Introduction
Animal migration, the more or less regular movement of animals to or from a particular geographic area, is a widespread phenomenon and is often associated with seasonality (Dingle Reference Dingle1996). Migration requires adaptations in physiology, behaviour and morphology, and individuals with optimal migration traits should be at a selective advantage. In flying animals which migrate, one obvious trait that should be subject to selection is wing shape. Comparative studies have found indirect evidence that wing shape is indeed under selection since many migrating populations and species have longer and/or more slender wings than non-migrating ones (Calmaestra and Moreno Reference Calmaestra and Moreno2001, Johansson et al. Reference Johansson, Söderquist and Bokma2009, Baldwin et al. Reference Baldwin, Winkler, Organ and Helm2010, Suarez-Tovar & Sarmiento Reference Suarez-Tovar and Sarmiento2016, Freedman and Dingle Reference Freedman and Dingle2018). In addition, comparative studies on migrating insects have also found that long-distance migrating populations and species have a larger wing area (Altizer & Davis Reference Altizer and Davis2010, Suarez-Tovar & Sarmiento Reference Suarez-Tovar and Sarmiento2016, Li et al. Reference Li, Pierce and de Roode2016). An alternative indirect approach to study such selection would be to compare wing shape and wing area in a population before and after completing migration (Yang et al. Reference Yang, Ostrovsky, Rogers and Welker2016, Flockhart et al. Reference Flockhart, Fitz-Gerald and Brower2017). We use this approach here with a migratory dragonfly as our study organism.
One of the best-known examples of migration is the seasonal migration of birds from wintering grounds in the tropics to temperate breeding grounds. However, even within the tropics migration is common. For example, many insect species, including dragonflies, migrate within the tropics in response to variation in rainfall and resource availability (Suhling et al. Reference Suhling, Martens and Marais2009, Drake and Reynolds Reference Drake and Reynolds2012). Dragonfly migration is known to occur in about 25–50 of the 5200 or so species in the world (Russell et al. Reference Russell, May, Soltesz and Fitzpatrick1998, Corbet Reference Corbet1999, May Reference May2013, Schilling et al. Reference Schilling and Kardynal2020, Borisov et al. Reference Borisov, Lakovlev, Borisov, Zuev and Tiunov2020a). One of the most familiar examples is that of the Globe Skimmer or Wandering Glider Pantala flavescens (Fabricius, 1798). This species is the most widely distributed of all dragonflies, with a circumtropical distribution, although it does also occur in many temperate areas (Corbet Reference Corbet1999, Günther Reference Günther2019). It has been suggested that P. flavescens migrates from India to East Africa in a single generation (Figure 1), covering a total distance of about 4500 km, probably longer, of which about 3500 km at a stretch occurs over open ocean (Anderson Reference Anderson2009, Hobson et al. Reference Hobson, Anderson, Soto and Wassenaar2012, Borisov et al. Reference Borisov, Lakovlev, Borisov, Ganin and Tiunov2020b). A recent energy flight model study did indeed show that such a long flight distance is theoretically possible in P. flavescens if gliding and wind direction selection is taking into consideration (Hedlund et al. Reference Hedlund, Lv, Lehmann, Hu, Anderson and Chapman2021). The dragonflies probably leave the west coast of India in September-December, and some migrate through the Maldive Islands where numbers peak in November. Such a long migration presumably necessitates selection of wing characteristics that are optimal for long-distance flight.
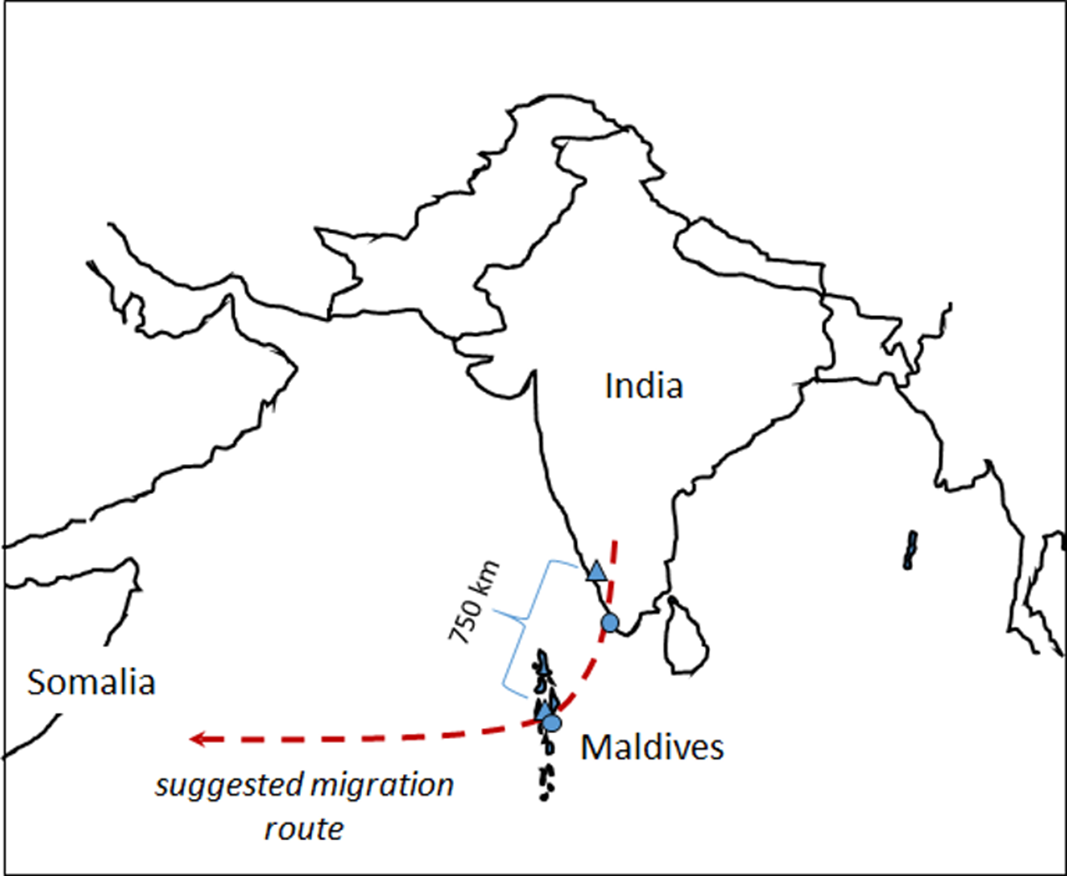
Figure 1. Map showing the suggested migration route, sampling sites of Pantala flavescens and the distance between one of the sampling sites on the mainland and the Maldives. Triangles and dots denote sampling sites in 2010 and 2018, respectively. Modified after Hobson et al. Reference Hobson, Anderson, Soto and Wassenaar2012.
Comparing wing shape and wing area of individuals at two positions along a migration route should provide indirect evidence for selection of wing shape, if it is indeed taking place. Since migration is energetically expensive (Bowling & Wikelski Reference Bowlin and Wikelski2008), individuals with an optimal wing shape and larger wing area will have a higher chance of survival during the migration, and successful migrants should thus on an average have different wing characteristics compared to the average before migration.
Recent comparative studies of wing shape in migrating dragonflies have found that migrants tend to have basally wider wings and a more pointed apex than non-migrants (Johansson et al. Reference Johansson, Söderquist and Bokma2009, Suarez-Tovar & Sarmiento Reference Suarez-Tovar and Sarmiento2016, Outomuro & Johansson Reference Outomuro and Johansson2019). These empirical results agree with predictions from aerodynamic modelling, that is theoretical explanation at the functional level (Wootton & Newman Reference Wootton, Newman and Córdoba-Aguilar2008; Bomphrey Reference Bomphrey2016). Among other things, such differences should favour gliding, which might save energy during migration. Dragonflies have two pairs of wings, and the consensus from comparative studies suggests that the shape of the hind wings differed far more than that of fore wings between migrating and non-migrating species (Johansson et al. Reference Johansson, Söderquist and Bokma2009, Suarez-Tovar & Sarmiento Reference Suarez-Tovar and Sarmiento2016). In addition, it has been suggested that the basal lobe of the hind wing found in many migrating species is associated with gliding, which might be adaptive in migrating species (Corbet Reference Corbet1962, Johansson et al Reference Johansson, Söderquist and Bokma2009). Thus, the fact that migratory dragonfly species have hind wings with wider bases and the fact that hind wings and front wings differ much more in shape in migratory species than in non-migratory species together suggest that gliding might be important for efficient migration in dragonflies. However, both wings are used in active flight (Wootton & Newman Reference Wootton, Newman and Córdoba-Aguilar2008).
While the majority of studies on migrating dragonflies have compared different species (see references above), the massive annual migration of P. flavescens from mainland India to the Maldives provides a unique opportunity to compare wing shape before and during migration within a single species. We investigated this possibility by collecting individuals in Kerala, near the southern end of Peninsular India, and in the Maldives. Based on previous empirical results from other dragonfly species and results from aerodynamic modelling, we predicted that on average the wings of P. flavescens dragonflies collected in the Maldives should be more pointed, but with a wider base and a larger wing area, compared to those collected in Kerala. We further predicted that the wing shapes seen in Maldivian specimens should be a subset of those collected in Kerala. Empirical confirmation of our predictions on wing shape and area would provide indirect evidence for selection during migration, but we are aware of the limitations of this approach (Gould and Lewontin, Reference Gould and Lewontin1979) and more information would be needed to confirm the pattern, as we discuss below.
Material and methods
Specimen collection
Kerala: Adult P. flavescens were caught with an entomological net in October 2010 close to the coast at Kuriachira, Thrissur, Kerala, India (10.508964, 76.219694) and in October 2018 at Vellayani, Trivandrum, Kerala, India (8.430082, 76.985632) (Figure 1). The left front and hind wings were removed with a pair of forceps and scanned together with a 1 cm reference scale on a flatbed scanner. All specimen handling was carried out in India.
Maldives: In the same years, but 1-2 weeks later, adults of P. flavescens were caught with a butterfly net on the Maldives (4.503356, 73.556317 and 4.208593, 73.541659 in 2010 and 2018, respectively) (Figure 1). There are no freshwater lakes or streams on the Maldives, suggesting that these individuals were migrants from mainland India: see Anderson (Reference Anderson2009), Hobson et al. (Reference Hobson, Anderson, Soto and Wassenaar2012) and Borisov et al. (Reference Borisov, Lakovlev, Borisov, Ganin and Tiunov2020b) for more details on this migration. Specimens were sent by mail to the Department of Ecology and Genetics at Uppsala University where the wings were processed and scanned in the same way as those from India. Body length was measured from the tip of the head to the end of the abdomen (excluding appendages). Body length of the specimens from Kerala was not measured.
Wing shape data
Wing shape was analysed with geometric morphometrics (Rohlf & Marcus Reference Rohlf and Marcus1993). Nine and thirteen landmarks were used to capture the shape of the front and hind wings, respectively (electronic supplementary material, Fig. S1). Since it was difficult to find prominent landmarks on the expanded lobe of the hind wings close to the body, we used semi-landmarks (landmarks 9–12) that were permitted to slide along their tangent direction until minimizing the Procrustes distance between specimens (Bookstein Reference Bookstein1991). The digitization of the landmarks was carried out using the software tpsDig version 2.32 (Rohlf Reference Rohlf2018).
Using tpsRelw (Rohlf Reference Rohlf2019), landmarks were subjected to a generalized Procrustes analysis (Rohlf & Slice Reference Rohlf and Slice1990) and the analyses were performed separately for fore and hind wings, sex and year. We saved the shape variables, uniform and non-uniform shape components from this analysis (Rohlf & Marcus Reference Rohlf and Marcus1993), for subsequent statistical comparisons of wing shape between specimens from Kerala and the Maldives.
To visualize how wing shape differed between mainland Kerala and the Maldives, we first computed the average wing shape of specimens from Kerala and the Maldives. We then generated thin-plate spline deformation grids for each of these (relative to the overall reference shape) using tpsSplin version 1.22 (Rohlf Reference Rohlf2016).
As a measure of specimen size, we measured wing length. Wing length was estimated from the scanned images of wings using ImageJ, version 1.52p (Rasband Reference Rasband1997). On both the front and the hind wings, length was estimated as the distance between landmark 1 and 3 (Fig. S1).
Since we assume that P. flavescens migrates from Kerala and that individuals with wings that are more pointed and with wider base are more likely to be successful migrants to the Maldives, we expected the Maldives samples to be a subset of the Indian samples. Hence, the Indian samples should be more variable and should include the variants that occur in the Maldives. To explore this possibility, we performed a principal component analysis (PCA) on the shape variables and saved the score from the first three principal components for visualization of the wing shape variation between samples from Kerala and the Maldives. This approach of using PCA scores to compare shapes is similar to relative warps analysis in geometric morphometric terminology and is used regularly when comparing shapes (Rohlf Reference Rohlf1993). We used only the first three PC axes since the rest of the axes explained only a low percentage of the variation. The first three axis explained 60.2 % and 55.6% of the variation for front and hind wings, respectively, see supplementary material for more information about the variance explained for each PCA. The analyses and visualization of wing shape variation between Kerala and Maldives were restricted to female samples from 2018, because only for this data set did we have enough replicates (>30) for meaningful comparisons. Since sample size differed between Kerala and the Maldives, we adjusted replicate numbers to be equal using a random subsample of wings.
Wing area
Wing area was estimated from the same data set as for wing shape, but the number of individuals differs slightly since some damaged wings could not be used for wing marks and vice versa. We used ImageJ, version 1.52p (Rasband Reference Rasband1997) for estimating wing area.
Statistical analysis
Wing shape difference between samples from Kerala and Maldives was analysed with MANCOVAs, using the shape components as response variables, location as a factor and wing length as a covariate. Unfortunately, we did not have body size measurements of individuals from Kerala, so we used wing length measurements as our covariate. For the Maldives data set, there was a positive relationship between wing size and body size (linear regression, p < 0.05). Body length or body mass and wing size of dragonflies are usually correlated (May Reference May1981, Koenig & Albano Reference Koenig and Albano1987, Johansson et al. Reference Johansson, Söderquist and Bokma2009). If the covariate in the MANCOVAs was non-significant (p> 0.15), we removed it from the final shape analysis comparison. We analysed the years, sex and front/hind wings separately for several reasons. First and most important, the program tpsRelw calculates a consensus wing against which all wings are compared, and hence including year, sex and front/hind wing as separate factors would visualize differences between Kerala and the Maldives that is confounded by these factors. Second, our main interest was to compare wing shape between samples from Kerala and the migrants on the Maldives rather than differences between years. Wing shape might differ between years because of condition-dependent growth. Moreover, wing shape differs between males and females in P. flavescens (unpublished F. Johansson) as in many other dragonfly species, for example Outomuro et al. (Reference Outomuro, Dijkstra and Johansson2013), and therefore, we also excluded sex as a separate factor in the analysis.
For the 2010 hind wing data set, there were insufficient degrees of freedom for a powerful MANCOVA (this data set had fewer individuals, see Supplementary Table 1, and in addition, hind wings had more landmarks). Therefore, for this data set, to reduce the number of variables analysed, we ran a PCA on the uniform and non-uniform shape components (which were calculated exactly as above) and saved the scores. This gave us 21 and 23 PC axis for the samples from Maldives and Kerala, respectively, from where we saved the scores for subsequent MANCOVA analyses (a similar MANCOVA as above, but here we used the score from the PCA instead). Since scores from 21 and 23 PC axes were too many for this MANCOVA, we limited our analysis to scores from 18 of these PC axes, which explained 97.3% and 99.9% of the variation for male and female hind wings, respectively. This approach of using scores is similar to the relative warps analysis in geometric morphometric terminology and is used regularly when comparing shapes between factors (Rohlf Reference Rohlf1993). Thus for male and female hind wings for the 2010 data, we used the scores from the PCA and ran a MANCOVA. The statistical analyses were carried out using the software R version 3.5.2 (R Core team 2018), except for the analyses on the hind wing in 2010 where SYSTAT 11 (Systat 2004) was used. The number of wings used from each locality and sex can be found in Supplementary Table 1.
Wing area was analysed with F-test in SYSTAT (2004). Wing length was not used as a covariate in this analysis since it was correlated with wing area (r2 ≥ 0.94 for all comparisons). We made the same comparisons as for the wing shape analysis since this allowed us to compare the results between shape and area quantitatively. We also analysed wing length independent of wing shape and wing area. For this, we also used F-tests with locality as the factor.
Results
2010 samples
Front wing shapes differed significantly between samples from Kerala and the Maldives (Table 1, Figure 2). Wings from Maldivian individuals had a wider wing base, a wider wing apex and the tip of the wing pointed posteriorly. In addition, the middle part of the wing was more slender in individuals from the Maldives. This pattern of differences in wing shape was similar for males and females, as determined by visual comparison.
Table 1. Results of MANCOVAs examining shape variation between individuals from Kerala and the Maldives (Location) in 2010 for front and hind wings in males and females. Wing length was used as a covariate.

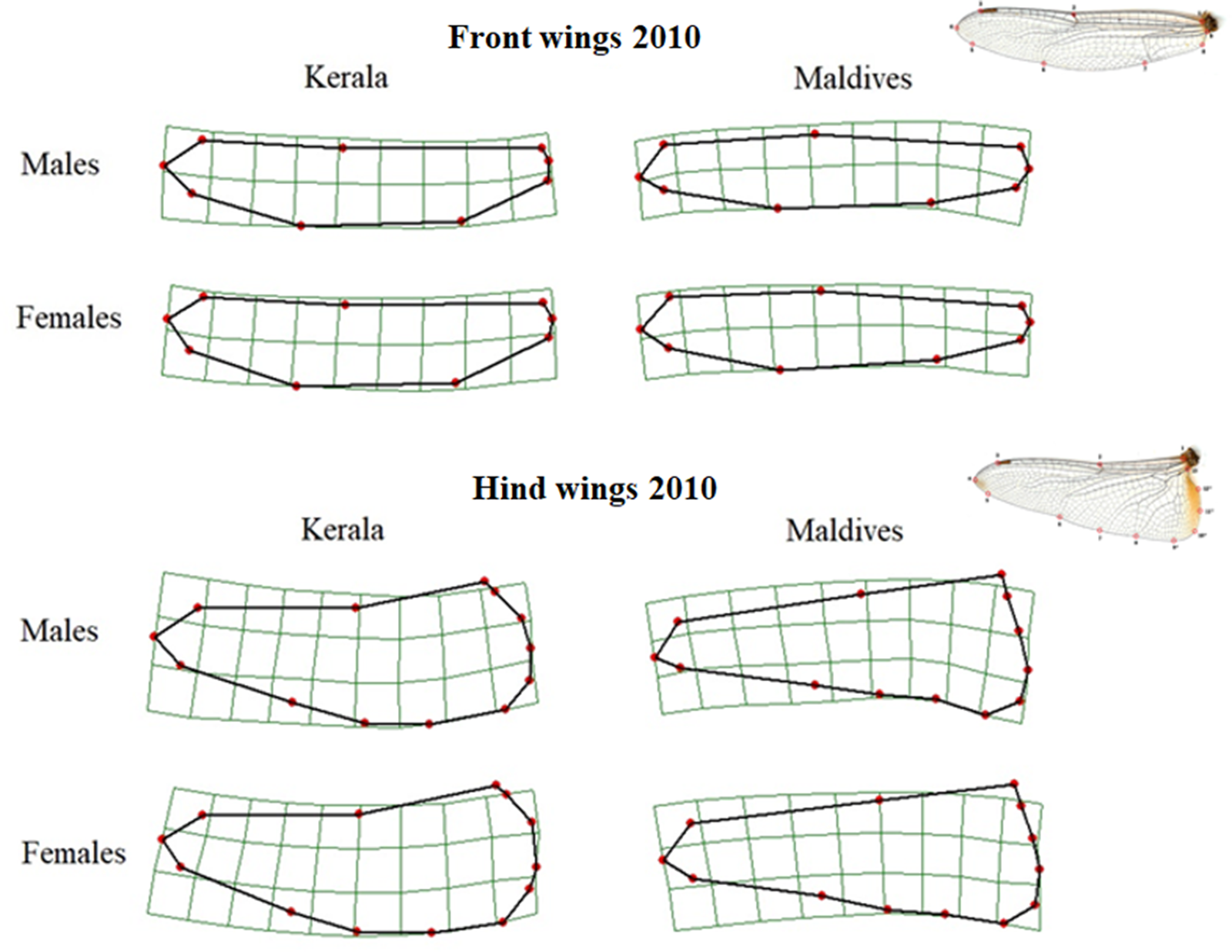
Figure 2. Thin-plate spline transformations showing consensus shapes of left front and hind wings of samples from Kerala and the Maldives in 2010. The deformation grids are scaled up with a factor of 5 to exaggerate the differences in shape, and the wing tip is to the left. The wing images at the right show wings and their landmarks.
Though front and hind wing shapes are different, the pattern of difference in the shape of hind wings between those caught in Kerala and the Maldives was similar to that of the front wings (Figure 2). Thus, the hind wing was wider at the base, and the tip of the wing pointed more posteriorly in individuals from the Maldives compared to those from Kerala. While this difference was significant for males, the p-values for female wing shape were marginally outside the level of significance (Table 1).
2018 samples
As with the 2010 samples, for those collected in 2018 the shape of the front wings differed significantly between samples from Kerala and the Maldives (Table 2, Figure 3). Front wings from Maldivian individuals had a wider base, a more pointed wing tip while the middle area of the wing was narrower. This pattern of wing shape difference was similar for both males and females.
Table 2. Results of MANCOVAs examining shape variation between Kerala and the Maldives (Location) in 2018 for front and hind wings in males and females. Wing length was used as a covariate.
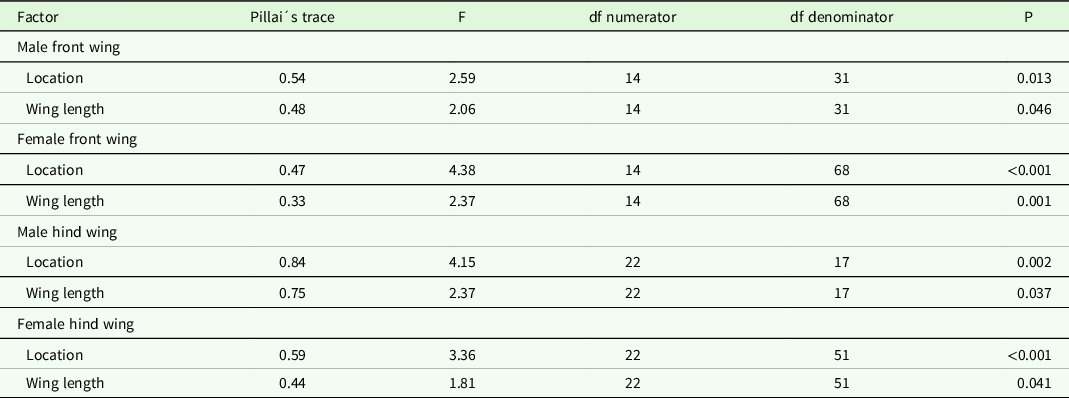
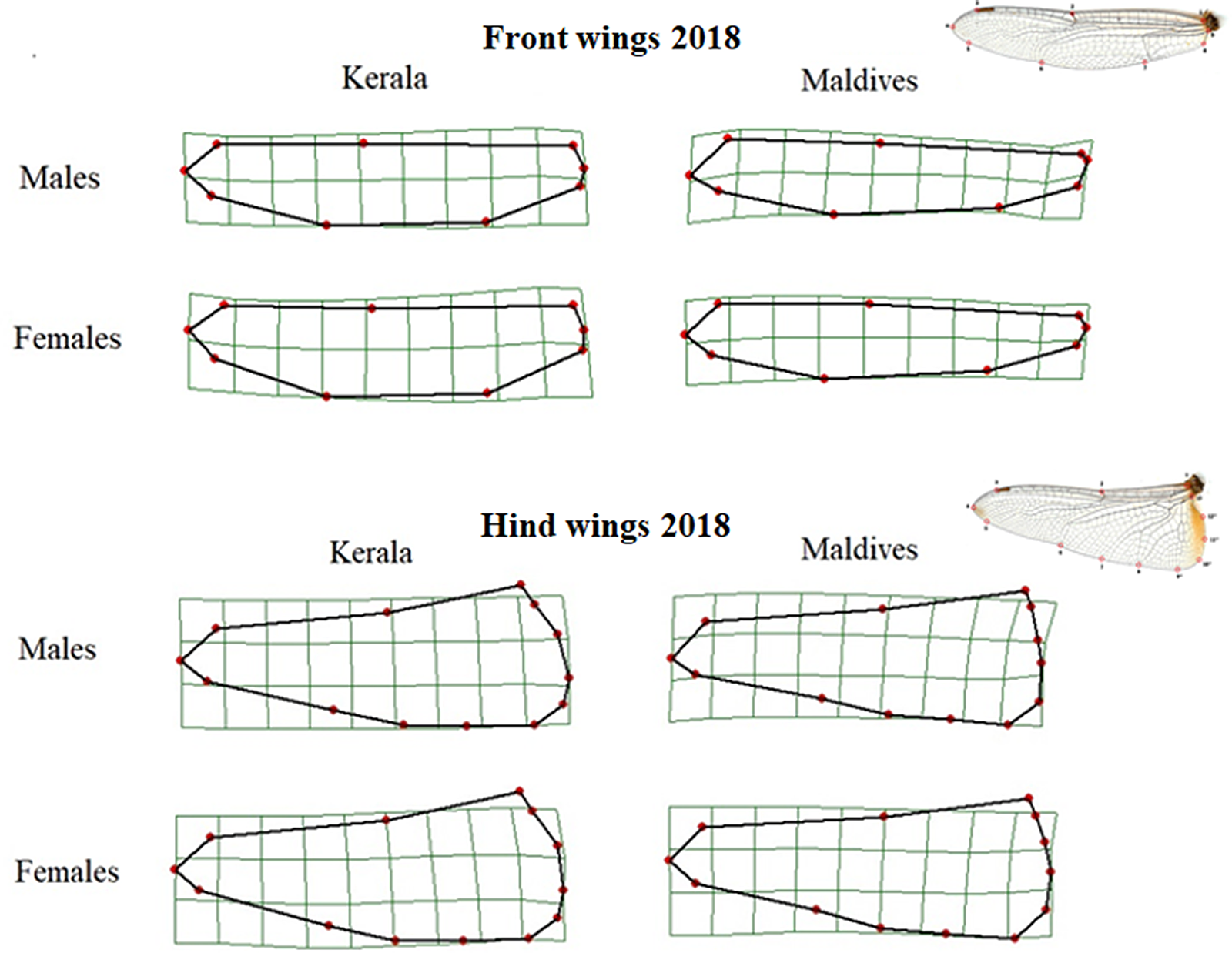
Figure 3. Thin-plate spline transformations showing consensus shapes of left front and hind wings of samples from Kerala and the Maldives in 2018. The deformation grids are scaled up with a factor of 5 to exaggerate the differences in shape, and the wing tip is to the left. The wing images at the right show wings and their landmarks.
The hind wings of Maldivian individuals were slightly wider at the base than those from Kerala. The wing tip pointed more posteriorly in individuals from the Maldives compared to those from Kerala (Figure 3), and this difference was significant for both males and females (Table 2).
2018 females (wing shape variation)
Wing shape variation between samples from Kerala and Maldives females collected in 2018 (i.e. our largest subsample) can be seen in electronic supplementary material Fig. S2. Visual comparison clearly showed that there was no support for our expectation that the Maldives sample was a subset of the Indian sample. The overall variation of the shape scores was similar between samples from the Maldives and Kerala.
Wing area and length
In 2010, wing area of both front and hind wings in males and females did not differ significantly between individuals collected at the Maldives compared to those collected at Kerala in any of the years (Tables 3 and 4). Wing length for both sexes and both wings was significantly larger in the year 2010 for individuals collected at the Maldives (Tables 3 and 4, p < 0.03). In the year 2018, only male hind wings were significantly longer for individuals collected at the Maldives (Table 3, P = 0.01). The other three comparisons for 2018 were non-significant (Table 3, p ≥ 0.08).
Table 3. Mean length and area of wings from Kerala and the Maldives by year, sex and front/hind wings. Values within parenthesis are standard deviations. n denotes number of individual analysed for Kerala and Maldives, respectively.
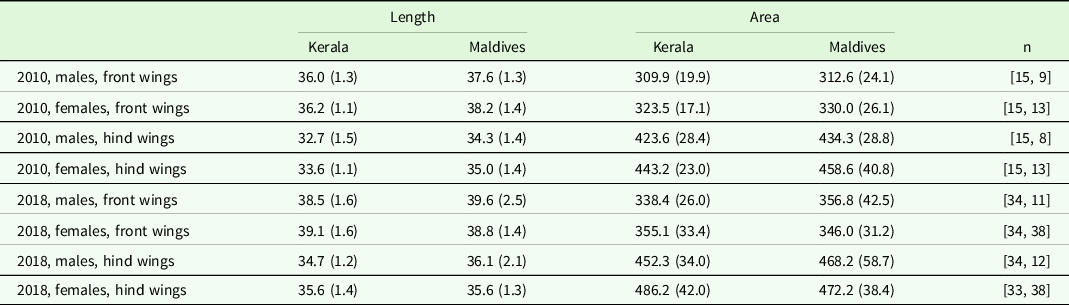
Table 4. Results of F-tests examining wing area variation between individuals from Kerala and the Maldives (Location) in 2010 and 2018 for front and hind wings in males and females.
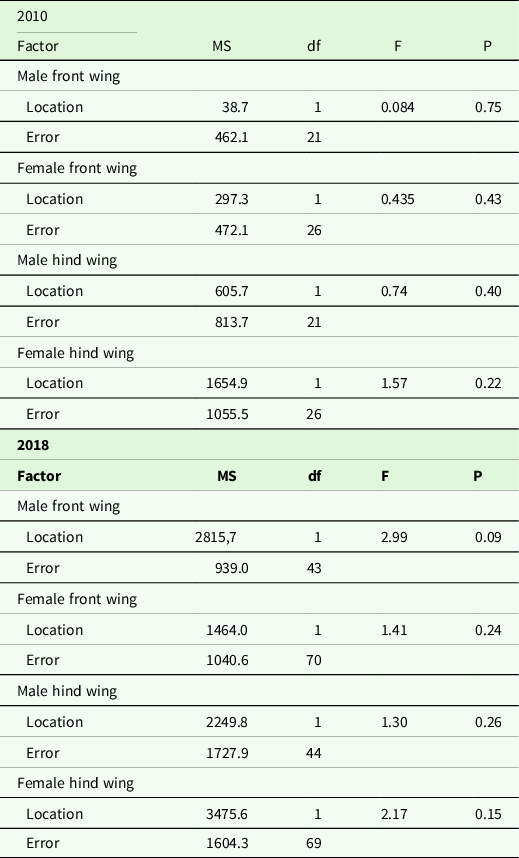
Discussion
Wing shape of migrant individuals from the Maldives differed from those from Kerala. Individuals from the Maldives tended to have a wider wing base, a narrower middle and a somewhat more pointed outer tip compared to those from Kerala. This pattern of wing shape differences was seen in samples from both 2010 and 2018.
These wing shape differences correspond qualitatively to the differences found between migrating and non-migrating dragonfly species. Migratory dragonfly species tend to have a larger anal lobe, with otherwise more pointed and narrower wings than non-migratory species (Johansson et al. Reference Johansson, Söderquist and Bokma2009, Suarez-Tovar & Sarmiento Reference Suarez-Tovar and Sarmiento2016). Similar wing shape differences are also found between migrating and non-migrating populations within species of birds and butterflies (Altizer & Davis Reference Altizer and Davis2010, Baldwin et al. Reference Baldwin, Winkler, Organ and Helm2010). These differences are mirrored in our study, where the Maldives specimens (i.e. the individuals which had presumably migrated the furthest) had wings wider at the base and narrower in the middle. In addition, theoretical predictions at the functional level also suggest that the wing shape observed in the migrants found at the Maldives should be optimal for long-distance flying (Wootton & Newman Reference Wootton, Newman and Córdoba-Aguilar2008).
We suggest that the differences in wing shape observed between the specimens collected in the Maldives and Kerala are due to differential mortality during migration. Individuals with a sub-optimal wing shape do not survive the long, over-water migration to the Maldives: only those with a wing shape that is beneficial for long-distance migration successfully complete the crossing. This explanation hinges on the assumption that the specimens collected in Kerala and the Maldives are from the same population. Stable isotope analysis suggests that P. flavescens found at the Maldives originate from north eastern India and fly via Kerala (Hobson et al. Reference Hobson, Anderson, Soto and Wassenaar2012). We suggest that the individuals found in both Kerala and the Maldives do indeed originate from north of Kerala and that those arriving in Maldives have departed from the coast of Kerala. Two lines of evidence support these suggestions. Firstly, individuals caught in Kerala were not newly emerged (teneral) individuals: their wings were not pristine, with several individuals showing wing damage, suggesting that they had emerged sometime earlier. In addition, we find it unlikely that P. flavescens take off from the coast in northern India and migrate over the seas all the way to the Maldives. A more likely scenario, and one supported by observations (e.g. Fraser Reference Fraser1924, Reference Fraser1936, Larsen Reference Larsen1987, Anderson Reference Anderson2009), is that they follow the mainland (where they can forage) until the southern end of the Indian peninsula and then take off. Second, the wing shape differences found were in the direction predicted, that is the wings of P. flavescens caught on the Maldives had a shape that is suggested to decrease energy use during migration (Johansson et al. Reference Johansson, Söderquist and Bokma2009, Wootton & Newman Reference Wootton, Newman and Córdoba-Aguilar2008, Suarez-Tovar and Sarmiento Reference Suarez-Tovar and Sarmiento2016, Bomphrey, Reference Bomphrey2016). The chances of finding this wing shape pattern by pure chance compared to any other difference if wing shape is probably low. It seems more likely the wing shape differences should be in a different direction, not a reflection of the optimal wing shape for migration, if it was not caused by selection during migration. Still, the wing shape of the successful migrants mirrors those found when comparing migrating and non-migrating dragonfly species (Johansson et al. Reference Johansson, Söderquist and Bokma2009, Suarez-Tovar and Sarmiento Reference Suarez-Tovar and Sarmiento2016). Nevertheless, whether the individuals found at the Maldives take off from the coast of Kerala still needs to be confirmed.
We did not find support for our expectation that the wing shape of the Maldives samples should be a subset of the Indian samples. We suggest two possible explanations for this result. First, it might be that our sample sizes were too small. Including more individuals should certainly capture more wing shape variability. Second, it might be that individuals reaching the Maldives did not originate from the coast of Kerala, and hence, our samples represent two different migration pathways. If this were the case, the differences observed may not necessarily be the result of selection for migration.
Pantala flavescens has been characterized as a global species, which migrates over enormous distances and has little genetic population structure across huge areas (Anderson Reference Anderson2009, Hobson et al. Reference Hobson, Anderson, Soto and Wassenaar2012, Troast et al. Reference Troast, Suhling, Jinguji, Sahlén and Ware2016, Alvial et al. Reference Alvial, Vargas, Marinov, Esquivel, Araya, Araya-Donoso, Vila and Véliz2019, Cao & Wu Reference Cao and Wu2019). It is an animal that in its adult phase is constantly on the move (Corbet Reference Corbet1999, Anderson Reference Anderson2009), which raises the question why might there still be scope for selection on wing shape during migration? The answer may be that during some periods, individuals might spend little time on migration or if they are migrating do so entirely over land with no long-distance flights over water (e.g. Ishizawa, Reference Ishizawa2007). During such periods, selection for other wing shapes might occur, thereby maintaining variation in wing shape. For example, in the damselfly Lestes sponsa, selection for survival favoured a different wing shape than did sexual selection (Outomuro et al. Reference Outomuro, Söderquist, Cortázar-Chinarro, Lundgren, Nilsson-Örtman and Johansson2016). Similarly, predation by birds was selected for shorter and broader hind wings in the damselfly Calopteyx splendens (Outomuro & Johansson Reference Outomuro and Johansson2015). Thus, sexual selection and predation may play a role in determining wing shape in P. flavescens during some periods. In our case, the long over-water flight to the Maldives may be of particular significance. Those P. flavescens arriving in Kerala in October-November must have already flown many hundreds of kilometres over land from northern India or beyond (Hobson et al. Reference Hobson, Anderson, Soto and Wassenaar2012, Borisov et al. Reference Borisov, Lakovlev, Borisov, Ganin and Tiunov2020b), during which time they would have had the possibility to roost at night, to mate, to hunt and to be subject to predation by terrestrial birds. Such ecological factors are expected to select for a different wing shape compared to a strong selection for over-water migration in those arriving in the Maldives in October-November, for which there would have been no opportunity to rest for several hundred kilometres.
Our analyses did not demonstrate a larger wing area in individuals caught at the Maldives, but we did find that wing length was longer for the Maldives individuals in 2010. We have no explanation for why our prediction of a larger wing area in migrants was not supported. Previous within-species studies have shown that a larger wing area is correlated with migration status in Monarch butterflies Danaus plexippus (Altizer & Davis Reference Altizer and Davis2010, Li et al. Reference Li, Pierce and de Roode2016), while a between-species comparison showed that migrating dragonflies have a larger wing area compared to non-migrating ones (Suarez-Tovar & Sarmiento Reference Suarez-Tovar and Sarmiento2016). The absence of an effect on wing area in our study suggests that wing shape may be more important for successful migration in P. flavescens.
This preliminary study is correlative and thus provides only indirect evidence of micro-evolutionary natural selection of wing shape in a migratory dragonfly. Individuals that made a successful migration to the Maldives had a broader wing base and an overall more slender wing than those sampled in India. However, further studies with larger sample sizes (ideally from more than just two locations along the proposed migration track), and using additional methods such as mark-recapture, radio transmitters, satellite tracking or stable isotope information, are needed to provide direct evidence that wing shape is indeed driven by long-distance migration in P. flavescens.
Acknowledgements
For assistance with specimen collection in the Maldives, we thank Ibrahim Faizan, Yoosuf Rilwan, Mohamed Ushan, Ahmed Najeeb and Adam Shareef. FJ was supported by the Swedish Research Council.
Supplementary material
To view supplementary material for this article, please visit https://doi.org/10.1017/S0266467421000444