Post-weaning diarrhoea, induced by enterotoxigenic Escherichia coli (ETEC), is one of reasons causing poor growth performance and swine disease. The in-feed antibiotics have been widely used in weaning diets to prevent diarrhoea incidences and as growth promoters in piglets( Reference Pluske, Pethick and Hopwood 1 , Reference Lange, Pluske and Gong 2 ). Since the pharmacological dose of ZnO at 3000 mg/kg was first reported to reduce diarrhoea and increase growth rates in weaned pigs( Reference Poulsen 3 ), moreover, many studies have confirmed this improvement on intestinal health and body weight (BW) gain in the first 2 weeks after weaning( Reference Heo, Kim and Hansen 4 – Reference Walk, Wilcock and Magowan 7 ). Accordingly, the combination of in-feed antibiotics and pharmacological dose of ZnO had been widely used in pig diet. However, the environmental pollution and antibiotics resistance of pathogen, resulting from in-feed antibiotics and ZnO( Reference Sales 8 , Reference Looft, Johnson and Allen 9 ), promotes us to seek the alternative strategies.
Live yeast (LY) (Saccharomyces spp.) has been used as a preventive and therapeutic agent for intestinal diseases in humans and animals( Reference Zanello, Meurens and Berri 10 ). The Saccharomyces cerevisiae CNCM I-4407 strain has been found to have anti-inflammatory effect( Reference Zanello, Berri and Dupont 11 – Reference Badia, Lizardo and Martinez 13 ), increase antibody levels of sow milk and immunity of piglets( Reference Jurgens, Rikabi and Zimmerman 14 ) and reduce incidences of diarrhoea, as well as the mortality of piglets infected with ETEC( Reference Trckova, Faldyna and Alexa 15 , Reference Trevisi, Colombo and Priori 16 ). To our knowledge, however, the effects of replacing both antibiotics and zinc oxide by LY on growth performance, diarrhoea severity and intestinal and immunological parameters have rarely been reported. In this study, we hypothesised that the inclusion of LY in the diet would maintain similar effects as the combination of antibiotics and zinc oxide on growth, diarrhoea, intestinal permeability and immunological function of piglets challenged with ETEC.
Methods
Ethics approval
All experimental protocols for the present study were approved by the Animal Care and Use Committee of Sichuan Agricultural University, and carried out in accordance with the National Research Council’s Guide for the Care and Use of Laboratory Animals.
Animals, housing and experimental design
A total of 108 piglets (Duroc×(Landrace×Yorkshire)), weaned at 21 (se 2) d of age, were moved to the piglet experimental house with a controlled temperature at 28–30°C. The piglet experimental house contained eighteen pens (1·5×1·5 m) with infrared lamps (250 W) hanged above the pens. Before weaning, no creep feed was supplied. The initial BW of piglets was 6·39 (se 0·05) kg and randomly allocated into three dietary treatments with six pens and six piglets (three barrows and three gilts) per pen, receiving the control diet (CON), diet supplemented with antibiotic plus ZnO (ANT–ZnO, 20 mg/kg of Colistin+75 mg/kg of Aureomycin+2100 mg/kg of ZnO) and diet supplemented with LY S. cerevisiae (strain CNCM I-4407, 1010 colony-forming units (CFU/g) Actisaf®; Phileo Lesaffre Animal Care) at 1 g per kg diet (LY), respectively. The ingredient composition and nutrient levels of CON diet are presented in Table 1; ANT–ZnO and LY diets were formulated at the expenses of maize grain. All piglets had free access to feed and water; the experimental period lasted for 2 weeks.
Table 1 Ingredient composition and nutrient levels of CON diet (as fed basis, %)
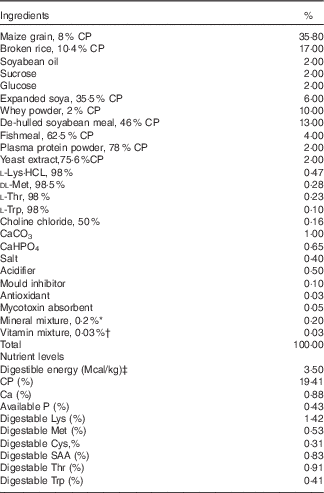
CP, crude protein.
* The mineral premix provided the following per kg of complete diet: Fe, 150 mg; Cu, 195 mg; Zn, 150 mg; Mn, 30 mg; iodine, 0·3 mg; Se, 0·3 mg.
† The vitamin premix provided the following per kg of complete diet: vitamin A, 3·6mg; vitamin D3, 0·8mg; vitamin E, 80 mg; vitamin K3 32·50 mg; vitamin B1 2·50 mg; vitamin B2 6·50 mg; vitamin B6 5 mg; vitamin B12 50 μg; nicotinic acid, 45 mg; pantothenic acid, 20 mg; folic acid, 1·50 mg; biotin, 0·15 mg.
‡ 14·6 MJ/kg.
Enterotoxigenic Escherichia coli K88 challenge
On day 8, two male piglets in each pen in the CON group and one male piglet in each pen in the ANT–ZnO and LY groups were selected to be individually fed in metabolic cages (1·2×0·4×0·5 m), with environmentally controlled rooms (28–30°C). The selected piglets were healthy with BW near the average BW of each pen. Piglets from the CON group received either 100 ml of sterilised Luria broth as the unchallenged group (CON, n 6) or Luria Broth containing 109 CFU/ml ETEC (serotype O149:K91:K88ac; China Veterinary Culture Collection Center) as the challenge group (CON–ETEC, n 6), whereas piglets from ANT–ZnO (n 6) and LY (n 6) groups also received ETEC as challenge groups named by ANT–ZnO–ETEC and LY–ETEC groups. During the challenge study, piglets from CON, ANT–ZnO or LY groups were fed CON, ANT–ZnO or LY diets, respectively. The ETEC-challenged and unchallenged piglets were housed in separate sanitary rooms to avoid cross-contamination between groups. The diarrhoea scores were recorded at 6, 12, 24 and 36 h after ETEC challenge (1, normal; 2, pasty; 3, semi-liquid; 4, watery) and considered diarrhoeic when scored for 3 and 4.
Collection of blood and tissue samples
At 08.00 hours after 2 d of ETEC challenge, blood samples were collected via the anterior vena cava puncture, and 5 ml of blood sample was injected into sodium heparin anti-coagulative tubes. Blood samples with sodium heparin anticoagulation were centrifuged (3000 g , 15 min, 4°C) to obtain plasma samples and stored at −80°C for assays. Immediately after the collection of blood samples, piglets were euthanised with intramuscular injection of 15 mg/kg BW of pentobarbital sodium and slaughtered. As the previous study( Reference Hou, Wang and Ding 17 , Reference Hu, Liu and Yan 18 ), the duodenal, jejunal and ileal tissue samples of about 2 cm in length were stored in 4 % paraformaldehyde solution for histological analyses. Ileal segments (10 cm in length) were opened longitudinally and the contents were flushed with ice-cold PBS. Mucosa was collected by scraping using a sterile glass microscope slide at 4°C, rapidly frozen in liquid N2 and stored at −80°C for the analysis of protein expression. Another ileum (3 cm in length) and mesenteric lymph node (MLN) tissue samples were collected, snap-frozen and stored at −80°C for the analysis of mRNA expression. Aliquots (10 g) of freshly collected digesta from the proximal colon were put into steril Eppendorf tubes and immediately stored at –70°C for bacterial analyses( Reference Shen, Yi and Ni 19 ).
Measurements
Growth performance
Throughout the study, the feed supplied to piglets per pen was recorded daily. Individual piglet BW and feed disappearance per pen was measured weekly to calculate average daily gain (ADG), average daily feed intake (ADFI) and F:G (ADFI:ADG ratio) for weeks 1 and 2, respectively.
Immunological parameters
Plasma concentrations of IL-6, IL-1β and IgA were evaluated using the spectrophotometric method (Spectra Max M2; Molecular Devices), following the protocols of commercially available ELISA kits (Nanjing Jiancheng Bioengineering Institute). The minimal detection limit was 0·5 ng/l for IL-1β, 2 ng/l for IL-6 and 10 µg/ml for IgA, respectively. In addition, the plasma concentrations of IgG and IgM were detected using the automatic biochemical analyzer (Model 7020; Hitachi), followed the protocols from the assay kits (Sichuan Maker Biotechnology Co. Ltd); the minimal detection limit was 0·8 g/l for IgG and 30 mg/l for IgM, respectively. The intra-assay and inter-assay CV were <5 % for each assay.
Intestinal morphology
The duodenal, jejunal and ileal samples were embedded in paraffin. Each sample (duodenum, jejunum and ileum) was used to prepare five slides, and each slide had three sections (5-μm thickness), which were stained with haematoxylin–eosin for intestinal morphology measurement by twenty well-oriented villi and crypts in each section (Optimus software version 6.5; Media Cybergenetics), and villous height:crypt depth ratio (VCR) was calculated.
Intestinal permeability
For the test of intestinal permeability, at 4 h before euthanasia, the piglets were dosed intra-gastrically with 20 ml of distilled water containing 500 mg lactulose/kg BW (Sigma-Aldrich) and 50 mg mannitol/kg BW (Sigma-Aldrich). Urinary concentrations of lactulose and mannitol (10 ml), collected by cystocentesis at the time of euthanasia, were measured using an enzymatic spectrophotometric method (Spectra Max M2), following the protocols of commercial kits for lactulose (GMS19199.3; GENMED SCIENTIFICS INC.) and mannitol (GMS19049.4, GENMED SCIENTIFICS INC.)( Reference Behrens, Docherty and Elia 20 , Reference Blood, Ingle and Allison 21 ). The urinary recovery (% of administered dose) of lactulose or mannitol was calculated, and the ratio between the recovery percentages of lactulose and mannitol was considered as an index of intestinal permeability( Reference Zhang and Guo 22 ).
Gene expressions
As the previous study by Hu et al.(
Reference Hu, Liu and Yan
18
), total RNA was extracted from the frozen ileum and MNL tissues using Trizol Reagent (Invitrogen) according to the manufacturer’s instructions. The quality and purity of RNA samples were assessed by electrophoresis on 1·0 % agarose gel and nucleic acid analyzer (A260/A280, Beckman DU-800; Beckman Coulter, Inc.), respectively. Subsequently, the RNA was performed at 37°C for 15 min, followed by RT inactivation at 85°C for 5 s using Prime Scripte RTTM reagent kit (Takara). Quantitative RT-PCR was performed on a ABI-7900HT instrument (Applied Biosystems). Oligonucleotide primers were used to detect the expressions of the target genes and the reference gene (β-actin) using the SYBR green system (Takara). The primer sequences are listed in Table 2. The reaction mixture (10 μl) contained 5 μl of fresh SYBR®Premix Ex TaqII (Tli RNase H Plus) and 0·2 μL of ROX Reference Dye II (50×), 0·8 μl of the primers, 1 μl of RT products and 3 μl of diethylpyrocarbonate-treated water. The following PCR protocol was used: one cycle at 95°C for 30 s, forty cycles at 95°C for 5 s and 60°C for 31 s and one cycle at 95°C for 15 s, 60°C for 1 min and 95°C for 15 s. The standard curve of each gene was run in duplicate and three times for obtaining reliable amplification efficiency values. The correlation coefficients (r) of all the standard curves were >0·99, and the amplification efficiency values were between 90 and 110 %. The β-actin transcript was used to standardise the mRNA expression of all genes. The relative quantification of gene expression among the treatment groups was analysed by the
$$2^{{{\minus}\Delta \Delta C_{t} }} $$
method(
Reference Livak and Schmittgen
23
).
Table 2 Primer sequences of target and reference genes

TLR, Toll-like receptor; MyD88, myeloid differentiation factor 88; TRAF-6, TNF receptor-associated factor 6; SIGIRR, single Ig IL-1-related receptor; TOLLIP, Toll-interacting protein; IL, IL; ZO-1, Zonula occludens protein-1.
Western blot analysis for protein expressions
Western blot analysis was performed as previously described( Reference Guo 24 ); the frozen ileal mucosa was homogenised with cell lysis buffer for extracting total protein according to the method described for the cell lysis buffer in the Western and IP kit (Beyotime), and the protein concentration was determined using the Bicinchoninic Acid Protein Assay Kit (Pierce). Equal amounts of protein lysates (100 μg) were separated on 12 % SDS-PAGE and then transferred to apolyvinylidene fluoride membranes (Bio-Rad Laboratories), which were blocked in TBS-T buffer (50 mm TRIS-HCl, 150 mm NaCl, 0·1 % Tween, pH 7·6) supplemented with 5 % bovine serum albumin (Sigma-Aldrich) at room temperature for 1·5 h, followed by overnight incubation at 4°C with diluted antibodies against claudin-1 (1:250; Invitrogen), occludin (1:1000; Invitrogen) and β-actin (1:500; Santa Cruz). After 1 h of incubation with horseradish peroxidase-linked secondary antibody anti-mouse IgG (1:2000; Cell Signaling) at room temperature, chemiluminescence detection was performed using the ECL Plus TM Western Blotting Detection System (Amersham), according to the manufacturer’s instructions. The relative expression of target protein was normalised using β-actin as the internal protein, and then the normalised values were used for comparison of the expression of target proteins across groups.
Bacterial counts in colonic contents
The frozen colonic contents were thawed for 10 min. Samples were immediately weighed and serially diluted up to 107 in sterile physiological saline. Dilutions were subsequently plated on duplicate selective agar media for enumeration of bacteria, including E. coli, Lactobacillus spp. and Bifidobacterium spp. The appropriate selective agar media, incubation conditions and period and colony identification were in accordance with those described by Giannenas et al.( Reference Giannenas, Papadopoulos and Tsalie 25 ). In brief, diluted samples were plated on Man–Rogosa–Sharpe agar and Eosin Methylene Blue agar (Microbiology Laboratories) following anaerobic or aerobic incubation at 37°C for 24 h to count Lactobacillus spp. and E. coli, respectively, and plated on Reinforced Clostridial Agar (Microbiology Laboratories) following anaerobic incubation at 37°C for 48 h to count Bifidobacterium spp. Anaerobic incubation was achieved under anaerobic atmosphere (80 % N2, 15 % CO2 and 5 % H2) without agitation. Results were expressed as base-10 logarithm CFU/g (wet mass) of colonic content (CFU/g).
Statistical analysis
The performance data were calculated separately for weeks 1 or 2, and the pen was recognised as a statistical unit for ADG, ADFI and F:G. The selected piglet in each pen was taken as an experimental unit for the parameters related to the intestinal and immunological function in the challenge study. Before the analyses, values of bacterial counts in colonic contents were transformed to log10 values. Data were analysed using the general linear models (GLM) procedure of SPSS 20.0 (SPSS Inc.). The change of diarrhoea scores of weaned piglets following an ETEC challenge was analysed by an unequally spaced repeated-measures and multivariate ANOVA process of the GLM in SPSS. Multi-comparison was conducted by Duncan’s multiple-range test. The difference was considered to be significant at P<0·05.
Results
Performance
During the 1st week, piglets fed LY diet had lower (P<0·05) ADG and ADFI than piglets fed ANT–ZnO diet (Table 3). During the 2nd week, however, piglets fed LY diet had similar ADG as piglets fed ANT–ZnO diet.
Table 3 Growth performance of weaned piglets fed diets supplemented with antibiotics plus zinc oxide (ANT–ZnO) or live yeast (LY) (Mean values with their standard errors)

BW, body weight; ADG, average daily gain; ADFI, average daily feed intake; F:G, feed:gain ratio.
a,b Mean values within a row with unlike superscript letters were significantly different (P<0·05).
Diarrhoea score and morphology
Piglets with CON–ETEC had the higher diarrhoea scores (P<0·05) than piglets with CON or ANT–ZnO–ETEC during the 24-h post challenge. However, the diarrhoea score in piglets with LY–ETEC had similar value as piglets with ANT–ZnO–ETEC at 24 h after ETEC challenge (Fig. 1). In addition, piglets with CON–ETEC had lower villous height in both jejunum and ileum (P<0·05) than that of piglets with CON, but piglets with LY–ETEC or ANT–ZnO–ETEC had similar villous height in jejunum as piglets with CON or CON–ETEC. Although piglets with ANT–ZnO–ETEC had higher villous height in the ileum (P<0·05) than piglets with CON–ETEC, the villous height in the ileum did not markedly differ between piglets with LY–ETEC and ANT–ZnO–ETEC (Fig. 2(A)). There were no significant differences in the crypt depth (Fig. 2(B)) and ratio of VCR (Fig. 2(C)) across all treatments.

Fig. 1 Diarrhoea scores of weaned piglets fed diets supplemented with antibiotics plus zinc oxide (ANT–ZnO) or live yeast (LY) following an enterotoxigenic Escherichia coli K88 (ETEC) challenge. , Control diet (CON);
, CON–ETEC;
, ANT–ZnO–ETEC;
, LY–ETEC. Values are means, with their standard errors represented by vertical bars. a,b,c Mean values with unlike letters were significantly different (P<0·05).

Fig. 2 Small-intestinal (A) villous height, (B) crypt depth and (C) the ratio of villous height:crypt depth (VCR) of weaned piglets fed diets supplemented with antibiotics plus zinc oxide (ANT–ZnO) or live yeast (LY) following an enterotoxigenic Escherichia coli K88 (ETEC) challenge. Values are means, with their standard errors represented by vertical bars. , Control diet (CON);
, CON–ETEC;
, ANT–ZnO–ETEC;
, LY–ETEC. a,b,c Mean values with unlike letters were significantly different (P<0·05).
Intestinal permeability and claudin-1 abundance
The LR and LR:MR ratio were increased (P<0·05) in the urine of piglets with CON–ETEC compared with piglets with CON; however, piglets with LY–ETEC or ANT–ZnO–ETEC had similar values on LR and LR:MR ratio as piglets with CON (Fig. 3). Relative to piglets with CON, the expression of intestinal tight junction protein claudin-1 was markedly lower (P<0·05) in the ileum of piglets with CON–ETEC, whereas piglets with LY–ETEC or ANT–ZnO–ETEC maintained similar expression of claudin-1 as piglets with CON (Fig. 4(A)). There was no significant difference in the protein expression of occludin (Fig. 4(B)) and the gene expression of zonula occludens protein-1, occludin and claudin-1 (Fig. 4(C)) across all treatments.

Fig. 3 Urinary recovery (% of administered dose) of lactulose (LR) and mannitol (MR) and lactulose:mannitol ratios (LR:MR) of weaned piglets fed diets supplemented with antibiotics plus zinc oxide (ANT–ZnO) or live yeast (LY) following an enterotoxigenic Escherichia coli K88 (ETEC) challenge. Values are means, with their standard errors represented by vertical bars. , Control diet (CON);
, CON–ETEC;
, ANT–ZnO–ETEC;
, LY–ETEC. a,b Mean values with unlike letters were significantly different (P<0·05).

Fig. 4 Relative protein expressions of (A) claudin-1 and (B) occludin in ileum mucosa of weaned piglets fed diets supplemented with antibiotics plus zinc oxide (ANT–ZnO) or live yeast (LY) following an enterotoxigenic Escherichia coli K88 (ETEC) challenge. (C) Relative mRNA abundance of zonula occludens protein-1 (ZO-1), occludin and claudin-1 in ileum of weaned piglets fed diets supplemented with ANT–ZnO or LY following an ETEC challenge. Values are means, with their standard errors represented by vertical bars. , Control diet (CON);
, CON–ETEC;
, ANT–ZnO–ETEC;
, LY–ETEC. a,b Mean values with unlike letters were significantly different (P<0·05).
Bacterial counts in colonic contents
The E. coli counts were increased (P<0·05) in the colonic contents of piglets with CON–ETEC compared with piglets with CON, whereas the counts of both E. coli and Lactobacillius were markedly decreased in piglets with ANT–ZnO–ETEC. However, the counts of Lactobacillus were markedly higher (P<0·05) in piglets with LY–ETEC than piglets with CON–ETEC or ANT–ZnO–ETEC (Fig. 5).

Fig. 5 The bacterial count in the colonic digesta of weaned piglets fed diets with antibiotics plus zinc oxide (ANT–ZnO) or live yeast (LY) following an enterotoxigenic Escherichia coli K88 (ETEC) challenge. Values are means, with their standard errors represented by vertical bars. CFU, colony-forming units; , control diet (CON);
, CON–ETEC;
, ANT–ZnO–ETEC;
, LY–ETEC. a,b,c Mean values with unlike letters were significantly different (P<0·05).
Immunological and inflammatory responses
ETEC challenge markedly decreased the plasma concentration of IgA than that of piglets without ETEC challenge. Moreover, piglets with CON–ETEC had markedly higher concentration of plasma IL-6 (P<0·05) than that of piglets with CON; however, piglets with LY–ETEC or ANT–ZnO–ETEC had similar concentration of plasma IL-6 as that of piglets with CON (Table 4).
Table 4 Immunoglobulin and inflammatory responses of weaned piglets fed diets supplemented with antibiotics plus zinc oxide (ANT–ZnO) or live yeast (LY) following an enterotoxigenic Escherichia coli K88 (ETEC) challenge (Mean values with their standard errors)

CON, control diet.
a,b Mean values within a row with unlike superscript letters were significantly different (P<0·05).
Innate-immunity-related gene expressions
The mRNA expressions of genes in the ileum (myeloid differentiation factor 88 (MyD88) and IL-6) and MLN (NF-κB and IL-1β) were up-regulated (P<0·05) in piglets with CON–ETEC compared with piglets with CON, but these genes did not markedly differ between piglets with LY–ETEC and ANT–ZnO–ETEC. In addition, the mRNA expression of SIGIRR in both ileum and MLN were markedly up-regulated in piglets with ANT–ZnO–ETEC relative to piglets with CON or CON–ETEC (Fig. 6).

Fig. 6 Relative mRNA abundance in the ileum (A) and mesenteric lymphoid node (MLN, B) of weaned piglets fed diets supplemented with antibiotics plus zinc oxide (ANT–ZnO) or live yeast (LY) following an enterotoxigenic Escherichia coli K88 (ETEC) challenge. Values are means, with their standard errors represented by vertical bars. , control diet (CON);
, CON–ETEC;
, ANT–ZnO–ETEC;
, LY–ETEC; TLR, Toll-like receptor; MyD88, myeloid differentiation factor 88; TRAF-6, TNF receptor-associated factor 6; SIGIRR, single Ig IL-1-related receptor; TOLLIP, Toll-interacting protein. a,b Mean values with unlike letters were significantly different (P<0·05).
Discussion
Antibiotics or/and zinc oxide had been used in starter diet to prevent diarrhoea and stimulate the growth rate of weaning piglets( Reference Walk, Wilcock and Magowan 7 , Reference Bosi, Merialdi and Scandurra 26 , Reference Yin, Li and Li 27 ). As a positive control, in this study, the combination of antibiotics and zinc oxide in the diet had improved ADG and final BW of piglets; however, the inclusion of LY in diet could maintain similar ADG as piglets fed the ANT–ZnO diet during the 2nd week post weaning.
Compared with piglets with CON, during the 24 h after ETEC challenge, piglets with CON–ETEC exhibited diarrhoea with semi-liquid or watery faeces (scored at 3–4), however, which was normalised in piglets with ANT–ZnO–ETEC. Although piglets with LY–ETEC had diarrhoea with pasty or semi-liquid faeces (scored at 2–3) during the 12 h, these piglets had similar diarrhoea score as piglets with ANT–ZnO–ETEC at 24 h after ETEC challenge, indicating the decreasing effect of LY on diarrhoea severity and duration in piglets with ETEC. Consistently, the recent studies showed that both severity and duration of diarrhoea in piglets could be reduced by feeding LY( Reference Trckova, Faldyna and Alexa 15 , Reference Trevisi, Colombo and Priori 16 ). In addition, there was decreased villous height in the jejunum of piglets with CON–ETEC, however, which did not occur in piglets with LY–ETEC or ANT–ZnO–ETEC, suggesting the preventive role of LY or ANT–ZnO on the intestinal integrity of piglets. The previous studies have shown that diarrhoea and poor intestinal integrity are often associated with the activation of innate immunity and inflammatory process, in which the TLR4-Myd88-NF-κB signal pathway is involved( Reference Duan, Zhang and Li 28 – Reference Uematsu and Akira 30 ). In this study, we found that the innate-immunity-related genes that were highly expressed in the ileum tissues of piglets with CON–ETEC were down-regulated in the piglets with LY–ETEC or ANT–ZnO–ETEC, suggesting that the excessive innate immune response of piglets with ETEC may be alleviated by feeding LY or ANT–ZnO diet. Moreover, the inflammatory response of piglets under ETEC challenge may be alleviated by LY or ANT–ZnO, as indicated by the lower concentration of plasma IL-6 in piglets with LY–ETEC or ANT–ZnO–ETEC. Similarly, a recent study also showed that dietary supplementation of Lactobacillus acidophilus GG, as a probiotic strain, could alleviate the inflammatory response of piglets to ETEC by modulating NF-κB and MAPK signalling pathways( Reference Li, Zhang and Chen 31 ). In this study, however, we did not observe the improvement of either LY or ANT–ZnO supplementation on the humoral immunity, as indicated by the decreasing concentration of plasma IgA in piglets with ETEC challenge; this result is inconsistent with the previous study by Trevisi et al.( Reference Trevisi, Casini and Coloretti 32 ), who reported that ETEC challenge could not markedly alter the concentration of total blood IgA in piglets with Lactobacillus rhamnosus GG. The differential immune response of piglets to ETEC challenge may be related to the type of probiotics, ETEC strains or the challenge period. Furthermore, the inhibition of ANT–ZnO on innate immune and inflammatory response may be ascribed to their antibacterial activity( Reference Jensen, Thymann and Cilieborg 33 , Reference Hu, Song and Xiao 34 ) and decreasing adhesion of bacteria( Reference Roselli, Finamore and Garaguso 35 , Reference Zapater, González-Navajas and Such 36 ), as evidenced by the decreasing counts of E. coli and Lactobacillius in piglets with ANT–ZnO–ETEC, relative to piglets with CON–ETEC. In addition, the modulating effect of LY on inflammation or innate immunity had been attributed to the secreted molecules( Reference Zanello, Berri and Dupont 11 , Reference Zanello, Meurens and Berri 12 ) or probiotic effects, as indicated by the increased counts of Lactobacillius in piglets with LY–ETEC relative to piglets with CON–ETEC or ANT–ZnO–ETEC. More recent data suggest that the direct binding effect occurred between LY probiotics and pathogenic bacteria such as E. coli, Salmonella and Listeria ( Reference Broadway, Carroll and Sanchez 37 ), which result in the competitive exclusion of exogenous pathogens from the intestinal lumen. In addition, lactic acid fermentation in S. cerevisiae lowered the gut pH, which may inhibit the proliferation of E. coli ( Reference Ansanay, Dequin and Camarasa 38 ).
The intestinal bacteria has been shown to target various intracellular process including the expression and distribution of tight junction proteins, thereby modulating the intestinal permeability( Reference Ulluwishewa, Anderson and Mcnabb 39 ) and gastrointestinal diseases( Reference Bischoff, Barbara and Buurman 40 , Reference Michielan and D’Incà 41 ). In this study, the intestinal permeability was increased in piglets with ETEC challenge, as indicated by the higher recovery of urine lactulose and ratio of lactulose to mannitol in piglets with CON–ETEC; however, these values did not markedly alter in piglets with LY–ETEC or ANT–ZnO–ETEC, suggesting the improvement of LY or ANT–ZnO on the intestinal permeability. Supportively, the higher expression of tight junction protein claudin-1 was observed in piglets with LY–ETEC or ANT–ZnO–ETEC relative to CON–ETEC. Similarly, the previous studies also showed that the supplementation of LY could improve the barrier function, as indicated by the increased expression of tight junction protein, thickness of mucosal layer and number of mucosal macrophages( Reference Bontempo, Giancamillo and Savoini 42 , Reference Sivignon, De and Barnich 43 ).
Conclusion
The observations in the current study indicate that dietary LY supplementation could alleviate the severity of diarrhoea of piglets with ETEC, which may be associated with the improved intestinal permeability and immunity, as well as bacterial profile.
Acknowledgements
We acknowledge Romain D’Incà, Liang Hu, Xie Peng, Yan Liu, Ru Wang, Linlin Qin, Yang Liu and Qiang Zhou for their suggestions about study design and technical assistance.
This project was funded by Phileo Lesaffre Animal Care, France (B. Z., E. A., grant no. C2729-01); the National Key Research and Development Program of China (L. C., Q. X., C. W., Y. L., grant no. 2016YFD0501204); Sichuan provincial project on S&T application and demonstration (X. H., grant no. 2016CC0070); and the project on commercialisation of research findings under funding of the government of Sichuan province (Z. F., Y. L., S. X., B. F., J. L., D. W., grant no. 16ZHSF0385). The Phileo Lesaffre Animal Care (France) donated the live yeast Saccharomyces cerevisiae (strain CNCM I-4407, Actisaf®) for this study and partly contributed to the study design.
The authors’ contributions are as follows: L. C., Q. X., B. Z. and E. A. contributed to formulate the research questions and study design; Q. X., C. W., Y. L., Z. F., Y. L. and S. X carried out the study; X. H., B. F. and J. L. contributed to the sample analysis; L. C., Q. X. and D. W. contributed to the data analysis; and L. C., Q. X., C. W. and D. W. contributed to the data interpretation.
The authors declare that there are no conflicts of interest.