Impact statement
The significant financial and societal impacts of drought events on agriculture are not solely limited to arid and semi-arid regions, but increasingly occur in more temperate and humid regions. This review characterises the sensitivity of the main agricultural and horticultural farming sectors, focussing on the UK, to different types of drought, considering both impacts and the short- to long-term responses, to draw out the salient knowledge gaps to inform future research priorities, data needs, and practitioner and policy priorities. Despite recognition of the agronomic and socio-economic risks, there remains poor quantitative and empirical explanatory evidence of the multi-scalar impacts of drought, ranging from the impacts on production (yields and quality) to the financial implications for farm businesses, and the consequences for fresh produce supply chains and consumers. Drought impacts are exacerbated by highly competitive financial environments that drive farm business decision-making strategies to increase the economic efficiency of production to the detriment of system redundancy, diversity and resilience to drought. In humid-temperate climates, increasing drought risk carries costs that in most years do not return financial benefits. Enablers such as enhancing social capital and collaboration, therefore, need to be better understood. The reactive responses of most farmers to drought are partly due to a lack of information on expected changes in environmental conditions. There remain key knowledge gaps regarding the forecast lead times and reliability of drought monitoring and early warning (MEW) systems that are required to influence business decisions in such variable climate regimes. Finally, future changes in agroeconomic policy, dietary and market needs, climate and water resources availability and regulation will profoundly change the spatial nature and composition of agricultural and horticultural production. Agricultural futures that are co-developed with representatives from across the primary production and food supply chain sectors are needed to inform future research needs and policy priorities to support sustainable agricultural transformation.
Introduction
Agriculture is often cited as one of the most drought-sensitive economic sectors (e.g., Wilhite, Reference Wilhite, Sivakumar and Motha2007), with crop failure, reduced yields, livestock loss and reduced fertility, soil degradation and agricultural abandonment being widely reported, particularly in arid and semi-arid climates (Howitt et al., Reference Howitt, Medellin-Azuara, MacEwan, Lund and Sumner2014; FAO, 2017). However, such drought impacts are not only limited to arid and semi-arid regions (e.g., Azadi et al., Reference Azadi, Keramati, Taheri, Rafiaani, Teklemariam, Gebrehiwot, Hosseininia, Van Passel, Lebailly and Witlox2018; Kuwayama et al., Reference Kuwayama, Thompson, Bernkopf, Zaitchik and Vail2019), but increasingly occur in more temperate and humid regions where droughts have not been previously posed a major risk to agricultural production and food security (Europe: Bachmair et al., Reference Bachmair, Kohn and Stahl2015; UK: Rey et al., Reference Rey, Holman and Knox2017; Parsons et al., Reference Parsons, Rey, Tanguy and Holman2019).
Wilhite and Glantz (Reference Wilhite and Glantz1985) classified the many definitions of drought at the time into four basic approaches with three being considered as a ‘physical’ phenomenon (meteorological, agricultural and hydrological) and one as a socio-economic phenomenon (e.g., where reduced water availability leads to a reduction in hydro-electric power generation). Whilst the term ‘agricultural drought’, which refers to the impacts of low rainfall levels leading to low soil moisture content, is often used to mean ‘drought affecting agriculture’ much research has sought to find relationships between indicators of meteorological drought (prolonged periods of low rainfall) and agricultural impacts such as crop yield deviations (e.g., Bachmair et al., Reference Bachmair, Tanguy, Hannaford and Stahl2018; Vergni et al., Reference Vergni, Vinci and Todisco2021), qualitative impact reports (e.g., Stagge et al., Reference Stagge, Kohn, Tallaksen and Stahl2015; Parsons et al., Reference Parsons, Rey, Tanguy and Holman2019) or remotely sensed vegetation indices (e.g., Bachmair et al., Reference Bachmair, Tanguy, Hannaford and Stahl2018; Xu et al., Reference Xu, Wang, Zhao and Yang2018). However, the spatial differences in correlation and the most influential drought indicators and accumulation periods (e.g., Stagge et al., Reference Stagge, Kohn, Tallaksen and Stahl2015; Parsons et al., Reference Parsons, Rey, Tanguy and Holman2019) are indicative of an uncharacterised spatially varying drought sensitivity within agriculture which may be a consequence of different farming systems and their ability or willingness to invest in adaptation, irrigation utilisation, or adaptive capacity and its dependence on business scale.
Farming systems in the UK are highly diverse in terms of specialism (livestock to mixed to arable); management intensity (extensive upland to intensive lowland farms); water-dependency (rainfed to irrigated); environmental conditions (outdoor farming to protected cropping in polytunnels and glasshouses; outdoor and indoor livestock); business size (small family farms to large agribusinesses) and so forth. The UK has also experienced a number of significant droughts over the last five decades including 1975–1976 (Dornkamp et al., Reference Dornkamp, Gregory and Burn1980), 1988–1992 (Bryant et al., Reference Bryant, Arnell and Law1994), 1995–1997 (POST, 1995), 2003 (Marsh, Reference Marsh2006), 2004–2006, 2010–2012, 2018 (Turner et al., Reference Turner, Barker, Hannaford, Muchan, Parry and Sefton2021) and 2022. Studies have evaluated U.K. drought impacts and responses in agriculture but these have tended to be either generalised (e.g., Parsons et al., Reference Parsons, Rey, Tanguy and Holman2019; Holman et al., Reference Holman, Hess, Rey and Knox2021) or focused on specific sub-sectors, for example, irrigated agriculture (Hess and Knox, Reference Hess and Knox2013; Rey et al., Reference Rey, Holman and Knox2017) and outdoor livestock (Salmoral et al., Reference Salmoral, Ababio and Holman2020). There remains a need to better understand how drought affects the sector and its different key farming systems.
Consequently, this review, focussing on the dominant agricultural and horticultural production systems within the UK, aims to characterise their sensitivity to the different types of drought, considering both impacts and responses, to draw out the key knowledge gaps from both research and policy perspectives. Although focussing on the UK, the insights and recommendations are directly relevant to informing drought risk management in other temperate and humid regions.
Overview of U.K. agriculture and horticulture
Agriculture, as measured by the utilised agricultural area (UAA), covers 71% or 17.2 million hectares of the UK, with 6.1 million hectares being croppable (Defra, 2021) and 99% being rainfed (Hess et al., Reference Hess, Knox, Holman and Sutcliffe2020). The significant spatial variability in the U.K.’s agroclimate, topography and soils has resulted in multiple different outdoor and protected farming systems resulting in large regional differences in the distribution of crops and livestock (Figure 1) and in the spatial distribution of different agricultural sub-sectors including livestock, arable (cereals), outdoor field scale horticulture and indoor (protected) horticulture (Figure 2). For example, the milder, wetter maritime-influenced areas (SW and NW England, Wales and Northern Ireland) support near three quarters (74%) of the dairy industry which relies extensively on good grassland production for forage, in contrast to the colder and wetter upland areas of Wales that contain almost 30% of the U.K. sheep and lamb herds. The drier Eastern and Midlands regions are dominated by combinable cropping with arable and high-value field vegetable production. In SE England, due to the continental climatic influence, nearly 40% of both orchard fruits (e.g., apples and pears) and small fruits (e.g., strawberries) are concentrated.

Figure 2. Spatial distribution of main farming systems in the UK based on Eurostat (2016) data, expressed as regional percentage of national farm numbers of a given farm type, for (upper left) specialised livestock farms (dairy, beef and lamb, pigs and poultry), (upper right) specialist cereal farms, (lower left) specialist outdoor horticulture (vegetables and fruit) and (lower right) specialist indoor horticulture. (Source: Office for National Statistics licensed under the Open Government Licence v.3.0; Contains OS data © Crown copyright and database right 2023)
Drought impacts and adaptation in arable and livestock farming
Arable and livestock systems that rely on rainfall are the two dominant farming systems in the UK, but the climate means that they tend to be the most extensive farming types in the south/east and west/north of the U.K., respectively Figure 2). There are much fewer drought studies related to livestock systems in the UK compared to crop-based systems, despite international understanding of the impacts of high temperatures and drought on grass yield and quality (e.g., Sheaffer et al., Reference Sheaffer, Peterson, Hall and Stordahl1992); animal productivity (quality and quantity of meat, milk, wool) and livestock fertility and health, particularly in cows (e.g., De Rensis and Scaramuzzi, Reference De Rensis and Scaramuzzi2003; Alves et al., Reference Alves, Gonçalves, Pavão, Palazzi, Souza, Queiróz and de Achilles2013).
Meteorological drought
Wreford and Topp (Reference Wreford and Topp2020) suggested that rainwater harvesting (RWH) and storage, which is sensitive to meteorological drought, may be used in some livestock farms but there were no cited studies.
Agricultural (soil moisture) drought impacts
All rainfed agricultural systems in the UK are sensitive to the impacts of soil moisture drought as there is little opportunity for reactive adaptation (Rial-Lovera et al., Reference Rial-Lovera, Davies and Cannon2017). However, the impacts on productivity (yield and quality: Knox et al., Reference Knox, Morris and Hess2010; Keay et al., Reference Keay, Jones, Hannam and Barrie2014) differ between individual drought events (depending on the timing, duration and severity), between individual crop types (including fodder crops), target markets (whether crops are destined for processing or pre-pack [fresh]) and between crop and grass varieties (due to differences in drought tolerance).
Wheat is less sensitive to drought than barley but has greater sensitivity than rye and triticale (Cho et al., Reference Cho, Falloon, Gornall, Betts and Clark2012). Although most wheat is cultivated under rainfed conditions, around 25% (Foulkes et al., Reference Foulkes, Sylvester-Bradley and Scott2001a) of the U.K. wheat crop is grown on shallow or sandy soils which are easily droughted. Yield losses from drought can range between 2 and 4.5 t/ha (Foulkes et al., Reference Foulkes, Scott and Sylvester-Bradley2001b), with modelled historic yield losses for cv. Claire near Cambridge due to key drought events ranging from 15% in 1943 to 29% in 1976 and 38% in 1921 (Clarke et al., Reference Clarke, Hess, Haro-Monteagudo, Semenov and Knox2021). The importance of cultivar and drought timing was shown in a lysimeter study by Dickin and Wright (Reference Dickin and Wright2008), who found that yields were reduced by drought from growth stage (GS) 61 by 17% (cv. Xi-19) to 24% (cv. Deben), whereas drought from GS 45 reduced yields from cv. Claire by 53%.
Within crops, genetic improvements due to crop breeding coupled with changes in agronomy also makes estimating yield loss to drought problematic when separating natural variability from drought response (Talbot, Reference Talbot1984; MacKay et al., Reference Mackay, Horwell, Garner, White, McKee and Philpott2011). Mechler et al. (Reference Mechler, Hochrainer, Aaheim, Salen and Wreford2010) suggested that decreasing deviations from a 5-year moving average of yield during drought years in oilseed rape, unlike barley, was evidence of adaptation but may have reflected the most severe (1976) drought being at the beginning of the time series.
Notwithstanding impacts on yield, food crops have minimum quality assurance standards that are required for their target market and drought during key growth phases may adversely affect the post-harvest quality, shelf life (OECD, 2018; UK Flour Millers, 2023) and thus price. However, there are very few studies that have assessed drought impacts on crop quality (e.g., Rey et al., Reference Rey, Holman, Daccache, Morris, Weatherhead and Knox2016).
The majority of studies investigating drought impacts in U.K. outdoor livestock systems have assessed the impacts of soil moisture drought on fodder and forage crops. Perennial ryegrass (Lolium perenne L.) is the most important grass species in Britain, being a major constituent of both permanent and temporary grassland but is drought sensitive (Lambert et al., Reference Lambert, Van der Veeren, Decamps, Cremer, De Toffoli and Javaux2020). Grass growth during the 1976 drought was negligible in the south of England with many areas completely desiccated and animals needing supplementary feeding (Roy et al., 1978). Lee et al. (Reference Lee, Howard-Andrews and Chester2019) found that drought caused a reduction in productivity in perennial ryegrass, but there was substantial variation between varieties (up to 82%), with the optimal variety changing depending on drought severity. Grass yields in dry years can be increased through inclusion of other grasses (Lambert et al., Reference Lambert, Van der Veeren, Decamps, Cremer, De Toffoli and Javaux2020) and legumes (Kuchenmeister et al., Reference Kuchenmeister, Kuchenmeister, Kayser, Wrage-Monnig and Isselstein2013). Forage maize (Zea mays L.) is an increasingly important fodder crop for intensive production systems, which requires 300–400 mm from May to October in southern England (Bunting, Reference Bunting, Bunting, Pin, Phipps, Wilkinson and Gunn1978) and is reported to have medium–high sensitivity to drought (Brouwer and Heibloem, Reference Brouwer and Heibloem1986) but a good yield response to irrigation (Klocke et al., Reference Klocke, Payero and Schneekloth2007, Reference Klocke, Currie, Kisekka and Stone2014).
Agricultural practices can also increase the drought sensitivity of grassland, with reductions in soil water retention in improved grassland compared to neighbouring semi-natural grassland being ascribed to soil compaction (Wallace and Chappell, Reference Wallace and Chappell2020), which is common in some grassland systems (Palmer and Smith, Reference Palmer and Smith2013). Irrigation is currently not commonly used in grassland systems in the UK to reduce drought sensitivity (Wreford and Topp, Reference Wreford and Topp2020), but the impacts of drought on permanent pasture in Wallace and Chappell (Reference Wallace and Chappell2020) were offset by the addition of moisture from summer slurry applications which enabled continued sward growth and reduced sward damage. Future changes in climate with increased aridity and drought risk will inevitably change land suitability for some crops (Daccache et al., Reference Daccache, Keay, Jones, Weatherhead, Stalham and Knox2012) and the economic viability of irrigation for crops that were previously rainfed including grassland and cereals.
Surprisingly, there are very few studies that have investigated the indirect impacts of drought on U.K. livestock and meat and milk production. Salmoral et al. (Reference Salmoral, Ababio and Holman2020), combining analysis of U.K. national farmers magazines with farmer interviews in Derbyshire, showed that the reported impacts of drought were overwhelmingly negative and spanned pasture growth and reduced yields, feed and bedding availability, animal growth and welfare, milk production, financial performance and farmer wellbeing. Wreford and Adger (Reference Wreford and Adger2010) analysed the deviation from the 5-year moving average around historic drought years in U.K. cattle, sheep, pig and poultry production, and showed that sheep and cattle production tended to both increase and decrease whilst pig and poultry production mostly decreased. However, they recognised that their method could not absolutely separate the effects of drought, policy changes and farmer responses. Mechler et al. (Reference Mechler, Hochrainer, Aaheim, Salen and Wreford2010) similarly found livestock production to have a varying response to drought/heat wave years over time and that it was difficult to separate the effects of weather on livestock production from policy and/or other shocks. Similarly, in their study of heat stress impacts on milk yields, Dunn et al. (Reference Dunn, Mead, Willett and Parker2014) concluded that their observed changes could be the combined effects of reduced pasture quality due to drought associated with the hot weather and heat stress in the animals.
Hydrological drought impacts
Livestock farms require access to reliable supplies of water as animals need a regular daily water supply to regulate body temperature and maintain organ functions (Ward and McHague, Reference Ward and McHague2007). Drinking water for livestock is the largest agricultural use of water accounting for 41% of total use or 75 million m3, with a further 13 million m3 used for washing down (Defra, 2011). Consequently, they can be highly reliant on private boreholes and small water sources such as drainage channels, streams and springs to provide drinking water for cattle and sheep (Hess and Sutcliffe, Reference Hess and Sutcliffe2018; Salmoral et al., Reference Salmoral, Ababio and Holman2020) and to form ‘wet fences’ to control livestock movement (Holman et al., Reference Holman, Knox, Hess, McEwen, Salmoral, Rey, Hannaford, Grove, Thompson and Quinn2019).
In 1975, a set of designations for agricultural land use were developed to support special measures to assist farming in areas where local geographical conditions were particularly challenging. Within this designation, less favoured areas for agriculture include ‘severely disadvantaged’ and ‘disadvantaged areas’ and represent (mainly upland) areas where the natural characteristics including geology, altitude and climate make it difficult for farmers to compete. The proportion of farms abstracting water direct from surface water is significantly higher in farms in hill farming and mountainous areas (about 53% in ‘disadvantaged areas’ and about 69% in ‘severely disadvantaged areas’) compared to lowland farms (about 25%) (Defra, 2017), although no volumes were recorded. Consequently, such ‘less favoured areas’ grazing livestock farms used the lowest percentage of mains water of all farm types (Defra, 2017). Given that between 25 and 39% of sheep, beef cows and other cattle have access to watercourses (Defra, 2011), the drying up of such small water sources during droughts leads to significant livestock risks and costs to provide vital alternative water supplies. Riley et al. (Reference Riley, Potter, Biggs, Collins, Jarvie, Jones, Kelly-Quinn, Ormerod, Sear, Wilby, Broadmeadow, Brown, Chanin, Copp, Cowx, Grogan, Hornby, Huggett, Kelly, Naura, Newman and Siriwardena2018) considered that the high connectivity of small water bodies with their surrounding environment makes them particularly vulnerable to having highly modified discharge as a consequence of agricultural and forestry drainage, with implications for flood and drought control further downstream but make no mention of the associated hydrological drought risk to livestock-based agriculture. Consequently, the financial implications of the short-term failure of small water supplies during a hydrological drought, particularly in upland areas which could lead to a reduction in herd size through slaughter or sale of stock (Hess and Sutcliffe, Reference Hess and Sutcliffe2018), are unknown.
Drought responses
Research in the UK has shown similarities in reported drought responses within outdoor livestock farming at national and local scales that are largely reactive, crisis-driven and contribute to the hidden personal burden of extreme events on farmers (Salmoral et al., Reference Salmoral, Ababio and Holman2020). The main farm-level coping strategies reported from the 2018 drought were: (1) management of available grazing and feed; (2) selling livestock to reduce feed demands and improve cash flow and (3) purchase of additional feed (Salmoral et al., Reference Salmoral, Ababio and Holman2020). Additional short-term measures included government derogations or temporary prescription adjustments (TPAs) to relax certain regulations on farming activity; and private sector actions including flexible loan agreements from financial institutions; and modifications to retailers’ and processors’ contractual terms and specifications of livestock products (Salmoral et al., Reference Salmoral, Ababio and Holman2020). Feed purchases in 2018 were affected by socio-economic drought impacts as fodder import subsidies in the Republic of Ireland increased competition for domestic feed (Byrne, Reference Byrne2018).
Wreford and Topp (Reference Wreford and Topp2020) identify many longer-term adaptation options to variable precipitation and water availability for the livestock sector. For example, drought impacts may be reduced by introducing drought tolerant species (e.g., Neal et al., Reference Neal, Fulkerson, Lawrie and Barchia2009; Marshall et al., Reference Marshall, Collins, Humphreys and Scullion2016), incorporating legume and herb forage species that provide greater nutrition into pastoral systems (e.g., Kemp et al., Reference Kemp, Kenyon and Morris2010) or general species diversification (Kirwan et al., Reference Kirwan, Luscher, Sebastia, Finn, Collins, Porqueddu, Helgadottir, Baadshaug, Brophy, Coran, Dalmannsdóttir, Delgado, Elgersma, Fothergill, Frankow-Lindberg, Golinski, Grieu, Gustavsson, Höglind, Huguenin-Elie, Iliadis, Jørgensen, Kadziuliene, Karyotis, Lunnan, Malengier, Maltoni, Meyer, Nyfeler, Nykanen-Kurki, Parente, Smit and Thumm2007), but consideration needs to be given to growth patterns and production trade-offs (Lee et al., Reference Lee, Clark and Roche2013; Wreford and Topp, Reference Wreford and Topp2020). However, there is scant evidence of increased drought resilience resulting from adaptation within the outdoor livestock sector (Wreford and Adger, Reference Wreford and Adger2010; Salmoral et al., Reference Salmoral, Ababio and Holman2020). The majority of farmers interviewed by Salmoral et al. (Reference Salmoral, Ababio and Holman2020) reported no measures being implemented or considered to reduce the impact of future droughts. Wreford and Adger. (Reference Wreford and Adger2010) suggested declining production impacts of recurring U.K. droughts were indicative of evidence of adaptation within pigs and poultry production, although the national scale of analysis does not allow for the differing regional nature of the droughts or balance of indoor versus outdoor production systems. Wreford and Adger (Reference Wreford and Adger2010) did not look at dairy production due to the impacts of changing policy on production.
Protected cropping
Protected cropping (Figure 2) can include both edible and non-edible produce and relates to crops which are grown under polythene or glass. The area of protected cropping under glasshouses and polytunnels (including Spanish polytunnels) has increased greatly in recent years, particularly in the soft fruit sector. In the last Glasshouse Survey (Defra, 2007), a fifth (22%) of the total protected area was under polythene but this is expected to have increased significantly in both area and proportion since. There are currently around 800 ha of protected fresh vegetables, worth £374 M, and 3,000 ha of glasshouse crops (Defra, 2021). However, an unknown proportion of the 12,000 ha of plant and flower production, worth £1,137 M, and 10,000 ha of soft fruit worth £629 M (Defra, 2021), is grown under protection. However, 81% of hardy nursey stock growers used irrigation, based on the 2005 irrigation survey (Knox et al., Reference Knox, Morris and Hess2010). Earlier estimates of the proportion of strawberries (the main soft fruit) grown under polytunnels include grower estimates of at least 70% (Evans, Reference Evans2013); whilst Calleja et al. (Reference Calleja, Ilbery and Mills2012) estimated that 81.3% of the strawberry crop on ‘large farm enterprises’ was grown under polytunnels in 2009. Defra (2011) estimated that 9 ± 2% of farms use rainwater storage, but no indication of use is provided, although irrigation using collected rainwater was estimated at 442,000 m3 in 2010. Mains water supply, which makes up about 1% of total irrigation water for agriculture, is mostly used on protected edibles cropping to ensure reliability of supply and to minimise risks associated with low microbiological water quality (Hess et al., Reference Hess, Knox, Holman and Sutcliffe2020).
Impacts of drought
Salad crops are increasingly grown hydroponically using artificial substrates (coir) or by nutrient film technique (NFT), whilst the U.K. soft fruit and ornamental horticultural industry are increasingly using container grown systems, including bags, buckets, troughs and modules (Atwood, Reference Atwood2013). The underlying drought sensitivity of protected cropping stems from their complete reliability on irrigation and, particularly in container or pot-based production, the smaller soil volumes available for water storage mean the production process is particularly susceptible to short-term drought stress (Lea-Cox et al., Reference Lea-Cox, Ross and Teffeau2001). These are also all high-value sectors that are entirely reliant on irrigation to maintain yields and quality (Rey et al., Reference Rey, Holman and Knox2017). Increasing pressures to reduce the volumes of water used due to rising costs for mains water, reduced availability of water in some catchments and changes to abstraction licencing combined with concerns regarding runoff and enhanced downstream flood risk (Entec, 2006; Centre for Rural Research, 2008), has led to growing interest in switching to RWH as an alternative source of supply. This can be as a sole water source, or in combination with surface water abstraction, groundwater abstraction or mains water supplies (Dunn and Adlam, Reference Dunn and Adlam2019).
Protected cropping can therefore potentially be vulnerable to meteorological drought when rainfall supplies (frequency and amount) are insufficient for RWH to meet irrigation needs; and hydrological drought if abstraction licences are constrained through abstraction restrictions and unable to meet irrigation need. Although crops grown under protection and container-grown crops have an exemption from the Water Resources Act (1991) Section 57 emergency drought restriction on surface water abstraction (AHDB, 2022), abstraction licences for irrigation of protected cropping can be subject to so-called Hands-off Flow (HoF) conditions. However, due to the high financial value of protected crops (Rey et al., Reference Rey, Holman and Knox2017) and their very high sensitivity to water stress, such businesses are likely to have back-up from groundwater and/or mains water. There is also a statutory exemption from Drought Order restrictions for irrigating plants that are grown or kept for sale for commercial use using mains water, reducing the likelihood of supply restrictions. Consequently, the direct impacts of drought are likely to be felt through reduced product quality and increased production costs, but there is little science or industry published information on drought impacts on this sub-sector.
Responses to drought
The protected cropping sector is entirely dependent on irrigation and therefore most vulnerable to hydrological droughts and the consequences of partial or total restrictions on direct abstraction, due to declining river levels and low flows. As a response to the increasing likelihood of drought-imposed abstraction restrictions, many businesses have invested in alternate sources of supply to spread the risk and reduce their system vulnerability. These include investing in on-farm storage reservoirs as an insurance policy to buffer peak irrigation demand periods when abstraction could be restricted. RWH is also increasing in popularity (Environment Agency, 2009), with runoff from polytunnels and glasshouses being diverted into farm reservoirs. Because many soft fruit businesses have recognised the potential resource and drought response benefits of RWH but have lacked access to drought risk-based tools to inform their investment decision making, Holman et al. (Reference Holman, Knox, Panigrahi and Hess2022) developed a nationally-applicable tool to evaluate the hydrological viability and reservoir storage requirements for RWH for soft fruit, ornamentals and salad crops growers.
Outdoor irrigated cropping
Unlike many cereal crops which are milled or processed prior to sale, the value of fresh produce is strongly determined by visual aspects and buyer protocols which define stringent requirements relating to colour, size, shape and skin finish (Hingley et al., Reference Hingley, Lindgreen and Casswell2006; OECD, 2018). Consequently, controlling soil moisture through supplemental irrigation becomes critical for growing high quality, high-value fresh produce in drier regions of the country (Figure 2), and for meeting the consistent supplies of produce demanded by the retailers. Irrigation was second largest user of water in agriculture accounting for 38% of the total volume used or 70 million m3 (Defra, 2011), although this volumetric demand varies significantly from year-to-year, both spatially and temporally, with recent estimates for ‘dry year’ irrigation demand in Eastern England being 140,000,000 m3 (Knox et al., Reference Knox, Haro-Monteagudo and Morris2018). Main crop potatoes and vegetables for human consumption dominate the irrigated area (38% and 25%, respectively) and volumes applied for irrigation (48% and 26%) in 2010 (Defra, 2011). U.K. potato and outdoor vegetable production were estimated to be worth £820 M and £1,320 M, respectively, in 2020 (Defra, 2021), although not all production is irrigated. High-value irrigated vegetable cropping typically abstracts 160,000,000 m3 in a dry year (Weatherhead et al., Reference Weatherhead, Knox, Hess and Daccache2015) with half the total irrigated area and 57% of the total volume of water applied concentrated in the EA Anglian region (corresponding approximately to Eastern region in Table 1).
Table 1. Sensitivity of farm types to different types of drought (adapted from Holman et al., Reference Holman, Knox, Hess, McEwen, Salmoral, Rey, Hannaford, Grove, Thompson and Quinn2019)

Impacts of hydrological drought
The emphasis of retailers’ on quality standards within the fresh produce supply chain has resulted in supplementary irrigation becoming an essential component of production for quality assurance and to ensure the viability and profitability of key crops, including potatoes, field-scale vegetables and orchard fruit, in some regions (Vicario et al., Reference Vicario, Holman, Sutcliffe and Hess2023). Rey et al. (Reference Rey, Holman, Daccache, Morris, Weatherhead and Knox2016) estimated that the on-farm net financial benefits of irrigation for these outdoor crops that would be lost in a ‘design’ dry year if irrigation in England Wales were banned were around £204 million, reflecting the crop-specific loss of quality and yield benefits. Anglian Water and the University of Cambridge (2013) reported that drought in 2011 led to an estimated 15% reduction in potato yield.
However, abstraction of water for agricultural irrigation has the lowest priority for water allocation in the UK under drought conditions to protect public water supplies and the environment (Salmoral et al., Reference Salmoral, Rey, Rudd, de Margon and Holman2019). Salmoral et al. (Reference Salmoral, Rey, Rudd, de Margon and Holman2019) probabilistically estimated the national- and regional-scale economic impacts arising from mandatory abstraction restrictions (Section 57 restrictions) within drought management plans (taking account of catchment-specific river flows and irrigated crop areas). However, these estimates did not reflect the impacts arising from any irrigation constraints which would have been imposed by HoF conditions which are stated on many abstraction licences.
Responses to drought
Agriculture’s low priority for water during drought partly reflects perceptions that the financial value of water use in agriculture and horticulture is relatively low compared to other sectors despite high financial benefits per unit of water applied for some crops (Rey et al., Reference Rey, Holman, Daccache, Morris, Weatherhead and Knox2016), and that there is scope to increase the ‘efficiency of use’ of agricultural irrigation during drought conditions (Knox et al., Reference Knox, Kay, Hess and Holman2020). Whilst irrigating at night to reduce evaporation losses was the most common short-term coping strategy identified by Rey et al. (Reference Rey, Holman and Knox2017), changing irrigation schedules to either reduce irrigation depth over the full area or reduce the area irrigated were also common strategies (Knox et al., Reference Knox, Morris, Weatherhead and Turner2000), both of which lead to (uncertain) yield and quality penalties. Socio-economic drought impacts also hindered responses in 2018, when hiring or buying additional irrigation equipment was challenging due to a lack of equipment on the second-hand market and limited supplies of new irrigation equipment being imported due to the wider European drought (National Farmers Union (NFU), 2019).
In common with many other countries, the allocation of water for agricultural irrigation remains economically sub-optimal. The lack of regulatory flexibility to overcome the historic limitations of licence allocations (e.g., those awarded on a first come, first served basis) means that many irrigation licences have allocated water that is rarely or sometimes never abstracted (often referred to as ‘sleeper’ licences), even in drought years (Chengot et al., Reference Chengot, Knox and Holman2021); whilst other abstraction licences are often fully utilised and businesses have unmet demand. Multiple bureaucratic, regulatory, financial and timing constraints on water trading and contract options (Rey, Reference Rey2015; Rey et al., Reference Rey, Pérez-Blanco, Escriva-Bou, Girard and Veldkamp2019) limits their utility to address this mismatch in the UK.
Longer-term actions reported in Rey et al. (Reference Rey, Holman and Knox2017) included the development of a farm drought management plan to inform drought responses (76% of respondents), investment in alternative water resources and more efficient irrigation infrastructure (43%) and modification of crop choice and planting programmes (18%). There has also been major investment in on-farm storage reservoirs to synchronise the timing of abstraction with water availability (harvesting high flows) rather than irrigation need (National Farmers Union (NFU), 2015; Rey et al., Reference Rey, Holman and Knox2017), which may partly explain the perceived improvements in drought resilience within irrigated growers in easter England (Rey et al., Reference Rey, Holman and Knox2017).
Discussion
Given the complexity of the U.K. agricultural and horticultural sector, it is apparent from the review of evidence that sub-sectors have differing sensitivity to the different types of droughts, from meteorological drought to socio-economic drought (Table 1). Drought impacts on agriculture develop over multiple timescales and can persist, materialise or be realised long after rain has returned and the drought has been considered to have ended (Parry et al., Reference Parry, Wilby, Prudhomme and Wood2016). Such delayed impacts range from reduced crop yields and/or quality at harvest; shortages of stored fodder and bedding in the following winter; difficulty in filling on-farm water storage reservoirs affecting irrigation supply in the following growing season; to reduced livestock fertility affecting births in the following year; to economic impacts on business profitability and viability (Figure 3). Drought impacts can also be cumulative and often have unexpected ‘knock-on’ (i.e., secondary and tertiary) impacts that are difficult to track.
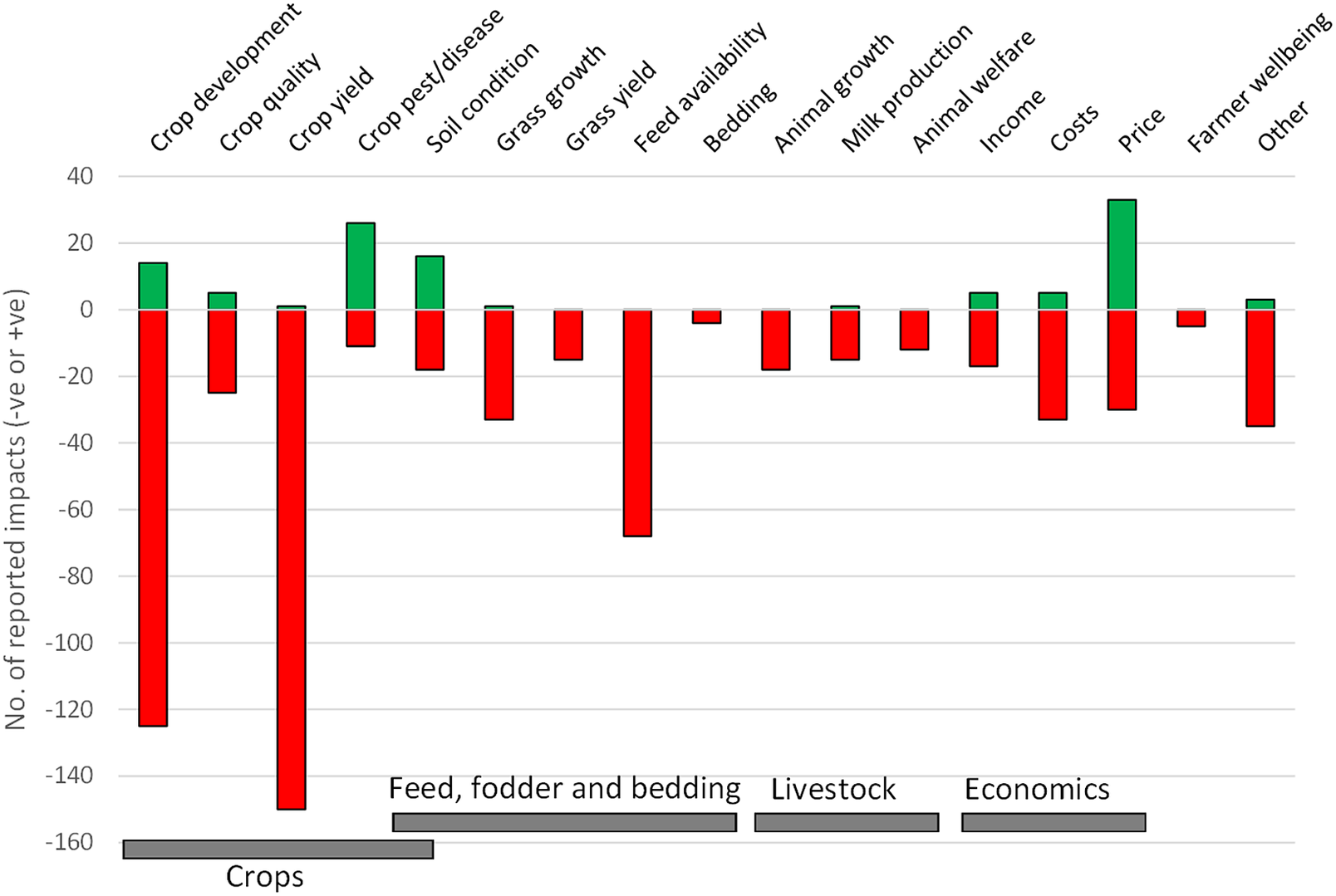
Figure 3. Negative (red) and positive (green) drought impacts reported in Farmers Weekly and Farmers Guardian trade magazines in 2018 (adapted from Holman et al., Reference Holman, Hess, Rey and Knox2021).
Because of these lags between the termination of a given drought event and some of the adverse consequences for farms and businesses, drought impacts in agriculture may be ignored, or forgotten, both by society and government (Holman et al., Reference Holman, Hess, Rey and Knox2021). However, the large economic damages associated with drought events, whether in the UK (e.g., Salmoral et al., Reference Salmoral, Rey, Rudd, de Margon and Holman2019), Spain (Perez and Barreiro-Hurle, Reference Perez and Barreiro-Hurle2009), Australia (Wittwer and Waschik, Reference Wittwer and Waschik2021) or the USA (Ziolkowska, Reference Ziolkowska2016) are expected to increase in both magnitude and frequency under a changing climate (Naumann et al., Reference Naumann, Cammalleri, Mentaschi and Feyen2021), emphasising the urgency to better understand and respond to drought in agriculture and horticulture. However, there are important uncertainties in our understanding, many of which will be shared with other humid-temperate climates. The key issues to emerge from this review relate to (i) the need to better understand the impacts arising from past droughts; (ii) assess the barriers and enablers for improving drought resilience, (iii) evaluate the potential of drought monitoring and early warning (MEW) systems and (iv) develop future scenarios and trends to forecast agricultural water demand.
Understanding past and current impacts
Despite agriculture’s widely acknowledged sensitivity to drought, there remains poor quantitative and empirical evidence of the impact of drought on U.K. farming. This includes understanding drought impacts on production (yields and, especially, quality) of crops, livestock and livestock outputs through to the financial costs to farm businesses, the supply chain and consumers. Consequently, there is a lack of baseline evidence to underpin operational and regulatory decisions and to demonstrate the need to pro-actively support agriculture and food supply chains to become more drought resilient (Table 2). This partly contributes to the largely reactive, short-term responses from regulators, farmers and the food industry that are seen during each drought event.
Table 2. Identified key knowledge gaps for understanding drought impacts within selected farming systems

The impacts of each drought are different due to the interaction of drought event characteristics (location and spatial extent; duration, timing and severity) with agriculture and horticulture’s drought vulnerability which differs regionally, between farming systems and between farms due to different soils, soil condition (Table 2: Q1), water sources (Q2), farming systems (crops or livestock) and target markets. Modelling assessments provide simplified insights into potential crop-specific yield losses due to drought but, for example, only one wheat cultivar on the U.K. recommended list to growers had been calibrated in the widely used Sirius wheat model (Clarke et al., Reference Clarke, Hess, Haro-Monteagudo, Semenov and Knox2021) that was estimated to be grown on 2% of the total U.K. wheat area.
The complex inter- and intra-farm differences mean that understanding the financial impacts of drought is challenging as multiple effects make up the aggregate drought impact, including drought-induced changes in productivity, disease burden, input costs and output prices (Salmoral et al., Reference Salmoral, Ababio and Holman2020; Clarke et al., Reference Clarke, Hess, Haro-Monteagudo, Semenov and Knox2021). All of these can change positively or negatively as a consequence of a drought, leading to a spatially complex mix of winners and losers at all scales. For example, crop yields can be adversely impacted by soil moisture stress or be increased due to higher radiation levels where soil moisture stress is low due to soil type, marginal climatic areas or due to irrigation. Consequently, regional droughts do not clearly affect national yields (Clarke et al., Reference Clarke, Hess, Haro-Monteagudo, Semenov and Knox2021) but often have significant regional economic damages and local impacts.
There are few robust assessments of the regional or national impacts of drought on crop production (Q3 and Q5) and financial damages in the UK due to lack of up-to-date data on, for example, polytunnels areas (Q3), water sources (Q4 and Q6), on-farm reservoirs (Q5 and Q9), irrigated crop areas (Q7) and irrigation application methods and scheduling approaches (Q8). It is difficult to separate out the impacts of soil moisture drought from restrictions on irrigation abstractions during a hydrological drought, but dry weather between 2010 and 2012 was estimated to have caused losses of £400 million to U.K. agriculture (Anglian Water, University of Cambridge, 2013) with losses in 2012 valued at £72 million to irrigated potato production in England (Akande et al., Reference Akande, Hussain, Knox, Hess, Hooftman, Stratford, Schafer, Acreman and Edwards2013). Knox et al. (Reference Knox, Morris, Weatherhead and Turner2000), Rey et al. (Reference Rey, Holman, Daccache, Morris, Weatherhead and Knox2016) and Salmoral et al. (Reference Salmoral, Rey, Rudd, de Margon and Holman2019) have all assessed the potential financial impacts of restrictions on irrigation abstraction, based on integrating the severity and timing of restrictions, irrigated cropping, crop prices and the crop-specific yield and crop quality benefits arising from supplemental irrigation that would be lost. However, irrigated areas had to be derived by applying regional crop-specific irrigated percentages from earlier (2005 and 2010) irrigation surveys to crop maps (Q7), whilst the latter component of the methods were based on Morris et al. (Reference Morris, Weatherhead, Mills, Dunderdale, Hess, Gowing, Sanders and Knox1997) which may no longer adequately reflect more recent irrigation benefits. Given that the environmental benefits of such mandatory abstraction restrictions have yet to be determined, there is an urgent need for an evidence-based justification for restrictions on agriculture and horticulture (Q12).
Outdoor vegetable growers typically maintain a buffer or ‘headroom’ (Q15) between the expected dry-year irrigation need of their cropping plan assumed to be based on the 16th driest year in 20 (Weatherhead et al., Reference Weatherhead, Knox, Twite and Morris2002) (Q10) and their water resources allocation (licenced volume). This aims to reduce the likelihood that a volumetric shortage during a drought will reduce their output of marketable produce and/or lead to financial loss. Consequently, most irrigation abstraction licences are not fully used in most years, leading to a perception of an inefficient allocation of water. However, there are other legitimate agronomic and economic reasons for maintaining a reasonable level of licence ‘headroom’, even in dry years, which needs to be understood (Q11). Otherwise there is a risk that reducing licence volumes to reduce (apparent) headroom will lead to reduced crop production and/or increased risk of financial penalties to growers and increased costs through the food supply chain during drought.
Not all hydrological drought impacts on irrigated agriculture and horticulture arise from abstraction restrictions imposed through regulatory drought plans as required under the Water Industry Act (1991) or from Section 57 restrictions. The inability to meet crop irrigation needs in a given year can also arise from the irrigation need being greater than the ‘design’ dry-year need, HoF conditions stipulated within abstraction licence conditions, insufficient capacity in the irrigation system design (in pumping, conveyance and application) to meet peak irrigation need and insufficient reservoir storage. The relative significance of each of these potential constraints is uncertain (Q10).
Understanding drought responses
Increasing the resilience of agricultural systems to drought can be achieved by farmers reducing the probability of the drought event, increasing the robustness of the system to drought or facilitating recovery (Hess et al., Reference Hess, Knox, Holman and Sutcliffe2020). Probability can be reduced by lower-cost options such as improving the water holding capacity of soils (soil moisture drought), increasing organic matter content, tied ridging or by mulching or higher capital investments such as reservoir storage (hydrological drought). However, large scale investments can lock growers into their existing production system leading to limited scope and reduced flexibility to change planting programmes. Robustness is generally achieved through building diversity (having access to different water sources; growing a wider range of crops; being able to substitute grazing for conserved fodder (Salmoral et al., Reference Salmoral, Ababio and Holman2020) and/or redundancy (e.g., having under-utilised grazing: Salmoral et al. (Reference Salmoral, Ababio and Holman2020); spare irrigation equipment, licence headroom). However, there is a tendency to reduce diversity and redundancy, as (1) market pressures drive increased economic efficiency, economies of scale and reduced marginal costs of production (Abson et al., Reference Abson, Fraser and Benton2013); (2) regulatory pressures are moving towards reducing headroom and (3) both diversity and redundancy have opportunity costs (Abson et al., Reference Abson, Fraser and Benton2013) that, unless outweighed by the avoided penalties of infrequent droughts, lead to the ‘spare’ resources being utilised. This situation has been reported in South Africa where investments intended to build drought resilience have instead led to business expansion with resources underpinning increased irrigated production (Lankford et al., Reference Lankford, Pringle, McCosh, Shabalala, Hess and Knox2023). Consequently, the highly competitive financial environments facing most farmers has a tendency to lead to farm business decision-making strategies that increase the economic efficiency of production to the detriment of system redundancy, diversity and resilience to drought (Hess et al., Reference Hess, Knox, Holman and Sutcliffe2020) (Table 3: Q18). This leads to a trade-off between the high expected gross margins (a common indicator of the economic performance of farm enterprises) of specialised production systems and the expected variance of those margins as a consequence of variability in environmental, economic and policy perturbations (Abson et al., Reference Abson, Fraser and Benton2013). Consequently the current and future land use choices are, and will be, influenced by the risk to returns preferences of individual farmers (Di Falco and Chavas, Reference Di Falco and Chavas2006; Baumgärtner and Quaas, Reference Baumgärtner and Quaas2010) and their perceptions of drought risk.
Table 3. Key knowledge gaps regarding agricultural responses to drought

Although there is an unknown potential for short-term ‘no- or low regret reductions in irrigation abstraction during drought events (Q13), increasing resilience to drought carries costs, including capital investment costs (e.g., farm reservoir construction), operational costs (e.g., soil management) and/or profit-foregone (e.g., reduced cropped areas), so that there will not be financial benefits of change in all years. Consequently, different typologies of farms will have different constraints and risk appetite which need to be better understood (Q14) – farms with limited financial capacity to adapt may be more likely to adopt practices that save money in all years (Rial-Lovera et al., Reference Rial-Lovera, Davies and Cannon2017); whilst capital intensive production systems may select risk averse options that minimise regret (Knox et al., Reference Knox, Morris and Hess2010).
Increasing social capital has also been shown as a low-cost means of enhancing drought resilience (Holman et al., Reference Holman, Hess, Rey and Knox2021). As there are many farms, both large and small, dependent on supplemental irrigation, irrigators arguably need to have a more coordinated and coherent voice at the local level. This can be facilitated by the formation of water abstractor groups (WAGs) (Whaley and Weatherhead, Reference Whaley and Weatherhead2015a) that fosters trust between farmers and between farmers and the regulator (Whaley and Weatherhead, Reference Whaley and Weatherhead2015b), increased negotiating power (Leathes et al., Reference Leathes, Knox, Kay, Trawick and Rodriguez-Diaz2008) and increased adaptive capacity through improved social networks and knowledge exchange (Holman and Trawick, Reference Holman and Trawick2011). However, despite the acknowledged benefits of WAGS, there remain only a limited active number (Whaley and Weatherhead, Reference Whaley and Weatherhead2015c) mainly in Eastern England, although recent droughts in 2018 and 2022 have refocused attention on their potential role in building social capital and local resilience to droughts and water scarcity (Q16).
Collaboration also has the potential to deliver further benefits in irrigated agriculture through facilitating the collaborative management of licenced water resources (Whaley and Weatherhead, Reference Whaley and Weatherhead2015b) through water trading (Rey et al., Reference Rey, Pérez-Blanco, Escriva-Bou, Girard and Veldkamp2019), secondary water markets (Rey, Reference Rey2015) and water sharing (Haro-Monteagudo et al., Reference Haro-Monteagudo, Knox and Holman2019; Chengot et al., Reference Chengot, Knox and Holman2021) to support more efficient water allocation (Q17). Chengot et al. (Reference Chengot, Knox and Holman2021) showed that water sharing could potentially significantly reduce irrigation deficits during drought across a group of abstractors in an Eastern England catchment within the pre-existing abstraction licence and HoF constraints that prevent environmental degradation. However, there remains a lack of understanding around the potential operationalisation (Q17) and need for near real-time hydrological data to support practical implementation of such approaches (Rey et al., Reference Rey, Holman and Knox2021).
Understanding these drought responses across and within different sub-sectors remains challenging. A number of knowledge gaps, particularly in the context of promoting collaborative water management, still need to be addressed through future research efforts (Table 3).
Drought forecasting
The reactive responses of most U.K. farmers to drought (Rey et al., Reference Rey, Holman and Knox2017; Salmoral et al., Reference Salmoral, Ababio and Holman2020) is partly due to a lack of reliable information on the state and expected changes in environmental conditions. Drought MEW systems offer the promise of enabling appropriate and timely management actions (Hannaford et al., Reference Hannaford, Collins, Haines and Barker2019) (Table 4: Q19). They typically use drought severity indicators (WMO and GWP, 2016) to monitor the changing status of rainfall, river flows, groundwater levels, and other hydrometeorological variables, relative to historical conditions, rather than impact indicators (Bachmair et al., Reference Bachmair, Stahl, Collins, Hannaford, Acreman, Svoboda, Knutson, Smith, Wall, Fuchs and Crossman2016).
Table 4. Key knowledge gaps related to MEW systems for agriculture

Key questions relating to MEWS implementation in agriculture are given in Table 4 and the design criteria for a useful MEW for agriculture are summarised below:
-
• Choice of indicators: It is unclear whether standardised indicators (such as the SPI used in the UK Water ResourcesFootnote 1 portal), quantitative multivariate composite indicators as recommended by Hannaford et al. (Reference Hannaford, Collins, Haines and Barker2019) or impact indicators (Clarke et al., Reference Clarke, Hess, Haro-Monteagudo, Semenov and Knox2021) will have resonance and uptake within the diverse agricultural and horticultural industry (Q20). Clarke et al. (Reference Clarke, Hess, Haro-Monteagudo, Semenov and Knox2021) reported that the correlation between SPI and SPEI with simulated yield loss were similar, suggesting that SPI may be suitable for U.K. drought monitoring for wheat. However, evapotranspiration rates are expected to increase due to climate change, so that SPEI may be more appropriate for longer-term use (Haro-Monteagudo et al., Reference Haro-Monteagudo, Daccache and Knox2017). Reinforcing this, Parsons et al. (Reference Parsons, Rey, Tanguy and Holman2019) found that a generalised linear model using SPEI-6 gave better results against reported impact data. Standardised indicators cannot be interpreted reliably at a national scale in a country with as much regional variation in climate and agricultural practice as the UK. The same value of SPEI in different locations corresponds to very different soil moisture deficits and growing conditions (and thus soil moisture drought severity), but Parsons et al. (Reference Parsons, Rey, Tanguy and Holman2019) found that this did not fully explain observed regional differences in reported drought impacts. They reported that such standardised indicators would need to be calibrated on a regional (or smaller) scale to be used with confidence as an indicator of agricultural drought within a MEW system. Future research may also benefit from the increased availability of high resolution multispectral remote sensed imagery (e.g., Sentinel 2) coupled with spatial soil moisture sensing networks, both in situ and from the cosmic ray (COSMOS) monitoring network (https://cosmos.ceh.ac.uk/) to derive improved composite based drought indicators.
-
• Forecast lead times: A business-appropriate forecast lead time is needed to enable farmers to take pre-emptive action to minimise the financial impact of drought (Q21). However, these lead times are likely to be different in different agricultural production systems, for example, informing buying in additional livestock feed during the early stages of a drought compared to changing the crops or varieties selected for planting programmes.
-
• Forecast reliability: Given the reliability of forecasts with sufficient lead-time to inform decisions and the relative infrequency of drought risk, farmers are reluctant to make changes that they know will lead to reduced returns if a drought does not occur (Rial-Lovera et al., Reference Rial-Lovera, Davies and Cannon2017; Hess and Sutcliffe, Reference Hess and Sutcliffe2018) (Q21 and Q22). Over 76% of potato growers reported by Vicario et al. (Reference Vicario, Holman, Sutcliffe and Hess2023) had forward contracts for between 50 and 100% of their potato crops, with over 50% of contracts specifying financial penalties if the grower were unable to fully deliver due to a water-related issue.
Understanding future trends
Following a long period of increasing abstraction, Weatherhead et al. (Reference Weatherhead, Knox, Hess and Daccache2015) reported that the volume of water abstracted for irrigation was declining by −1.4%/year between 1990 and 2010 after allowing for annual weather variability, partly reflecting increased yields and hence decreasing crop areas, increased efficiency, better irrigation scheduling and reduced water availability and reliability. However, it is questionable to project such past national (and regional) trends forward due to potential future changes in agroeconomic policy, market needs, shocks (Table 5: Q25), agroclimate variability and change, and resources availability (Knox et al., Reference Knox, Haro-Monteagudo and Morris2018). Agricultural water demand forecasting is essential to informing future policy and strategies (e.g., Knox et al., Reference Knox, Daccache, Weatherhead, Groves and Hulin2013; Weatherhead et al., Reference Weatherhead, Knox, Hess and Daccache2015). There have been numerous published studies of the impacts of climate change on irrigated agriculture including the implications for (unconstrained) irrigation needs of currently irrigated crops (e.g., Weatherhead and Knox, Reference Weatherhead and Knox1999; Daccache et al., Reference Daccache, Weatherhead, Stalham and Knox2011) and potential changes in the spatial distribution of irrigation demand due to changing land suitability (e.g., Daccache et al., Reference Daccache, Keay, Jones, Weatherhead, Stalham and Knox2012). However, there are multiple barriers (Knox et al., Reference Knox, Morris and Hess2010) to transformational changes in farming and food systems, such as movements in key commodity production areas in the UK, in response to more extreme conditions (Folke et al., Reference Folke, Carpenter, Walker, Scheffer, Chapin and Rockstrom2010; Rickards and Howden, Reference Rickards and Howden2012).
Table 5. Key knowledge gaps related to future trends

Supermarkets have increasingly dominated the U.K. grocery market so that their focus on product specification (aesthetics, size and quality standards) has driven supplemental irrigation practice and investment (Knox and Hess, Reference Knox, Hess and Wale2018; Sutcliffe et al., Reference Sutcliffe, Knox and Hess2021; Vicario et al., Reference Vicario, Holman, Sutcliffe and Hess2023). Widespread use of irrigation depends on the profitability of irrigation, which in turn depends considerably on the price differentials offered for quality produce in the market. The combination of the challenges of meeting product specifications within forward contracts during drought years (Vicario et al., Reference Vicario, Holman, Sutcliffe and Hess2023); price competition with discounting supermarkets and the reducing profitability of fresh produce coupled with major water regulatory reforms may lead to some major changes in market preference (pre-pack vs. processing) and irrigation practice (Q18).
There is much less understanding of the increased future water requirements for currently rainfed crops and livestock systems (Q23 and Q24). The main adaptations to drought within rainfed cropping include earlier planting, selection of more drought tolerant crops and varieties cultivars, changed cultivation practices, and increasing soil organic matter (Rial-Lovera et al., Reference Rial-Lovera, Davies and Cannon2017). As pro-active measures, they cannot reduce impacts once a drought has started, unlike supplemental irrigation. Irrigation of wheat in the UK has traditionally been considered uneconomic, but increases in world wheat prices, increased drought frequency and the impacts of climate change are likely to make the financial benefits stronger (El Chami et al., Reference El Chami, Knox, Daccache and Weatherhead2015) (Q24). Similarly, given observed drought impacts on maize yields in 2018 and the rapid expansion of anaerobic digestion plants, supplemental irrigation of maize may become more widespread (Knox and Hess, Reference Knox and Hess2019) particularly in regions where water resources are constrained and smaller irrigation applications deliver alternative sources of income.
Irrigation is currently not commonly used in livestock systems in the UK. Future changes in drought and/or climate that reduce growing season rainfall will reduce the quantity and quality of grass forage produced and may (where abstraction water is available) increase the adoption of supplemental irrigation in intensive grassland systems (Wreford and Topp, Reference Wreford and Topp2020) (Q23) to reduce the need for supplementary feeding (Roy et al., Reference Roy, Hough and Starr1978).
However, for most currently rainfed crops and on most soils, supplementary irrigation will not be needed in most years. Consequently, there is a need to better understand the regions and conditions in which rainfed farms would invest in supplementary irrigation (leading to increased demand for irrigation abstraction) (Q23), the situations within which irrigated farms would increase irrigation of the currently rainfed crops in their rotations (leading to increased usage of their volumetric limits) (Q24) and the potential for socio-economic change and shocks to change the spatial patterns of production and volumetric needs for irrigation (Q25).
Food system vulnerability to international drought and water scarcity
The UK relies extensively on fresh fruit and vegetable imports mainly from arid and semi-arid countries where there is already severe pressure on freshwater resources but where increasing drought risks pose a major threat to our food system. Three quarters (76%) of the freshwater consumed in the supply of fresh fruit and vegetables to the UK is withdrawn overseas, with our supply chain being particularly vulnerable to climate (drought) and water-related risks in seven ‘hotspot’ countries (Hess and Sutcliffe, Reference Hess and Sutcliffe2018). Collectively, these account for >90% of the blue water scarcity footprint associated with the import of fresh fruit and vegetables, including Spain (46%), Egypt (15%), South Africa (11%), Chile (6%), Morocco (6%), Israel (5%) and Peru (2%) (Hess and Sutcliffe, Reference Hess and Sutcliffe2018). For all these countries, drought is a major agricultural production risk, and with a changing climate with increased aridity this only exacerbate the current situation.
Whilst significant attention has been given to addressing the on-farm impacts of drought including adaptation options and responses, there remains a gap in understanding how different components of export orientated food supply chains are impacted by drought including their options to build resilience not only within the specific components (packing, processing, distribution) but also more generally to the food system per se. Recent research by Rey Vicario et al. (2022) focusing on potato supply chain showed it has increased its resilience to such weather extremes by retailers and packers having a wider geographical spread of supply, increasing their reliance on forward contracts, and favouring farm businesses that have water security (e.g., reservoirs). However, their analysis also showed that these resilience-building strategies were measures that were largely intended to reduce their own business drought and water scarcity risks rather than considering the consequences within other parts of the fresh produce supply chain. A more integrated approach to drought resilience that facilitates both horizontal and vertical collaboration and importantly trust between the different system components was recommended (Vicario et al., Reference Vicario, Holman, Sutcliffe and Hess2023).
Conclusions
This review has shown that the sensitivity of farming to drought differs between agricultural and horticultural sub-sectors, due to their reliance on different sources of water to sustain production. Consequently, agricultural and horticultural sub-sectors are variably affected by meteorological, soil moisture, hydrological and socio-economic drought – hence the term ‘agricultural drought’ when referring to high soil moisture deficits grossly over-simplifies the drought challenges facing the sector. Drought impacts manifest themselves in many different ways over a range of timescales and geographies, including through changes in productivity, quality, livestock welfare, business profitability and farmer wellbeing. Due to the resulting spatial complexity and major economic damages, there is an urgency to improve the granular understanding of drought impacts and the barriers and enablers of both reactive and pro-active drought responses. This includes understanding the minimum performance requirements for drought MEW systems, including the lead times and reliability needed to give business decision confidence in different agricultural sub-sectors. However, drought risk management responses need to increasingly recognise that drought interacts within an agricultural industry that is constantly evolving in response to a complex mix of agronomic, market, regulatory and financial externalities.
Open peer review
To view the open peer review materials for this article, please visit http://doi.org/10.1017/wat.2023.7.
Supplementary material
The supplementary material for this article can be found at https://doi.org/10.1017/wat.2023.7.
Data availability statement
No new data were created or analysed in this study. Data sharing is not applicable to this article.
Acknowledgements
We acknowledge the support of Stuart Neil, Andy Beverton and Glenn Watts at the Environment Agency.
Author contribution
I.P.H.: Funding acquisition, project administration, conceptualization; writing – original draft. J.W.K.: Writing – review and editing.
Financial support
This work was supported by the Environment Agency (Grant No. SC220025).
Competing interest
The authors declare no competing interest exists.
Ethics standard
The activity was approved by the Cranfield University Research Ethics System (CURES) – reference CURES/17922/2023.
Comments
Invited to submit review paper by Water Prism journal Editors