Perennial weed control is costly, time-consuming, and can negatively impact the environment. Quackgrass is a rhizomatous perennial grass that causes significant yield losses in agriculture in the temperate zones of the world. Like many other perennial weeds, quackgrass is generally controlled through glyphosate-based herbicides or intensive tillage between main crops (Melander et al. Reference Melander, Nørremark and Kristensen2013; Rasmussen et al. Reference Rasmussen, Melander, Askegaard, Kristensen and Olesen2014). In addition to the potential risk to human health and the environment, relying heavily on herbicides encourages the development and spread of herbicide-resistant weeds (Heap Reference Heap2014). To control weeds by intensive tillage in the postharvest period, on the other hand, increases the risk of nitrogen leaching (Aronsson et al. Reference Aronsson, Ringselle, Andersson and Bergkvist2015) and soil erosion (Meyer et al. Reference Meyer, Dabney, Murphree, Harmon and Grissinger1999). Intensive tillage also generally requires more on-farm energy usage than chemical weed control (Tzilivakis et al. Reference Tzilivakis, Jaggard, Lewis, May and Warner2005). Thus, there is a need for a selection of diverse methods that can efficiently control quackgrass and other perennial weeds with little environmental impact.
One of the primary reasons that quackgrass and other perennial weeds are so persistent is their ability to store reserves in their underground storage organs (e.g., fructan in quackgrass rhizomes). Underground storage organs enable vegetative propagation and aboveground shoot regrowth after disturbance and let perennial weeds pass on their resources from year to year. Control methods must either destroy/remove the underground storage organs or disrupt and suppress them by preventing or reducing the translocation of photoassimilates from the aboveground biomass (Håkansson Reference Håkansson1968a, Reference Håkansson1968b, Reference Håkansson1969). However, for rhizomatous plants such as quackgrass, it may be possible to use minimal tillage techniques to fragment the rhizomes, and consequently weaken the underground storage network. Weakened rhizomes are potentially more susceptible to low-intensity control methods, such as effective crop competition and mowing.
Crop competition effectively reduces both above- and belowground biomass acquisition of quackgrass (Bergkvist et al. Reference Bergkvist, Adler, Hansson and Weih2010; Cussans 1968, 1970). In addition, the crop provides other benefits, such as harvestable yield, reduced risk of erosion, increased soil water retention, and increased soil microbial activity (Lemessa and Wakjira Reference Lemessa and Wakjira2015). However, the competitive ability of crops on quackgrass depends on a large number of factors, such as the crop biomass, primary mode of competition (Ringselle et al. Reference Ringselle, Prieto-Ruiz, Andersson, Aronsson and Bergkvist2017), and allelopathy (Zou et al. Reference Zou, Santanen, Tein, Stoddard and Mäkela2014). Furthermore, as the suppressive effect of the crop is unlikely to be consistent across years, additional methods are necessary for enhancing the competitive effect of crops on quackgrass.
To enhance the competitive ability of crops against quackgrass, mowing has been used in grass–legume leys (Bergkvist et al. Reference Bergkvist, Ringselle, Magnuski, Mangerud and Brandsæter2017; Cussans Reference Cussans1973; Lötjönen and Salonen Reference Lötjönen and Salonen2016) and undersown cover crops (Brandsæter et al. Reference Brandsæter, Goul Thomsen, Wærnhus and Fykse2012; Ringselle et al. Reference Ringselle, Bergkvist, Aronsson and Andersson2015). Mowing cuts the aboveground biomass of plants, which reduces both belowground and aboveground biomass accumulation in plants in general (Ferraro and Oesterheld Reference Ferraro and Oesterheld2002; Harrison and Hodgson Reference Harrison and Hodgson1939; Holmøy and Teslo Reference Holmøy and Teslo2000; Youngner Reference Youngner1972) and in quackgrass specifically (Dexter Reference Dexter1936; Håkansson Reference Håkansson1969; Ringselle et al. Reference Ringselle, Bergkvist, Aronsson and Andersson2015; Turner Reference Turner1966, Reference Turner1968). In addition to reducing biomass, repeated mowing can also decrease quackgrass fructan reserves (Henskens Reference Henskens1993; Pollock Reference Pollock1984; Turner Reference Turner1968). Among grasses, quackgrass is particularly good at withstanding repeated defoliation (Harrison and Hodgson Reference Harrison and Hodgson1939). Therefore, to reduce the fructan storage reserves in quackgrass, the number of shoot cuttings needs to be higher than for many other grasses (Gräßler and Von Borstel Reference Gräßler and Von Borstel2005). Håkansson (Reference Håkansson1969) and Turner (Reference Turner1966) found that cutting every 14 d was necessary to prevent quackgrass from producing rhizomes, but cutting height influenced the effect. A low cutting height, and thus less foliage, is more effective than a cutting height that leaves more foliage for regrowth (Håkansson Reference Håkansson1969; Youngner Reference Youngner1972). In the field, the effect of mowing has often been weaker than traditional tillage and has been inconsistent between years (Brandsæter et al. Reference Brandsæter, Goul Thomsen, Wærnhus and Fykse2012; Lötjönen and Salonen Reference Lötjönen and Salonen2016; Ringselle et al. Reference Ringselle, Bergkvist, Aronsson and Andersson2015) and seasons (Bergkvist et al. Reference Bergkvist, Ringselle, Magnuski, Mangerud and Brandsæter2017). Additionally, the efficacy of mowing at very high frequencies and low cutting heights has to be balanced against the damage caused to the crop. Thus, it would be desirable to combine mowing with treatments that allow mowing to be effective at a low cutting frequency and high cutting height.
One of the reasons why tillage is effective against quackgrass is that it affects the weed in multiple ways: the aboveground biomass is generally destroyed, which interrupts photosynthesis until new shoots can be established, and the rhizomes are often displaced and/or fragmented. Displacement damages the root network and either brings the rhizomes to the soil surface so they dry out or can be collected (Melander et al. Reference Melander, Nørremark and Kristensen2013) or buries the rhizomes deeper in the soil, where the plant requires more resources to reach the surface (Håkansson Reference Håkansson1968a, Reference Håkanssonb). Rhizome fragmentation has been shown to cause an increase in shoot production due to broken apical dominance (Vengris Reference Vengris1962). Additionally, Turner (Reference Turner1968) found that the carbohydrate storage reserves from smaller rhizome pieces were depleted faster during reshooting and recovered more slowly than those from larger rhizomes. Thus, shoots from smaller rhizome fragments may be weaker than those from longer fragments, as they have fewer resources. For instance, the smaller the rhizomes and the deeper they are buried, the less likely their shoots are to reach the soil surface (Håkansson Reference Håkansson1968a, Reference Håkansson1968b; Permin Reference Permin1973; Vengris Reference Vengris1962). Thus, to avoid increased propagation and to take advantage of the weakened shoots, tillage is often repeated to starve the rhizomes (Håkansson Reference Håkansson1968a, Reference Håkansson1968b), a strategy that works unless the growth period is too short (Ringselle et al. Reference Ringselle, Bergkvist, Aronsson and Andersson2016).
One way to increase the efficacy and consistency of low-intensity control methods such as mowing and crop competition may be to combine them with rhizome fragmentation. The concept was tested by Bergkvist et al. (Reference Bergkvist, Ringselle, Magnuski, Mangerud and Brandsæter2017), who combined mowing with rhizome fragmentation, using a spade in a cross-cutting pattern (a recently developed prototype, “Kverneland Vertical rhizome/root cutter (tractor propelled)” can make similar slits at the field scale) in a white clover sward. The rhizome acquisition of quackgrass was reduced by up to 60% by fragmentation, and the effect tended to be higher when combined with mowing. However, questions remain regarding the consistency of the rhizome fragmentation effect, what shoot-cutting frequency and shoot-cutting height work most efficiently together with rhizome fragmentation, and whether there are synergistic effects when both crop competition and shoot cutting are combined with rhizome fragmentation.
The aim of the study was to investigate combinations of management practices that are likely to be efficient in reducing quackgrass in a clover-dominated sward. The hypotheses were: (1) rhizome fragmentation will increase the number of quackgrass shoots; and (2) combination of clover competition, shoot cutting, and rhizome fragmentation will reduce quackgrass biomass and fructan acquisition. To test these hypotheses, pot experiments with the following factors were conducted in 2012 and 2013: (1) rhizome fragmentation, (2) clover competition, (3) shoot-cutting frequency, and (4) cutting height. Hypothesis 1 was tested 20 d after planting in summer and hypothesis 2 by the end of the experiment, in fall.
Materials and Methods
Study Design, Plant Material, and Treatments
An outdoor pot experiment arranged in a factorial randomized design with three complete blocks was conducted in 2012 and 2013 in Ås, Norway (59.666°N, 10.766°E). Different levels of four factors and all their combinations were tested: (1) degree of rhizome fragmentation (4 levels: one 40-cm rhizome, two 20-cm rhizomes, four 10-cm rhizomes, and eight 5-cm rhizomes), (2) competition from white clover (‘Milkanova’; two levels: present or not), (3) shoot-cutting frequency (4 levels: cutting each time quackgrass reached 2, 4, 6, and 8 true leaves), (4) cutting height (3 levels: 25, 50, and 75 mm from the base). An untreated control (one 40-cm rhizome without shoot cutting or competition) was also planted for reference. Rhizomes were collected the day before trial establishment, in a nearby cereal field with a large quackgrass population. Rhizomes of similar age and health were selected. Node numbers were not counted, but quackgrass generally has, on average, 1 node per 3-cm rhizome (Håkansson Reference Håkansson1967). Rhizomes were fragmented by scissors, weighed, and buried at 5-cm depth in 10-L plastic pots filled with limed peat containing 900 mg N L−1, 35 mg P L−1, and 200 mg K L−1 (Gartnerjord Tjerbo, Felleskjøpet, Norway). Clover seeds were sown at a rate approximating 100 kg ha−1 in 2012, but the rate was reduced to 10 kg ha−1 in 2013. The experiment was established in mid-July in 2012 and early June in 2013 (exact management, sampling, and cutting times are given in Table 1). All pots in each block were planted and sown within 24 h. Pots were watered throughout the experiment to avoid drought effects and fertilized 1 to 2 times corresponding to 50 kg N ha−1 (Yara Superba® Brun NPK-fertilizer 14-4-21 with micro nutrients, Yara, Oslo, Norway) when nutrient deficiency symptoms emerged (Table 1). Shoot cutting was performed each time the treatment group reached the relevant growth stage, based on a visual estimation of the average number of leaves per plant. Earlier planting in 2013 (Table 1) resulted in more shoot cuttings (1 to 6 times) than in 2012 (1 to 4 times).
Table 1 Details on sampling, management, and shoot-cutting dates in 2012 and 2013.

a All rhizomes and seeds were planted and harvested within 24 h of each other in each block, but the three blocks were planted and harvested on different days.
Sampling
Number of main shoots and tillers (defined as per Hess et al. Reference Hess, Barralis, Bleiholder, Buhr, Eggers, Hack and Stauss1997) were recorded at the first cutting at the 4-leaf stage (Table 1). At trial termination (Table 1), the total number of aerial shoots was recorded (main shoots plus tillers), and shoots, rhizomes, and roots were harvested. The new rhizomes were separated from the old ones that remained from the planting, but not all old rhizomes could be retrieved. All plant material was dried for at least 2 d at 60 C and weighed, and dry weight was recorded.
Carbohydrate Analyses
Carbohydrate analyses were only performed in 2013. Dried mixed rhizomes (old and new) harvested in 2013 were ground to a powder (Cyclotec™ 1093 Sample Mill, Foss Tecator AB, Höganäs, Sweden). Samples of 10 to 15 mg were extracted with 1.5 ml 90% ethanol (v/v) for 30 min at 60 C in an ultrasonic bath (USC 200 TH, VWR, Leuven, Belgium) with three 5-min sonications with shaking in between. The tubes were centrifuged (3 min, 15,000 rpm) and the precipitate was washed twice with 1.5 ml 90% ethanol, with centrifugation after each wash to remove sugars. The precipitate after ethanol extraction was extracted and washed in the same way with distilled water. The three water supernatants were combined for fructan determination.
The degree of polymerization of fructans present was tested with thin-layer chromatography (TLC) (Smouter and Simpson Reference Smouter and Simpson1989) on ethanol and water extracts from aboveground and belowground plant material before quantitative analyses. These separate TLC analyses showed that fructans could be efficiently separated by the extraction method, because short-chain fructans partly extracted by ethanol and partly by water were not present.
The fructan concentrations in below- and aboveground plant parts from factorial combinations of the lowest and highest treatment levels (shoot cutting at 8 and 2 leaves, at 25- and 75-mm height, with or without competition) from 2013 were analyzed using the anthrone method (Yemm and Willis Reference Yemm and Willis1954) with fructose as the standard. Belowground plant material from old and new rhizomes was pooled, because initial analyses showed no difference in fructan concentration between old and new rhizomes from the intermediate treatment levels.
Data Analyses and Statistics
Data were analyzed using linear fixed-effects models, that is, all main effects (fragmentation, competition, cutting frequency, cutting height, year, and block) and interactions were modeled as fixed. It was possible to treat blocks as fixed because the blocks were complete (Piepho et al. Reference Piepho, Büchse and Emrich2003). Year was treated as fixed, because it is very difficult to estimate the variance when there are only two levels (e.g., years) (Piepho et al. Reference Piepho, Büchse and Emrich2003). However, as year and block were not of primary interest, they were modeled as blocking factors (i.e., without interactions). Initial rhizome fresh weight was included in the models as a covariate to adjust for any differences in potential shoot vigor and growth at the initiation of the experiments. The model was fitted twice to explore both the continuous and categorical aspects of some of the factors. The first time, three of the explanatory variables (fragmentation, cutting frequency, and cutting height) were treated as continuous explanatory variables, while competition was treated as a categorical explanatory variable. The second time all four explanatory variables were treated as categorical. The fructan concentration and content were only analyzed using the latter model, as there were only two levels per explanatory variable for these response variables. Moreover, year was excluded from these analyses, because fructan was only sampled in 2013. Whenever necessary, data were square-root transformed before analysis to achieve homogeneous variances. Student’s t-tests or Tukey’s HSD tests at α=0.05 were used for comparing means. All analyses and transformations were performed using JMP v. 10.0.0 (SAS Institute, Cary, NC).
Results and Discussion
Quackgrass Growth
By the end of the experiment in 2012 and 2013, quackgrass in the untreated control had acquired 19 (±6, 95% CI) and 79 (±12) g biomass pot−1, respectively (unpublished data). The number of living quackgrass shoots were 44 and 53 pot−1 and the below- to aboveground biomass ratio was 1.17 and 1.08 in 2012 and 2013, respectively (unpublished data).
Effect of Rhizome Fragmentation and Clover Competition on Quackgrass Early Shoot Production
At the early sampling date (about 20 d after planting), clover competition had reduced the total number of quackgrass shoots by 13%, but it did not significantly differ between fragmentation degrees (Table 2). The fragmentation degree did affect the number of tillers and main shoots (Table 2). However, while a higher fragmentation degree resulted in the rhizomes producing more main shoots (P=0.005; regression slope ∼0.02), they also produced fewer tillers per main shoot (P<0.001; ∼−0.02). The interaction between rhizome fragmentation and competition was significant for tillers but not for main shoots (Table 3). When exposed to clover competition, rhizomes produced the same number of tillers, regardless of rhizome fragmentation degree, but a higher fragmentation degree still led to a higher main-shoot production than at a lower fragmentation degree (unpublished data).
Table 2 Effect of clover competition and rhizome fragmentation degree on quackgrass main shoots, tillers, and total shoot numbers at the early sampling date (about 20 d after planting).a

a Numbers are means ± 95% confidence interval over both 2012 and 2013. Letters denote significant differences within the categorical variables competition (Student’s t-tests) and rhizome fragmentation degree (Tukey’s HSD tests).
Table 3 ANOVA table of the statistical model used to calculate statistical significance for quackgrass shoot number (tillers, main shoots, early total shoots, and final total shoots), biomass (shoot and new rhizome biomass), fructan concentrations (%, in aboveground [AG] and belowground [BG] biomass) and total fructan content (g).a

a Fixed factors included rhizome fragmentation degree (Fragment), shoot-cutting frequency (Cut freq.), shoot-cutting height (Cut height), and clover competition (Competition). Initial rhizome fresh weight was used as a covariate. Missing values indicate that the term was not included in the analysis. Some values were square-root (sq. root) transformed.
b P-values <0.05 in bold.
c The df=2 for fructan AG and BG % and total fructan content AG+BG.
There was no support for Hypothesis 1 that rhizome fragmentation results in a higher number of shoots. Previous studies have found an increase in main shoots and attributed it to the breakage of the rhizome apical dominance (Håkansson Reference Håkansson1968b; Permin Reference Permin1973; Vengris Reference Vengris1962). However, the shift in main shoot–tiller ratio indicates (Table 2) that the lower fragmentation degree resulted in more resources for tillers than the higher fragmentation degree. The difference in resource distribution due to the degree of rhizome fragmentation may affect quackgrass’s response to competition. For example, in this experiment, larger rhizomes could adjust the number of tillers they produced to the competitive pressure from the crop (no crop competition resulting in more tillers). However, smaller rhizomes could not likewise adjust the number of main shoots. Similarly, Ringselle et al. (Reference Ringselle, Prieto-Ruiz, Andersson, Aronsson and Bergkvist2017) found that there was a linear negative relationship between the crop biomass and number of tillers produced by quackgrass, but there was no such relationship with the number of main shoots.
Degree of Polymerization of Fructan in Quackgrass
Qualitative analysis with TLC showed that quackgrass had no short-chain fructans. This is in agreement with Arni and Percival (Reference Arni and Percival1951), who suggested fructans with degree of polymerization of 30, and Hammer and Morgenlie (Reference Hammer and Morgenlie1990), who found heavily branched fructans in quackgrass.
Combined Effect of Clover Competition, Shoot Cutting, and Rhizome Fragmentation on the Biomass and Fructan Acquisition of Quackgrass
By the fall, quackgrass biomass and fructan acquisition had been directly altered by clover competition and shoot-cutting frequency and height, but not by rhizome fragmentation (Table 3). Clover competition reduced quackgrass shoot numbers by 72%, shoot biomass by 51%, rhizome biomass by 81% (Figure 1), and belowground fructan concentration by 10 percentage points (Figure 2) compared with no competition (Table 4). Similarly, the more frequent the shoot cutting, the less rhizome biomass quackgrass acquired, and the most frequent shoot cutting reduced the belowground fructan concentration of quackgrass by 12 percentage points compared with the lowest frequency (Figure 2; Table 4). The combination of clover competition and shoot cutting reduced quackgrass biomass acquisition more than individual factors (Figures 1 and 2). For quackgrass shoot numbers, there was a significant interaction between competition and shoot-cutting frequency (Table 3); in pots without clover, a higher cutting frequency resulted in more quackgrass shoots (Figure 1). There were also significant interactions between shoot-cutting height and shoot-cutting frequency on quackgrass rhizome biomass and fructan concentration (Table 3). A lower shoot-cutting height had more impact on quackgrass rhizome biomass and belowground biomass fructan concentration when the shoot cutting was performed more frequently (at 2 or 4 leaves) than less frequently (at 6 and 8 leaves) (Figures 1 and 2).
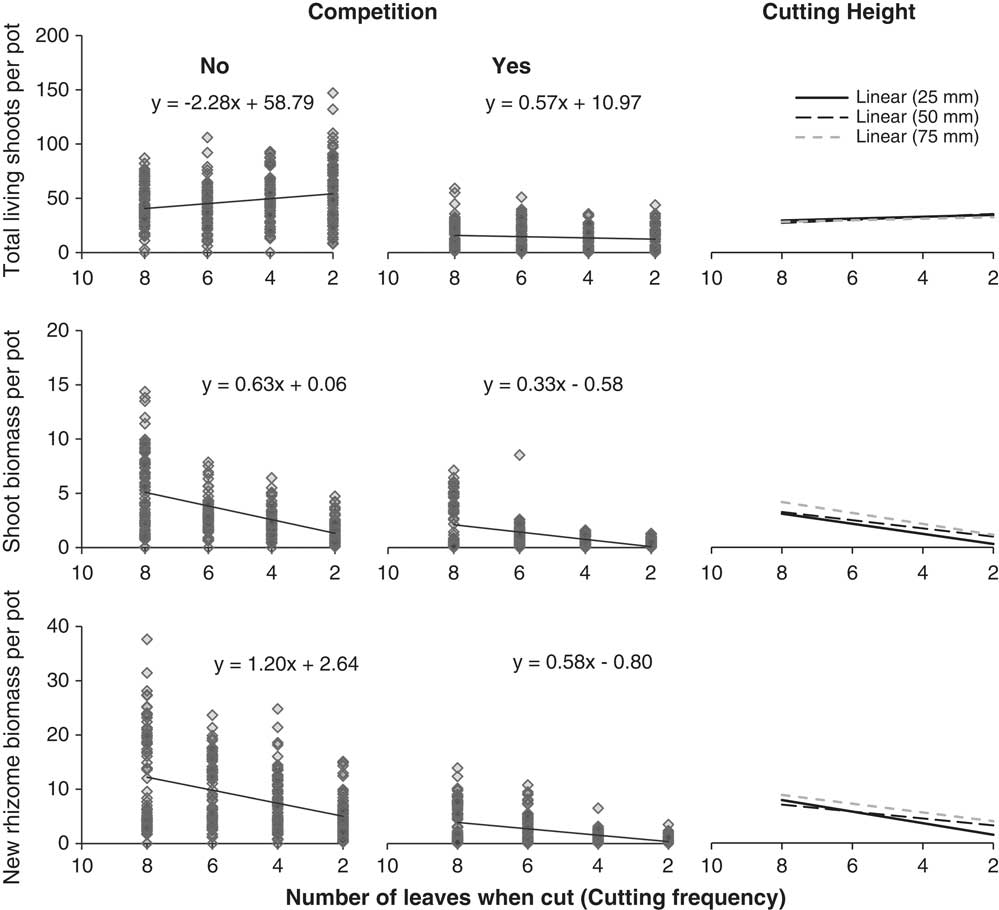
Figure 1 Results from the regression analysis between the number of quackgrass leaves when the plants were cut (cutting frequency) and quackgrass’s total living shoots (top), shoot biomass (middle), or new rhizome biomass (bottom) at the end of the experiment. Results are divided by competition (left) and cutting height (right). Effect of rhizome fragmentation is not shown, since it was not significant (Table 3).
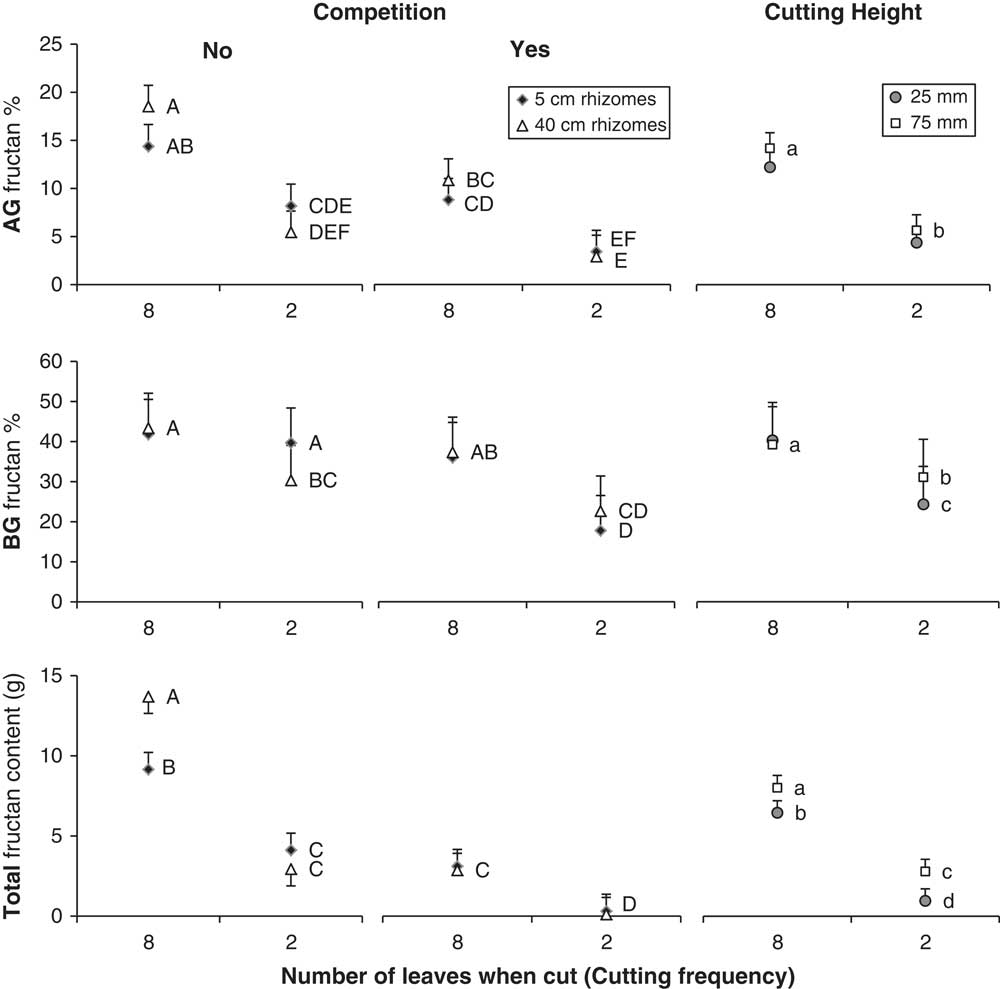
Figure 2 The fructan concentration in quackgrass’s aboveground (AG) biomass (top) and belowground (BG) biomass (middle), and total fructan content (bottom). Results are divided by competition, cutting height and frequency, and rhizome fragmentation degree. Letters indicate significant differences from Tukey’s HSD tests.
Table 4 Main effect of clover competition, rhizome fragmentation degree, shoot-cutting frequency, and cutting height on quackgrass total shoot numbers, new rhizome and shoot biomass, fructan concentration in belowground (BG) and aboveground (AG) biomass, and total fructan content at the final sampling (Table 1).a

a Numbers are means ±95% confidence intervals. Means are calculated over both 2012 and 2013 for the total number of shoots and the new rhizome and shoot biomass, but fructan was only sampled in 2013. Missing values are due to the fact that only the highest and lowest fragmentation degree, shoot-cutting frequency, and cutting height were used in the fructan concentrations and content analyses. When values were square-root (sq. root) transformed in the analysis, the table shows retransformed values. Letters denote significant differences within columns and within the categorical variables competition (Student’s t-test), rhizome fragmentation degree, shoot-cutting frequency, and shoot-cutting height (Tukey’s HSD test).
Rhizome fragmentation did not have a significant effect on quackgrass rhizome biomass acquisition but influenced fructan acquisition and final shoot number (Table 4). In the treatments with no clover competition and the lowest shoot-cutting frequency, fragmentation resulted in 33% lower total fructan content (Table 3; Figure 2). Moreover, in pots with no clover competition, the treatment with one 40-cm rhizome had a lower belowground fructan concentration than the eight 5-cm rhizomes treatment when shoot cutting was performed most frequently (at 2 leaves) compared with least frequently (at 8 leaves) (Table 3; Figure 2). The intermediate fragmentation degrees (two 20-cm rhizomes and four 10-cm rhizomes) had the fewest number of total shoots, but only the treatment with four 10-cm rhizomes was significantly lower by the fall compared with the two extremes of fragmentation (Table 4).
Hypothesis 2, which stated that the combination of clover competition, more frequent and lower shoot cutting, and a higher degree of rhizome fragmentation would reduce quackgrass biomass and fructan acquisition, was supported for clover competition and shoot cutting, but not for rhizome fragmentation. The results indicate that clover competition not only reduces quackgrass biomass acquisition, as seen in previous studies (e.g., Ringselle et al. Reference Ringselle, Prieto-Ruiz, Andersson, Aronsson and Bergkvist2017), but also the fructan concentration of its rhizomes. Fructan accumulates when light conditions promote photosynthesis, but plant growth is inhibited (Livingston et al. Reference Livingston, Hincha and Heyer2009). Thus, by competing for light, the white clover may have prevented the buildup of fructans. This can be contrasted with shoot cutting, which is suggested to reduce fructan concentrations, because regrowth is competing with reserve storage (Pollock Reference Pollock1984). However, from the present study, it is not possible to conclude whether the decrease is linear with increasing crop biomass and whether the crop’s mode of competition (e.g., primarily light or nutrients) has a strong influence on the fructan concentration.
The results indicate that quackgrass biomass acquisition is reduced more by shoot cutting when it is performed frequently with a low cutting height. Similarly, Ferraro and Oesterheld (Reference Ferraro and Oesterheld2002) found that a high cutting frequency and low recovery time between cuttings makes it more difficult for plants to compensate for the loss of biomass. Lowering the cutting height does not seem very effective in the low-frequency shoot-cutting treatment, but that could also be because the low-intensity treatment was not only performed less frequently, but also later in the season (at leaf stage 6 or 8) (Bergkvist et al. Reference Bergkvist, Ringselle, Magnuski, Mangerud and Brandsæter2017). The increase in total shoots due to shoot cutting is congruent with an increase in tillering caused by repeated cutting (Håkansson Reference Håkansson1969). This response is most likely due to an increase in light reaching quackgrass crown, particularly as the response was absent in pots with white clover.
In this experiment, rhizome fragmentation had no detrimental effect on quackgrass biomass acquisition but did reduce quackgrass total fructan content, especially in the aboveground biomass (Figure 2). This suggests that the total photoassimilate production of the plants in the treatment with eight 5-cm rhizomes was the same as in the treatment with one 40-cm rhizome, but that they produced more of something other than fructan. Thus, if what they produced is not as easily metabolized as fructan (e.g., cellulose), this may result in a reduced re-translocation of resources from the aboveground biomass to the rhizomes in fall.
A number of reasons can explain why quackgrass biomass acquisition was unaffected by rhizome fragmentation. The beneficial growing conditions (shallow planting, nutrient-rich soil, and the additional nitrogen added to avoid nutrient deficiency) used in the experiment may have mitigated the competitive advantage of larger rhizome fragments having more food reserves per fragment. Håkansson (Reference Håkansson1971) found that rhizome burial at 2.5- to 7.5-cm depth is optimal for aboveground shoot growth. So, the 5-cm planting depth used in the study is unlikely to have been detrimental, even with the highest fragmentation degree. In the same manner, the high nutrient content of the soil may have benefited plants in pots with a high fragmentation degree more than those from pots with a lower fragmentation degree. Turner (Reference Turner1966) found that a low nitrogen availability is more detrimental to smaller rhizomes than larger rhizomes with respect to new rhizome production. If nitrogen availability is a determining factor for whether rhizome fragmentation results in less-competitive quackgrass shoots, that may also explain why small and large rhizomes did equally well under competition from an nitrogen-fixating crop such as white clover. Clovers can compete well with quackgrass, even without intense nitrogen competition (Ringselle et al. Reference Ringselle, Prieto-Ruiz, Andersson, Aronsson and Bergkvist2017). In contrast, Håkansson (Reference Håkansson1971) reported that white mustard (Sinapsis alba L.), which can compete heavily for nitrogen, suppressed smaller quackgrass rhizome pieces more than larger ones. Nitrogen availability may also have affected the interaction between shoot cutting and rhizome fragmentation. Turner (Reference Turner1966) found that a high nitrogen availability caused a greater shoot growth in the plants from with a low fragmentation degree than a high fragmentation degree. This greater shoot growth resulted in a greater reduction in old rhizome weight when shoot cutting was applied.
Another explanation for the lack of a fragmentation effect on quackgrass rhizome biomass is that fragment weight was a more important explanatory variable than fragment length. The initial fresh weight was correlated with the final new rhizome weight, belowground fructan concentration, and number of tillers (Table 3). Rhizome age and exposure of soil tillage, including the number of operations, also affect subsequent growth potential (Håkansson Reference Håkansson2003). The rhizomes used in our study were of similar age and had only been harrowed once (2013) or twice (2012) before being collected. Previous studies show that one or two harrowing operations, without plowing afterward, do not severely limit the growth potential of quackgrass (Håkansson Reference Håkansson2003; Thomsen et al. Reference Thomsen, Mangerud, Riley and Brandsæter2015). One limiting factor is that the study did not include a real nonfragmentation level, as even the treatment with one 40-cm rhizome was a form of fragmentation. Fragmenting the rhizomes of quackgrass plants in an established vegetation cover can be very detrimental to their biomass acquisition (Bergkvist et al. Reference Bergkvist, Ringselle, Magnuski, Mangerud and Brandsæter2017).
Implications for Management of Quackgrass in Grass–Legume Crops
This study shows how effective mowing in a clover crop can be for controlling quackgrass when the cutting frequency is high and shoots are cut close to the soil surface. This supports studies in which few cuttings made with a relatively high stubble height in a full-season grass–clover promoted quackgrass proliferation (Melander et al. Reference Melander, Rasmussen and Olesen2016; Rasmussen et al. Reference Rasmussen, Melander, Askegaard, Kristensen and Olesen2014). However, the high efficacy may also have been partly due to shoot-cutting timing. In experiments by Bergkvist et al. (Reference Bergkvist, Ringselle, Magnuski, Mangerud and Brandsæter2017), mowing efficacy on quackgrass’s rhizomes was up to 95% when conducted from June to August–September, while it had no significant effect when conducted from August–September to October. Also, in crops that are more sensitive to mowing than white clover, the control effect on quackgrass must be balanced against the damage it will cause to the crop.
This study does not provide any evidence that rhizome fragmentation significantly contributes to quackgrass management. Rhizome fragmentation did affect quackgrass shoot numbers, main shoot–tiller ratio, and total fructan content, but did not directly reduce biomass acquisition. However, clonal integration generally benefits perennial plants (Song et al. Reference Song, Yu, Keser, Dawson, Fischer, Dong and van Kleunen2013), and previous findings make it clear that, under some circumstances, rhizome fragmentation is definitively detrimental to quackgrass (e.g., great soil depth, low nitrogen availability). As rhizomes in the field can vary greatly in terms of their environmental conditions and location in the soil, they will therefore likely respond differently to rhizome fragmentation. Given good growing conditions, as in this experiment, fragmented rhizomes are seemingly as productive as whole rhizomes. In poorer conditions, plants from larger rhizomes may have an advantage. Moreover, the lower fructan content due to rhizome fragmentation could have an effect on quackgrass competitiveness. Therefore, we need to clarify under which circumstances rhizome fragmentation weakens quackgrass, so that rhizome fragmentation can be effectively applied in quackgrass management.
The success of nonchemical weed control of creeping perennials, including both rhizomatous species and species with creeping roots such as Canada thistle [Cirsium arvense (L.) Scop.]., largely depends on which crops are included in the crop rotation. For instance, grasslands in rotations may decrease the abundance of Canada thistle but will often not decrease a population of quackgrass (Håkansson Reference Håkansson2003). However, the present study shows there can be large differences between management strategies within the same crop. Type of implements, settings, and timing of the treatment, both within season (e.g., within the fall [Ringselle et al. Reference Ringselle, Bergkvist, Aronsson and Andersson2016]), but also between seasons (e.g., autumn vs. spring [Brandsæter et al. Reference Brandsæter, Mangerud, Helgheim and Berge2017]) is crucial for optimal weed control.
Acknowledgments
This study was facilitated by experimental, statistical, and secretarial services at the Norwegian Institute for Agricultural and Environmental Research (Bioforsk) (now NIBIO, Norwegian Institute of Bioeconomy Research) and the Department for Ecology and Natural Resource Management at the Norwegian University of Live Sciences. Thanks to Yara Norge AS for providing partial financial support. The work was also partly funded by EU-FP7 as part of OSCAR (Optimizing Subsidiary Crop Applications in Rotations) and by the Research Council of Norway through the project “Sustained and Increased Organic Cereal Production by Improved Nutrient Supply and Pest Control.” The authors thank Johannes Forkman and Torfinn Torp for help and discussion regarding the statistical analyses.