1. Introduction
Aluminum nitride (AlN) is one of the most promising substrates for next generation high power, high frequency, and short wavelength optoelectronic devices based on gallium nitride (GaN) and its alloys Reference Edgar, Liu, Liu, Zhuang, Chaundhuri, Kuball and Rajasingam[1]. Its extraordinary physical, chemical, optical, and electrical properties make it attractive in III-nitride epitaxial film applications, as addressed elsewhere Reference Edgar, Strite, Akasaki, Amano and Wetzel[2]. The benefits of AlN compared to sapphire or silicon carbide as a substrate for GaN-based devices include a reduced lattice constant mismatch hence lower defect densities and a reduced coefficient of thermal expansion mismatch thus lower thermal stress. Because the structure of AlN is identical to GaN, epitaxial films can be grown on all crystal orientations, not just the (0001) plane. This may eliminate polarization effects and improve the quantum efficiency of nitride semiconductor LEDs and laser diodes. AlN will not alter the charge carrier background concentration in eptiaxial films by diffusion or autodoping as it is composed of only group III and group V elements that are isoelectronic with GaN. The properties of AlN even more closely match those of high Al mole fraction AlGaN epitaxial layers, which are necessary for the fabrication of deep ultraviolet (DUV) optoelectronic devices Reference DenBaars, Hanlon, Katona, Pattison, Kaeding, Sharma, Schmidt, Margalith, Moe, Keller, Cantu, Li and Nakamura[3] Reference Gaska, Khan and Shur[4]. The initial reports on transistors and LEDs using AlN substrates demonstrate its promising characteristics Reference Carlos Rojo, Schowalter, Gaska, Shur, Khan, Yang and Koleske[5] Reference Slack, Carlos Rojo, Schowalter, Morgan, Barani, Schujman, Biswas, Raghothamachar, Dudley, Shur, Gaska, Johnson and Kneissl[6]. In short, using AlN substrates should significantly improve the power density, efficiency, lifetime, and reliability of GaN-based devices.
Currently, several groups are producing bulk AlN crystals by the sublimation-recondensation technique Reference Edgar, Liu, Liu, Zhuang, Chaundhuri, Kuball and Rajasingam[1] Reference Carlos Rojo, Schowalter, Gaska, Shur, Khan, Yang and Koleske[5] Reference Schlesser, Dalmau, Yakimova and Sitar[7] Reference Bickermann, Epelbaum and Winnacker[8]. To achieve good quality bulk AlN single crystals with fast growth rates (>200 µm/h), high temperatures, above 2100 °C, are required. At such high temperatures, the crucible and furnace fixture components are no longer passive, as their vapor pressure may be significant, or they may react with the source materials (AlN powder and nitrogen). The presence of elements from the crucible material may alter the AlN crystal habit by changing the fastest growth direction. Incorporation of impurities from the crucible into the crystal may cause stress or defects. In this paper, attention is focused on the durability and performance of several refractory crucible materials. The most probable species present in the vapor phase and their vapor pressures in the crystal growth system are discussed from the results of thermodynamic analysis. The performance of those crucible materials under the crystal growth conditions is addressed as well.
2. Background
Almost all early works of AlN crystal growth in the 1960s and 1970s employed graphite crucibles Reference Cox, Cummins, Kawabe and Tredgold[9] Reference Rutz[10] Reference Kohn, Cotter and Potter[11] Reference Pastrnak and Roskovcova[12] Reference Krukowska-Fulde and Niemyski[13] Reference Ishii, Sato and Iwata[14] Reference Witzke[15] [16] Reference Kwabe, Tredgold and Inuishi[17], but carbon contamination was significant. Horiuchi and co-workers Reference Horiuchi, Ishii and Asakura[18] produced kite-shape twined AlN crystals by using graphite crucibles. Dark particles were attached to the corner of crystals. They suggested that Al2OC might be partly formed in the crystal structure of AlN Reference Ishii, Sato and Iwata[14]. To suppress carbon contamination, SiC coated graphite crucibles were employed Reference Balkas, Sitar, Zheleva, Bergman, Nemanich and Davis[19] Reference Balkas, Sitar, Zheleva, Bergman, Shmagin, Muth, Kolbas, Nemanich and Davis[20] Reference Hossain, Lindesay and Spencer[21]. Carbon containing precipitates were avoided and kite shaped crystals were no longer observed, but the coating eventually degraded, because of sublimation of the SiC coating and the diffusion of aluminum through the SiC barrier, reacting with the graphite and causing the coating to spall.
To avoid carbon contamination, Slack and McNelly Reference Slack and McNelly[22] Reference Slack and McNelly[23], as well as other researchers Reference Carlos Rojo, Schowalter, Gaska, Shur, Khan, Yang and Koleske[5] Reference Bickermann, Epelbaum and Winnacker[8] Reference Shi, Liu, Liu, Edgar, Meyer, Payzant, Walker, Evans, Swadener and Chaudhuri[24], chose tungsten for crucibles due to its high melting point and resistance to attack by Al vapor up to 2275 °C under 1 atm of nitrogen Reference Slack and McNelly[23]. Relatively large AlN (up to 15 mm in diameter) crystals have been grown in tungsten crucibles. At extremely high temperatures (2330 °C) the crucibles eventually failed when pinholes form at the tungsten grain boundaries. Schowalter and Slack Reference Schowalter and Slack[25] speculated that this was due to aluminum dissolving into the tungsten (approximately 5.3 wt%), causing the lattice constant of the tungsten to shrink and the grains to separate. Eliminating spurious polycrystalline growth, cracking, and the presence of highly strained regions Reference Carlos Rojo, Schowalter, Morgan, Florescu, Pollak, Raghothamachar and Dudley[26] are all major concerns with tungsten as the crucible.
Boron nitride is another choice for crucible material Reference Schlesser and Sitar[27] in AlN crystal growth. BN does not wet or react with either Al or nitrogen, and hot-pressed boron nitride (HPBN) is easy to machine. Our research group obtained clear and colorless thin platelets up to 60 mm2 in HPBN crucible Reference Liu, Edgar, Raghothamachar, Dudley, Lin, Jiang, Sarua and Kuball[28].
To date, there has been no systematic investigation of the effects the furnace fixture materials on both the crystal growth process and final properties of the AlN crystals. Materials suitable for bulk AlN crystal growth must have the following properties:
-
1. The material must have a melting point in excess of 2300 °C;
-
2. it must not form low melting temperature eutectics with Al, N, or the heating elements of the furnace (typically, graphite or tungsten);
-
3. it should not react with Al vapor, N2, or the heating elements, or the reaction should be self-limited;
-
4. its vapor pressure should be relatively low compared to that of Al and N2 over AlN.
In practical terms, the crucible material should not contain oxygen, because the great affinity of aluminum for oxygen makes these two elements very likely to react. This set of criteria suggests that potential candidates are limited to several pure refractory metals (W, Ta, Nb, and Hf), refractory carbides, nitrides, and borides.
Table 1 summarized the reactivity of these materials as reported in the literature, all of which have melting points higher than 2600 °C, excellent high-temperature strength, and good corrosion resistance. Aluminum reacts with transition-metal carbides and nitrides films only to a limited extent, and created pinholes into the films Reference Wittmer[29]. However, TaC and TiC particles have high stability and uniform distribution in molten Al up to 1000 °C Reference Kobashi, Harata and Chioh[30]. From calculation Reference Kaptay, Akhmedov and Borisoglebskii[31], the relative tendencies of these compounds to react with molten Al were HfC < TaC < NbC < TiC < WC, and TaN< TiN. Yet, there are no reports in the literature discussing the reactions between Al vapor and refractory carbides and nitrides in a carbon and nitrogen rich environment, i.e. in a resistively heated graphite furnace.
Table 1 Reactivities of selected crucible materials with Al, N, C, and W as reported in the literature.
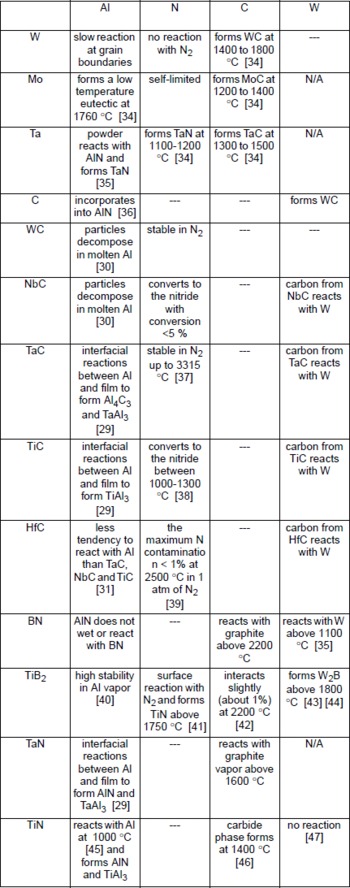
In the following sections, the performance and effects of several materials on AlN crystal growth are reported. First thermodynamic calculations were made to predict the vapor pressures of the candidate materials. Next, the candidate crucible materials were tested in two furnaces with different heating elements: one used tungsten, the other graphite. Tungsten crucibles were used in the tungsten furnace, since the reaction between tungsten and graphite at the temperature range from 1400 °C to 1800 °C will seriously harm the crucible and the crystal growth process. Similarly, BN crucible can only be used in a graphite furnace because early studies by our group showed BN crucibles react with tungsten. At the crystal growth temperature, pure refractory metals will react with the carbon and/or nitrogen in graphite furnace under nitrogen ambient. Thus, only some refractory carbides/nitrides might be considered as candidate materials in graphite system.
3. Thermodynamic Calculations
This section discusses the vapor pressure of refractory metals over solid materials from thermodynamic calculations. Calculations were made for the most probable species present in the system under the crystal growth conditions. Predictions were also made on the crucible materials that fulfilled all four requirements. The lower the vapor pressure of a species, the less the tendency there is for its elements to incorporate into the AlN crystals.
The equilibrium vapor pressures were calculated from Joint-Army-Navy-Air Force (JANAF) thermodynamic data Reference Stull and Prophet[32] by STANJAN, written by W. Reynolds of Stanford, using minimization of the total Gibbs free energy. Over the temperature range from 1700 °C to 2600 °C, the equilibrium vapor pressures were calculated for two different situations: for pure refractory metals in equilibrium with their solids under 1 atmosphere of an inert gas, i.e. argon; and for the refractory metal in equilibrium with its solid-state nitride and/or carbide in the presence of graphite and 1 atmosphere of nitrogen. The later simulated the conditions of the graphite furnace. The vapor pressures of C, Nb, Ta, and W over their solids at the first equilibrium are plotted in figure 1. The vapor pressure of Ti over solid titanium diboride under 1 atm of inert gas is also included in figure 1. Nb, Ta, and W each have a lower vapor pressure than carbon within the temperature range from 1700 °C to 2600oC. Table 2 lists the presumed species present in the vapor phase and solid phase under the second equilibrium for each candidate, and the calculated molar ratio of carbide over the total of carbide and nitride. In the second situation, we calculated the vapor pressure of Al, B, Ti, Nb, W, and Ta over their carbide and/or nitride. Figure 2 shows the calculated equilibrium vapor pressure for candidate crucible material in graphite system under the second situation, as well as Al vapor over AlN. B and Ti have much higher vapor pressure than Nb, W, and Ta, where Ta has the lowest vapor pressure in this graphite system.
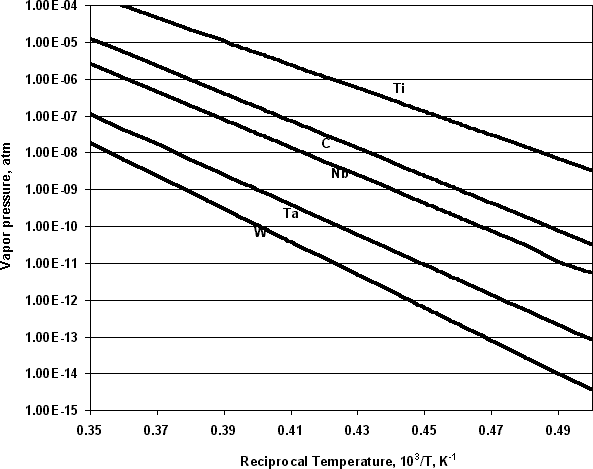
Figure 1. Pure metal vapor pressure over metal solid at 1 atmosphere of inert gas.
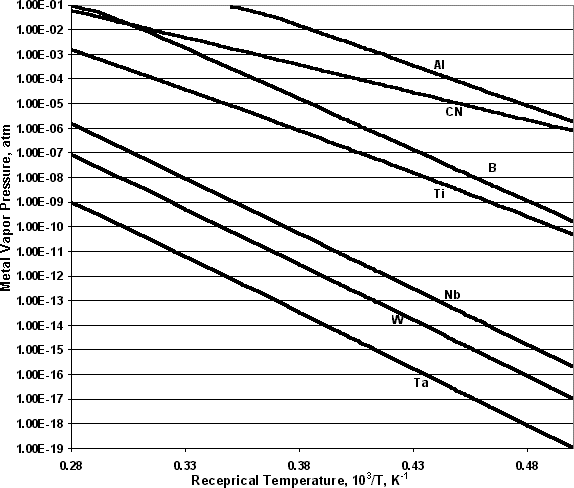
Figure 2. Refractory metal vapor pressure over their carbides and/or nitrides in graphite furnace system at 1 atmosphere of nitrogen.
Table 2 Presumed species present in the graphite furnace under the crystal growth condition.
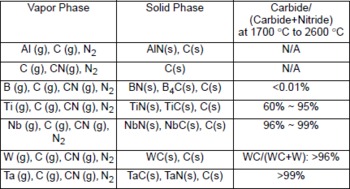
From these calculations, the following conclusions may be drawn.
-
1. BN will not convert to boron carbide;
-
2. TaC and NbC exist stably in the system with very little tendency to form nitrides;
-
3. TiC will transform into a mixture of carbide and nitride, with carbide dominating as temperature increases;
-
4. W will react with graphite and form WC.
From the thermodynamic calculations of those candidate materials, we predict that TaC and NbC are the most stable crucible materials with very low elemental vapor pressure in a graphite furnace under the crystal growth conditions.
4. Crystal growth
4.1 Experimental procedure and characterization
In the crystal growth process, the reaction AlN (s) <-> Al (v) +1/2 N2 is driven in the forward direction at the source material, and in the reverse direction at the crystal growth zone by a slight reduction in temperature. The source material was AlN powder with elemental analysis of O less then 0.9 wt% and C less than 0.04 wt%. Tungsten crucible was used in tungsten furnace. All other candidate crucibles, including TaC, NbC, HPBN, and graphite, were used in graphite furnace.
The AlN crystals were grown between 1800 °C and 2200 °C. Ultra high purity nitrogen continuously flowed through the system at 800 torr to prevent the heating element from reacting with trace oxygen. The growth environment was always nitrogen-rich as the nitrogen pressure is orders of magnitude higher than the saturation vapor pressure of nitrogen over AlN, which is less than 9.9×10−3 atm at the growth temperature range Reference Slack and McNelly[23]. The temperature difference between the source zone, where AlN source sublimes, and the growth zone, where Al and nitrogen recondense, was less than 10 °C as determined by the premeasured temperature profile.
Macrostructure features of the as-grown AlN crystals were characterized by optical microscopy at magnifications of 10-200X. Micro-Raman spectra were obtained using a Renishaw UV micro-Raman system with the 325 nm line of a HeCd laser as the excitation source. The spot size and the spectral resolution were 1-2 μm and 3-4 cm−1, respectively. X-ray topographies were taken at the Stony Brook Synchrotron Topography Station. Topographies were recorded on 8” ×10” Kodak Industrex SR-45-1 high-resolution X-ray film.
4.2 Growth of AlN in TaC coated graphite crucible
For the first time, TaC coated graphite crucible was employed in bulk AlN crystal growth. Amber colored AlN crystals were produced in a TaC coated graphite crucible at 2074 °C and 805 torr for 24 hours. The AlN crystals nucleated in high density on the TaC, and grew into a polycrystalline mass. The phonon frequency of E2(high) mode and A1(TO) mode from Raman characterization are 655 cm−1 and 612 cm−1, with line widths are 7 cm−1 and 15 cm−1 respectively, indicating the AlN crystal's high quality. However, since the AlN wetted with TaC, it was very difficult to separate the crystals from the nucleation surface. A scanning Auger microscopy (SAM) line scan on the cross-sectional area of the TaC foil with AlN crystal shows that the TaC foil lose carbon and pick up small amount of nitrogen (figure 3). Although the TaC film has good process durability and good crystal nucleation characteristics, pinholes in the coating lead to the reactions between the Al vapor and underlying graphite, and cause the coating to spall off.

Figure 3. Line scan of the cross-sectional area of TaC foil with AlN crystal showing N “segregation” and C “depletion”.
4.3 Growth of AlN in NbC coated graphite crucible
Prism shaped AlN needles and hexagonal hillocks of millimeter size crystals were grown on the nucleation surface with high nucleation density. However, NbC coated graphite crucibles suffered from the same pinhole problem as TaC coated crucibles. SAM characterization shows the NbC coating is stable against Al, and nitrogen is detected from the damaged NbC coating, primarily at the grain boundaries and not in the bulk NbC. From selective etching analysis in 45 wt% KOH solution, we infer that freely nucleated crystals predominately have the nitrogen to aluminum direction pointing out from the nucleation surface, which means that the ends of the AlN crystals facing the source are aluminum polarity Reference Zhuang, Edgar, Liu, Liu and Walker[33].
4.4 Growth of AlN in tungsten crucible
Typically, amber colored AlN crystals are produced in tungsten crucibles with a high nucleation density in a three-dimensional growth mode. Sliced and polished AlN crystals grown in W crucible have grain size about 0.5mmx0.5mm (Figure 4). The growth temperature was about 2000 °C and growth time was 14 hours. Due to a high nucleation density, it was hard to get single crystalline seeds from this kind of samples for the subsequent seeded growth. The crystals grown in tungsten crucible contain no intentional impurities, but W and O are inherited from the crucible and source material. Neutron activation analysis revealed 0.2 to 4.6 ppm wt W in the grown crystals, lower than the 7.9 ppm wt reported by Bickermann Reference Bickermann, Epelbaum and Winnacker[8]. However, combustion analysis shows an average value of 500 ppm wt O in the grown crystals, which is higher than the 86 ppm wt from Bickermann's result Reference Bickermann, Epelbaum and Winnacker[8]. There is no reaction between the tungsten furnace fixtures and nitrogen under the growth condition, thus no tungsten nitride, which decomposes at temperature less than 800 °C Reference Toth[34], formed during AlN crystal growth.

Figure 4. Sliced and polished AlN crystal grown in tungsten crucible.
4.5 Growth of AlN in graphite crucible
Growth in graphite crucibles consistently produces needles and kite-shaped twinned crystals bounded on edges along the c direction. These platelets are transparent and colorless m-plane (1


Figure 5. Needle and kite-shape AlN crystals grown in graphite crucible.
4.6 Growth of AlN in HPBN crucible
Hot pressed boron nitride (HPBN), pyrolytic boron nitride (pBN) and a titanium diboride/BN composite (60% TiB2, 40% BN), were investigated as crucible materials for bulk AlN crystal growth. Only AlN needles were obtained in TiB2/BN crucibles under the typical crystal growth condition. The pBN crucible began to form pits on the crucible wall at a temperature about 1800 °C. At higher temperatures, the crucible wall began to peel until it finally failed. The HPBN crucible was the most durable crucible of these three boron-based crucibles. Although the HPBN crucible encountered mass loss each run, which is less than 0.30 wt% per hour, a crucible with 2mm wall thickness can survive several hundred of hours under the growth conditions.
Clear and colorless AlN platelets up to 60 mm2 were prepared in HPBN crucibles by sublimation-recondensation technique under 2100 °C, 800 torr for 48 hours (Figure 6). Crystals grown in HPBN crucibles typically form thin platelets with the fastest growth rate above 400 μm/hr occurring in the c-axis direction. In the a-direction, the growth rate is much lower, about 50 μm/hr under the same growth condition. Growth striations run the length of the crystals in the c-direction, the cause of which has not been identified. In contrast to the other crucible materials employed in this study, the AlN nucleation density was relatively low on HPBN, and the crystals did not agglomerate together to form a dense polycrystalline mass. Instead, it was highly porous. Figure 7 shows the Raman spectrum for AlN crystals grown in HPBN crucibles. There is no significant difference in phonon frequency between sample with and without striations. The phonon frequency of E2 (high) mode and A1 (TO) mode from Raman characterization for both samples are 655 cm−1 and 609 cm−1. Line widths for both modes are 6 cm−1, which indicates good crystal quality. X-ray topography reveals numerous crystallites concentrate in some regions on the sample surface (figure 8). Crystallites are also scattered in some other regions. The crystallites induce strains and sometimes cracking on the surface, as evident in the x-ray topography. A few inclusions (I) are also observed. This sample appears essentially dislocation-free. However, from thermodynamic calculations, vapor pressure of B over BN in graphite furnace is very high among all the candidate crucible materials under the crystal growth condition, which is 3.5×10−7 atm.

Figure 6. AlN crystal produced in HPBN crucible up to 60 mm2.

Figure 7. Raman spectrum for AlN crystal as seen in figure 6.

Figure 8. Transmission topograph (g = 0002, λ=0.69Å) showing inclusions (I). Arrow marks indicate contrast from surface artifacts such as ridges, scratches, strains, and cracks due to crystallites.
5. Conclusions and future work
-
1. Graphite, silicon carbide, and titanium diboride either react with aluminum or sublime at typical crystal growth temperatures. Consequently, none of these materials are suitable as crucible materials.
-
2. Pure tungsten is a very durable crucible material for AlN crystal growth. However, AlN crystals nucleated together in a high density making it difficult to obtain good quality single crystals in tungsten crucibles.
-
3. Both TaC and NbC are very good candidate crucible materials, as they are compatible with graphite components, have low metal vapor pressures, and do not react with either nitrogen or aluminum. But the machining of both sintered TaC and NbC is difficult, and the costs of these materials are high. These significantly restrain their use as crucible materials. New procedures for producing the carbides from the metals may solve this problem. Coated graphite components are not suitable for AlN crystal growth, as the coatings tend to spall off.
-
4. Compared with TaC or NbC coated graphite, HPBN is a better crucible material for AlN self-seeding growth, as crystals tend to nucleate in a relatively low density and do not merge together. Unfortunately, it difficult to produce large crystals in HPBN crucibles, and the crystals produced are highly striated.
Acknowledgments
We are grateful for the support of this research from BMDO (Contract No: N00014-98-C-0407), ONR (Contract No. N00014-99-1-0104), and the Assistant Secretary for Energy Efficiency and Renewable Energy, Office of Transportation Technologies, as part of the High Temperature Materials Laboratory User Program, Oak Ridge National Laboratory. Oak Ridge National Laboratory is managed by UT-Battelle, LLC, for the U.S. Department of Energy under contract number DE-AC05-00OR22725.