regular follow-up visits in the DBS clinic along with the awareness to diligently check the battery status with the patient-programmer may have reduced EOS cases in our study.
Technical factors appeared the most common cause of neurological worsening seen. Although the deviation from the pre-replacement voltage was primarily responsible for worsening after IPG replacement in ten cases, a single case with new constant current dual-channel IPG had deviation in current delivered and worsening in another case resulted from switched laterality. Although technical factors resulted in neurological worsening in 19.4% of all IPG replacements in our study, similar factors accounted for neurological worsening in 19.6% of cases reported by Allert et al.Reference Allert, Kirsch, Weirich and Karbe3 These technical complications can be prevented by careful evaluation of the stimulation parameters along with the laterality while programming the new IPG to maintain a consistent TEED at the target brain tissue. Temporary delineation of the right extension lead before detaching from the drained IPG has been reported to avoid erroneous switch in laterality while replacing dual-channel IPGs.Reference Latini, Sensi, Preda and Cavallo9
IPG site infection affected two IPG replacements associated with neurological worsening, with one case requiring a repeat IPG replacement. Although Allert et al.Reference Allert, Kirsch, Weirich and Karbe3 reported short circuit of the active electrodes in two of their cases (2/56 = 3.5%) with both improving on IPG reprogramming, a single case in our series experienced a short circuit requiring surgical revision with repair of insulation in the DBS leads.
Impedance fluctuations appeared to be the primary source of electrophysiological inconsistency causing worsening following cases where no other technical problem was detected. Although this accounted for 3.2% of all IPG replacements in our study, Allert et al.Reference Allert, Kirsch, Weirich and Karbe3 reported neurological destabilization in 20% of their cases possibly due to deviation in the impedance from the pre-replacement values. Impedance depends on the connectivity between the IPG and electrodes and their surface area along with the conductivity of the tissue layers surrounding the IPG and electrodes.Reference Butson, Maks and McIntyre10, Reference Montuno, Kohner, Foote and Okun11 In constant-voltage IPGs, the impedance values of electrodes directly influence the current delivered to the target brain tissue, thereby affecting the volume of tissue activated and the neurological response achieved.Reference Bronstein, Tagliati and McIntyre12
Although reports have highlighted the importance of voltage in predicting the DBS response,Reference Moro, Esselink, Xie, Hommel, Benabid and Pollak5, Reference Huang, Watts and Montgomery13 some authors consider it inappropriate to give credence to a single parameter in the context of the complex and non-linear neuronal dynamics and favor using TEED.Reference Fasano and Lozano14, Reference Fasano and Lozano15 In similitude, the cases presented in this study, where the frequency and pulse width remained constant in pre- and post-replacement states and impedance fluctuated, adjusting the voltage to achieve a consistent TEED resulted in neurological stabilization in cases manifesting neurological worsening after IPG replacement.
Considering the possibility of neurological worsening after IPG replacement in a substantial number of PD cases on CDT, it is essential to contemplate these complications along with other immediate or long-term complications of DBS surgery. In view of our results, we propose an algorithm to reprogram the new IPG with an aim to match the pre-replacement TEED (Figure 2). By reducing the number of variables required to maintain consistent energy delivery to the target brain tissue, constant-current IPGs may minimize the influence of impedance fluctuation after IPG replacements, thereby reducing the possibility of neurological worsening.Reference Bronstein, Tagliati and McIntyre12 Use of rechargeable IPGs may reduce the requirement of repeated IPG replacements and is possibly more cost effective.Reference Rizzi, Messina, Penner, D’Ammando, Muratorio and Franzini16, Reference Pepper, Zrinzo, Mirza, Foltynie, Limousin and Hariz17
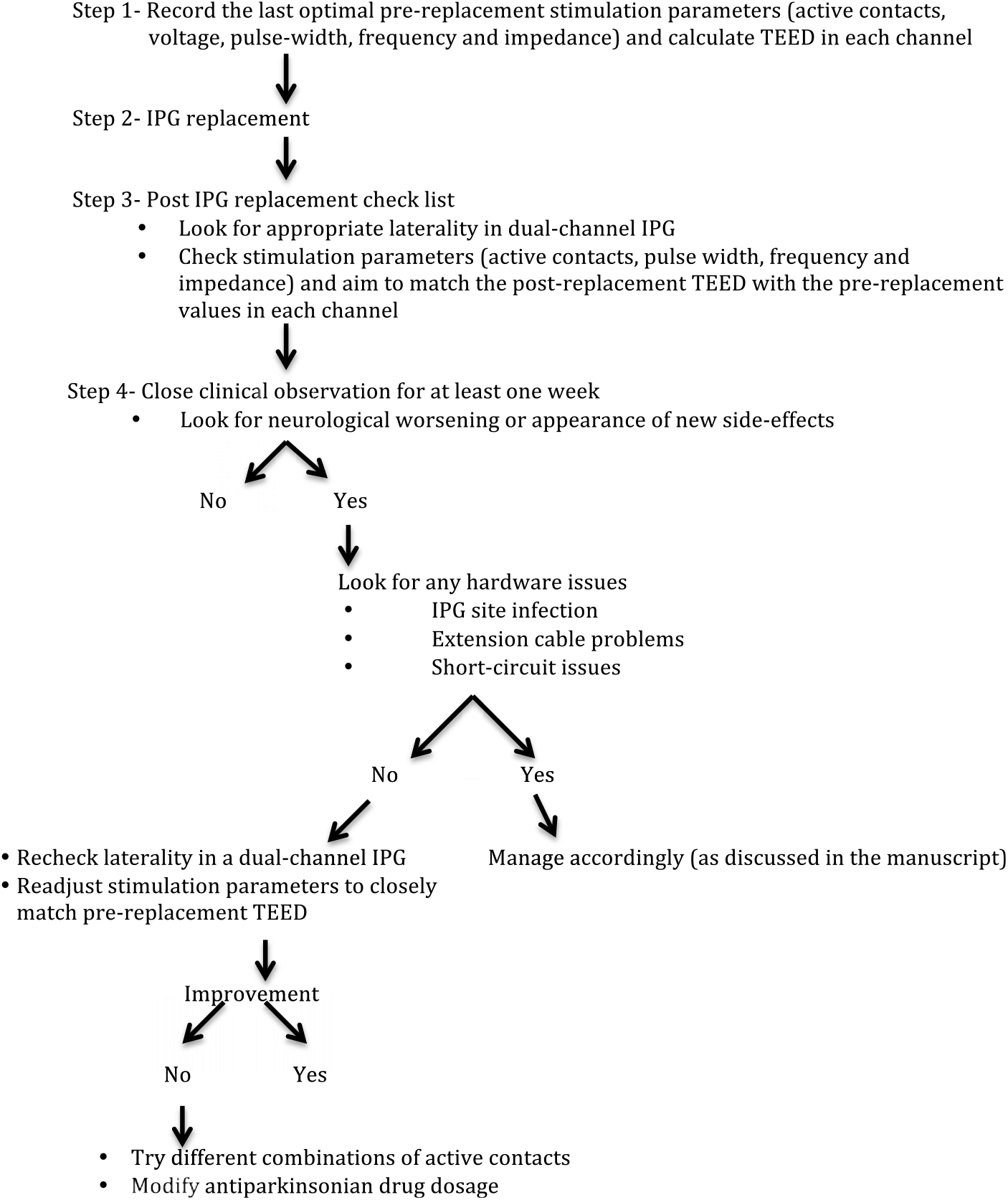
Figure 2: Algorithm to minimize and manage post IPG replacement neurological worsening in Parkinson’s disease cases on chronic deep brain stimulation therapy. Abbreviations: IPG: Implantable pulse generator; TEED: Total electrical energy delivered.
The retrospective nature and reliance on recorded information are a limitation of our study. Since our study includes data based on a single-center practice, studies from other centers may enhance the understanding in this regard.
Conclusion
Our study reports neurological worsening following 27.4% IPG replacements in PD cases on CDT. Approximately 82.4% of these complications can be averted by reprogramming the new IPG with an aim to match the pre-replacement TEED values and ensuring appropriate laterality in dual-channel IPGs. Use of constant-current IPGs or rechargeable IPGs may reduce the likelihood of neurological worsening after IPG replacement.
Disclosures
Drs. Kumar and Murgai report no disclosures. Dr. Jog reports no disclosure related to this study. Outside of this study, he receives speaker and consultant honoraria from Merz Pharmaceuticals, Allergan, AbbVie. He also receives research grants from CIHR, AMOSO, Allergan, Merz Pharmaceuticals and Lawson Health Research Institute and is part of the AGE-WELL Network of Centers of Excellence (NCE) of Canada program. From time to time, he serves on advisory boards of Allergan, Boston Scientific, AbbVie, and Merz Pharmaceuticals.
Statement of Authorship
Dr. NK: conception, design and writing the first manuscript. Dr. AM: review and critique. Dr. MJ: conception, review and critique.
Supplementary Material
To view supplementary material for this article, please visit https://doi.org/10.1017/cjn.2019.51.