Introduction
Defining the Ediacaran-Cambrian boundary and the precise timing of the Cambrian explosion has occupied the attention of paleontologists for decades. A very good measure of this interest is the considerable amount of work performed by the IUGS Cambrian Subcommission to settle a reference standard for the base of the Cambrian and internationally accepted series and stage subdivisions of the Cambrian system, which still are in progress (Narbonne et al., Reference Narbonne, Myrow, Landing and Anderson1987; Brasier et al., Reference Brasier, Cowie and Taylor1994; Terfelt and Ahlberg, Reference Terfelt and Ahlberg2010; Babcock et al., Reference Babcock, Robison and Shanchi2011; Harvey et al., Reference Harvey, Williams, Condon, Wilby, Siveter, Rushton, Leng and Gabbott2011; Peng and Babcock, Reference Peng and Babcock2011; Ahlberg and Terfelt, Reference Ahlberg and Terfelt2012; Ahn et al., Reference Ahn, Babcock and Hollingsworth2012; Moczydlowska and Yin, Reference Moczydlowska and Yin2012; Peng et al., Reference Peng, Babcock and Cooper2012a, Reference Peng, Babcock, Zuo, Zhu, Lin, Yang, Qi, Bagnoli and Wang2012b; Landing et al., Reference Landing, Geyer, Brasier and Bowring2013). Defining reference standards for the base of Cambrian and its subdivisions has promoted understanding of the successive stages of the Cambrian evolutionary radiation (Landing, Reference Landing1998; Landing et al., Reference Landing, Geyer, Brasier and Bowring2013).
A number of biostratigraphic and geochronologic techniques are used for correlation of the Ediacaran-Cambrian boundary and subdivision of pre-trilobitic lower Cambrian. Traditionally, biostratigraphy of the lower Cambrian has been based on small shelly fossils (SSFs) and trace fossils. Small shelly fossils are largely found in carbonates, whereas trace fossils are more common in siliciclastics (Narbonne et al., Reference Narbonne, Myrow, Landing and Anderson1987). Despite that, both groups are common, diverse, and show rapid changes during this critical time of Earth history (Narbonne et al., Reference Narbonne, Myrow, Landing and Anderson1987), Accordingly, they represent important biostratigraphic tools for the subdivision and correlation of pre-trilobitic strata of the Cambrian (e.g., Steiner et al., Reference Steiner, Li, Qian, Zhu and Erdtmann2007).
In spite of the various attempts to settle internationally accepted series and stage subdivisions of the Cambrian system, the Ediacaran-Cambrian boundary and the subdivision of pre-trilobitic lower Cambrian strata require continued attention. In northern Iran, the Ediacaran–Cambrian Soltanieh Formation is well exposed across the Alborz Mountains, and thus has the potential to illuminate some of these issues. In comparison with Ediacaran–Cambrian successions elsewhere, the ichnologic content of the Soltanieh Formation is poorly known. The aims of this study are to: (1) document the Soltanieh Formation ichnofauna, (2) propose a biozonation scheme for the Soltanieh Formation based on trace fossils, (3) suggest the most plausible position for the Ediacaran-Cambrian boundary in northern Iran by using integration of trace fossil and SSF biostratigraphy, and (4) assess the implications of this ichnostratigraphic scheme with respect to global schemes establishing biostratigraphic zonations for the lowermost Cambrian.
Geological setting
The Soltanieh Formation of the Alborz Mountains, northern Iran, regarded as Ediacaran–lower Cambrian, formed in the Proto-Paleotethys passive margin of northwestern Gondwana (Alavi, Reference Alavi1996). Stöcklin et al. (Reference Stöcklin, Ruttner and Nabavi1964) originally divided the Soltanieh Formation at the type section into three major subdivisions, from bottom to top: the Lower Dolomite Member (123 m), the Chopoghloo Shale Member (247 m), and the Upper Dolomite Member (790 m). This division was later revised by Hamdi et al. (Reference Hamdi, Brasier and Zhiwen1989), who divided the Soltanieh Formation into five members, in ascending order; the Lower Dolomite (165m), the Lower Shale (120 m), the Middle Dolomite (180 m), the Upper Shale (90 m), and the Upper Dolomite (580 m) members.
In addition to its type section in the Soltanieh Mountains, the Soltanieh Formation can be traced to several other localities, including Garmab, Vali-Abad, and the Dalir area along the Tehran–Chalous road, north of Tehran (Fig. 1.1). The Soltanieh Formation overlies the Bayandor Formation in the Soltanieh Mountains and the Kahar Formation in the Alborz Mountains. An Ediacaran age is indicated for the Bayandor and Kahar formations based on stratigraphic relationships in the case of the former and the presence of acritarchs in the latter (Hamdi et al., Reference Hamdi, Brasier and Zhiwen1989). The Soltanieh Formation is replaced upwards into the overlying Barut Formation (Cambrian Age 3), which is a succession of greenish and reddish shale and siltstone alternating with dark dolomites and chert (Salehi, 1989).

Figure 1 Location of study area and general geology. (1) Map showing the location of the studied section. Insert shows the location of Fig. 1.2; (2), Geological map of Garmab, Tehran-Chalous road, north of Tehran, Iran (modified from Aghanabati et al., Reference Aghanabati, Mahmoudi and Bathaee2005). Upper Tuff Member (UTM), Asara Shale Member (ASM), Middle Tuff Member (MTM).
This study is mainly based on the well-exposed Garmab section, along the Tehran–Chalous road, 97 km northwest of Tehran (36.040900° N 51.310767° E, WGS84) (Fig. 1.1, 1.2), where a more diverse and abundant ichnofauna has been reported. At the Garmab section, the Lower Dolomite Member is a 60 m thick succession of yellowish brown-blue dolomite, the Lower Shale Member is a 192 m thick succession of black shale and silty shale, the Middle Dolomite Member is a 90 m thick succession of well bedded grayish-green dolomite, the Upper Shale Member is a 180 m thick succession of silty shale and sandstone, and the Upper Dolomite Member is a 600 m thick succession light blue dolomite.
Sedimentary facies and depositional environment
Eight facies have been recognized in Soltanieh Formation, which are grouped into siliciclastic-dominated and carbonate-dominated facies associations (Shahkarami et al., Reference Shahkarami, Mángano and Buatois2017). The siliciclastic-dominated Facies Association comprises Facies 1–4, whereas the carbonate-dominated Facies Association includes Facies 5–8.
Facies 1 consists of coarsening-upward cycles of interbedded greenish-gray silty shale and erosionally based, very fine- to medium-grained sandstone and sandy dolomite. This facies forms a discrete package in the uppermost part of the Upper Shale Member. It records deposition in the upper offshore, reflecting the alternation of suspension fallout during fair-weather times and storm deposition. Facies 2 consists of parallel-laminated, greenish-gray silty shale with mm-scale lenses and laterally discontinuous laminae of erosionally based, very fine-grained sandstone. This facies comprises most of the Upper Shale Member. It records deposition immediately above storm wave base, in the lower offshore. Facies 3 is characterized by parallel-laminated to massive, dark-gray shale and thinly bedded shale-siltstone alternations. This facies is present in the uppermost interval of the Lower Shale Member and the lowermost interval of the Upper Shale Member. It records deposition immediately below storm wave base in the proximal shelf. Facies 4 is characterized by laterally extensive, dark-gray parallel-laminated and massive shale. This facies constitutes most of the Lower Shale Member. It reflects deposition from suspension fallout in the distal shelf.
Facies 5 consists of laterally continuous, gray-to-blue dolomite with desiccation cracks, peloids, and fenestral structures. This facies comprises most of the Lower and Upper Dolomite members, and records deposition in the upper intertidal to supratidal zones. Facies 6 is characterized by well-bedded to massive, gray-to-blue, moderately sorted peloidal and intraclastic dolo-grainstone. This facies is present in the Middle Dolomite Member and records deposition in the intertidal to shallow subtidal zones. Facies 7 consists of parallel-laminated, current-rippled and low-angle cross-laminated limestone (packstone and wackestone) and intercalated dark-gray massive calcareous shale and silty shale. This facies occurs in the lowermost interval of the Lower Shale Member and records deposition in a shallow low-energy subtidal setting. Facies 8 consists of black phosphatic limestone with diverse SSF assemblages, this facies is absent in the Garmab section.
The carbonate-dominated facies association represents deposition in very shallow water (shallow subtidal to peritidal), whereas the siliciclastic-dominated facies association mostly comprises deposition below storm wave base (i.e., shelf), and immediately above storm wave base (i.e., lower and upper offshore) (see environmental subdivisions in Buatois and Mángano, Reference Buatois and Mángano2011).
Materials and methods
Repository and institutional abbreviation
The trace fossils described herein were all collected in the Garmab section, Soltanieh Formation, northern Iran. Ichnotaxa are listed alphabetically. Specimens are housed at the Royal Saskatchewan Museum, Regina, Canada.
Systematic paleontology
Ichnogenus Cochlichnus Hitchcock, Reference Hitchcock1858
Cochlichnus anguineus Hitchcock, Reference Hitchcock1858

Figure 2 Trace fossils from the Soltanieh Formation, Garmab section. (1) Cochlichnus anguineus from the Lower Shale Member (black arrows) (P3309.2) preserved as positive hyporelief; scale bar is 0.5 cm; (2) Curvolithus isp. from the Upper Shale Member (P3311.19) preserved as positive epirelief; scale bar is 0.25 cm; (3) Cruziana isp., from the Upper Shale Member (P3313.10) preserved as positive hyporelief; scale bar is 0.5 cm; (4) Didymaulichnus miettensis from the Upper Shale Member (P3313.8) preserved as positive hyporelief; scale bar is 1 cm; (5) Cruziana problematica from the Upper Shale Member (P3311.13) preserved as positive hyporelief; scale bar is 0.5 cm.
Materials
Four slabs (P3309.1, P3311.15, P3311.17, P3311.19) containing four specimens.
Description
Simple, small unbranched horizontal, sinusoidal trails. Trail width is 1.0–1.7 mm; maximum preserved length is 36.5 mm. Infill is massive. Preserved as positive hyporelief or negative epirelief.
Remarks
Cochlichnus anguineus Hitchcock, Reference Hitchcock1858 is the type ichnospecies of Cochlichnus, and differs from the two other Cochlichnus ichnospecies, C. annulatus Orłowski, Reference Orłowski1989, and C. antarcticus, Tasch, Reference Tasch1968, by lack of annulations or lateral markings and having the same filling as the host rock, respectively (Buatois and Mángano, Reference Buatois and Mángano1993a; Keighley and Pickerill, Reference Keighley and Pickerill1997). Cochlichnus is a grazing trail (pascichnion) produced by many different invertebrates, including annelids and nematodes (Fillion and Pickerill, Reference Fillion and Pickerill1990; Lucas et al., Reference Lucas, Lerner, Bruner and Shipman2010). It ranges from the Cambrian to Holocene (Mángano and Buatois, Reference Mángano and Buatois2014), and occurs in a great variety of marine and non-marine environments (Fillion and Pickerill, Reference Fillion and Pickerill1990).
Remarks
The ichnogenus Cruziana is mostly represented by a bilobate and elongated trail covered by transverse or herringbone-shaped ridges. Trails may be bounded by lateral outer zones with or without ridges (Häntzschel, Reference Häntzschel1975; Fillion and Pickerill, Reference Fillion and Pickerill1990). Cruziana comprises over 30 ichnospecies mostly separated based on morphologic features resulting from the leg morphology of the producer (Seilacher, Reference Seilacher1970, Reference Seilacher1992). Cruziana is best interpreted as related to combined locomotion and feeding strategy (pascichnia) (Seilacher, Reference Seilacher2007). It is widely accepted that the main producers of Cruziana in the marine Paleozoic were trilobites or trilobitomorphs (Seilacher, Reference Seilacher1970, Reference Seilacher1985, Reference Seilacher1992; Hofmann et al., Reference Hofmann, Mángano, Elicki and Shinaq2012). Cruziana ranges in age from early Cambrian to Holocene (Seilacher, Reference Seilacher1970; Muñiz Guinea et al., Reference Muñiz Guinea, Belaústegui, Cárcamo, Domènech and Martinell2015), and has been reported from a varied spectrum of paleoenvironments, ranging from typical shallow-water marine to freshwater settings (Bromley and Asgaard, Reference Bromley and Asgaard1979; Crimes, Reference Crimes1987).
Cruziana problematica Schindewolf, Reference Schindewolf1921
Materials
Two slabs (P3311.13, P3313.10) containing two specimens.
Description
Horizontal, gently winding, strongly convex, small, parallel-sided trails with transverse striation, and central groove dividing the trace longitudinally into two lobes. Width is 2.1–3.5 mm; maximum preserved length is 44.5 mm. Preserved as positive hyporelief.
Remarks
Relatively small, simple Cruziana with transverse to nearly transverse scratch marks are traditionally included in Cruziana problematica (e.g., Schindewolf, Reference Schindewolf1921; Bromley and Asgaard, Reference Bromley and Asgaard1979; Fillion and Pickerill, Reference Fillion and Pickerill1990). Jensen (Reference Jensen1997) regarded C. problematica as a junior synonym of Cruziana tenella (Linnarsson, Reference Linnarsson1871). However, C. problematica is widely reported and is considered the most stable ichnospecies (e.g., Mángano et al., Reference Mángano, Buatois, West and Maples2002b). The specimens documented here are very similar to C. tenella described by Jensen (Reference Jensen1997) from the Mickwitzia Sandstone, south-central Sweden and C. problematica described by Keighley and Pickerill (Reference Keighley and Pickerill1996) from eastern Canada. The name C. problematica is preferred here over C. tenella for reasons of nomenclatural stability (Mángano et al., Reference Mángano, Buatois, West and Maples2002b).
Materials
Five slabs (P3310.2, P3311.3, P3311.12, P3311.19, P3313.10) containing six specimens.
Description
Small, horizontal, slightly oblique, unbranched, trails with bilobate lower surface, and poorly preserved scratch marks. Width is 2.3–5.2 mm; maximum preserved length is 46.50 mm. Preserved as positive hyporelief and negative epirelief.
Remarks
Poor preservation does not allow determining the pattern of transverse striation, which therefore prevents ichnospecific assessment.
Occurrence
Lower and Upper Shale members.
Ichnogenus Curvolithus Fritsch, Reference Fritsch1908
Curvolithus isp.
Materials
Three slabs (P3311.19, P3317.2, P3319.1) containing four specimens.
Description
Horizontal, straight to slightly oblique, unbranched trails with trilobate upper surface consisting of two outer narrow lobes (each 1.7–2.6 mm wide) and one broad, flat central lobe (4.2–5.6 mm wide). The marginal outer lobes are discontinuous along the structure. Width of the trace is 6.8–10.8 mm; maximum preserved length is 89.0 mm. Preserved as positive epirelief and full relief.
Remarks
Curvolithus comprises two ichnospecies: Curvolithus multiplex and Curvolithus simplex (Buatois et al., Reference Buatois, Mángano, Mikuláš and Maples1998b). Curvolithus multiplex has a smooth, trilobate upper surface and a convex, quadra-lobate lower surface; in contrast to C. multiplex, C. simplex does not have a quadra-lobate lower surface. The specimens documented here are assigned to Curvolithus isp. due to uncertainties in the number of lobes on the lower surface. Curvolithus has been interpreted as the locomotion trace (Repichnia) of predators, including gastropods, flatworms, or nemerteans (Buatois et al., Reference Buatois, Mángano, Mikuláš and Maples1998b). Curvolithus ranges in age from Cambrian to Miocene and has been recorded from shallow-marine siliciclastic successions (Buatois et al., Reference Buatois, Mángano, Mikuláš and Maples1998b; Mángano and Buatois, Reference Mángano and Buatois2014).
Occurrence
Upper Shale Member.
Ichnogenus Didymaulichnus Young, Reference Young1972
Didymaulichnus miettensis Young, Reference Young1972
Materials
Four slabs (P3313.4, P3313.8, P3313.10, P3317.1) containing fourteen specimens.
Description
Horizontal, straight or curving bilobate trails. The trails have a shallow median groove, which divides the trail into two parts, and gently sloping peripheral discontinuous bevels. Overlap among specimens is common. Overall width is 15.2–20.5 mm. The median groove is 2.5–3.4 mm wide. The double-furrow is 10.2–12.7 mm wide. The outer bevels vary in width along the length of the trail, but where well preserved are 2.5–4.4 mm; maximum preserved length is 143.0 mm. Preserved as positive hyporelief and full relief.
Remarks
There are four accepted ichnospecies of Didymaulichnus: D. lyelli (Rouault, Reference Rouault1850); D. tirasensis Palij, Reference Palij1974; D. miettensis Young, Reference Young1972; and D. alternatus Pickerill, Romano, and Melendez, Reference Pickerill, Romano and Melendez1984. Didymaulichnus miettensis differs from other Didymaulichnus ichnospecies by the presence of lateral bevels (Young, Reference Young1972; Jensen and Mens, Reference Jensen and Mens2001). The specimens from the Soltanieh Formation closely resemble the type material of D. miettensis described by Young (Reference Young1972) from the upper Miette Group, western Canada. Didymaulichnus is interpreted as locomotion traces (Repichnia) of gastropods, bivalves, or arthropods (Hakes, Reference Hakes1977; Bradshaw, Reference Bradshaw1981), and ranges from Cambrian to Cretaceous (Vossler et al., Reference Vossler, Magwood and Pemberton1989). Didymaulichnus miettensis is restricted to early Cambrian (Jensen and Mens, Reference Jensen and Mens2001). A partially preserved specimen from the Soltanieh Formation illustrated by CiabeGhodsi (Reference CiabeGhodsi2007) was referred to Didymaulichnus miettensis.
Occurrence
Upper Shale Member.
Ichnogenus Gordia Emmons, Reference Emmons1844
Gordia marina Emmons, Reference Emmons1844
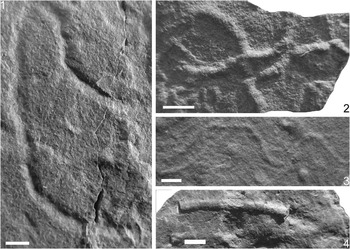
Figure 3 Trace fossils from the Soltanieh Formation, Garmab section. (1) Helminthoidichnites tenuis from Upper Shale Member (P3311.5) preserved as negative epirelief; scale bar is 0.25 cm; (2) Gordia marina from the Upper Shale Member (P3311.5) preserved as positive hyporelief; scale bar is 0.25 cm; (3) Helminthopsis tenuis from Upper Shale Member (P3311.18) preserved as positive hyporelief; scale bar is 0.25 cm; (4) Palaeophycus tubularis from the Upper Shale Member (P3319.3) preserved as positive hyporelief; scale bar is 0.5 cm.
Materials
One slab (P3311.5) containing a single specimen.
Description
Horizontal, non-branching, winding and curving, small trail with self-overcrossing. Width is 2.7 mm; maximum preserved length is 44.0 mm. Preserved as positive hyporelief.
Remarks
There are five recognized ichnospecies of Gordia: the type ichnospecies G. marina Emmons, Reference Emmons1844; G. arcuata Książkiewicz, Reference Książkiewicz1977; G. maeandria Jiang in Jiang, Luo, and Zhang, Reference Jiang, Luo and Zhang1982; G. nodosa Pickerill and Peel, Reference Pickerill and Peel1991; and G. indianaensis (Miller, Reference Miller1889). Gordia marina can be easily differentiated from the other ichnospecies of Gordia because it lacks the annulations of G. nodosa, the angled turns of G. indianaensis, the apical arcuate bends of G. arcuata, and the guided meanders of G. maeandria (Fillion and Pickerill, Reference Fillion and Pickerill1990; Buatois et al., Reference Buatois, Mángano, Maples and Lanier1998a). Fillion and Pickerill (Reference Fillion and Pickerill1990) questioned the validity of G. maeandria in that guided meander is not typical of Gordia. Gordia differs from Helminthopsis in its looped form, having overcrossing and never showing meanders (Pickerill and Peel, Reference Pickerill and Peel1991). The ethology of Gordia and its possible producers are not well constrained. Książkiewicz (Reference Książkiewicz1977) suggested that it might be a feeding burrow or trail produced by polychaetes. Yang (Reference Yang1984) considered it as a locomotion trace produced by worms or gastropods. Other authors (e.g., Aceñolaza and Buatois, Reference Aceñolaza and Buatois1993; McCann, Reference McCann1993; Geyer and Uchman, Reference Geyer and Uchman1995) have considered this ichnogenus as a pascichnia produced by worms or worm-like organisms. The ichnogenus Gordia ranges in age from Ediacaran to Holocene (Fillion and Pickerill Reference Fillion and Pickerill1990; Mángano and Buatois, Reference Mángano and Buatois2014), and is known from non-marine, and shallow- to deep-marine deposits (Narbonne, Reference Narbonne1984; Fillion and Pickerill, Reference Fillion and Pickerill1990; Norman, Reference Norman1996). The ichnospecies Gordia arcuata has been documented in the Soltanieh Formation by CiabeGhodsi (Reference CiabeGhodsi2007).
Occurrence
Upper Shale Member.
Ichnogenus Helminthoidichnites Fitch, Reference Fitch1850
Helminthoidichnites tenuis Fitch, Reference Fitch1850
Materials
Seven slabs (P3309.1, P3309.2, P3311.5, P3311.9, P3311.11, P3311.15, P3311.19) containing ten specimens.
Description
Simple, unbranched, horiztontal, mostly straight to slightly bent, nonmeandering trails. Diameter is 0.9–1.9 mm and may slightly vary along the course of individual trails. Maximum observed length is 83.8 mm. Overlapping among different individuals is common, but there is no self over crossing. Trace fill is identical to the host rock. Preserved as positive hyporelief and negative epirelief.
Remarks
Helminthoidichnites tenuis is interpreted as a grazing trace, most likely produced by vermiform animals (Buatois et al., Reference Buatois, Mángano, Maples and Lanier1998a). Helminthoidichnites comprises only one ichnospecies, H. tenuis, although the case may be made that a review of this ichnogenus may result in recognition of additional ichnospecies. Helminthoidichnites differs from Gordia by lacking self-overcrossing and from Helminthopsis by having a non-meandering course (Hofmann and Patel, Reference Hofmann and Patel1989; Buatois et al., Reference Buatois, Mángano, Maples and Lanier1998a). The ichnogenus ranges in age from Ediacaran to Holocene (Mángano and Buatois, Reference Mángano and Buatois2014).
Occurrence
Lower and Upper Shale members.
Ichnogenus Helminthopsis Heer, Reference Heer1877
Helminthopsis tenuis Książkiewicz, Reference Książkiewicz1968
Materials
Four slabs (P3309.1, P3309.2, P3311.17, P3311.18) containing eight specimens.
Description
Horizontal, smooth, unbranched, unlined, irregular, high-amplitude meandering trails. Width is 1.0–1.8 mm; maximum preserved length is 49.0 mm. Preserved as positive hyporelief and negative epirelief.
Remarks
There are three ichnospecies of Helminthopsis, separated based on their geometrical pattern (Wetzel and Bromley, Reference Wetzel and Bromley1996): H. abeli Książkiewicz, Reference Książkiewicz1977; H. hieroglyphica Wetzel and Bromley, Reference Wetzel and Bromley1996; and H. tenuis Książkiewicz, Reference Książkiewicz1968. Helminthopsis tenuis is distinguished from H. abeli and H. hieroglyphica by the lack of horseshoe-like turns and its high-amplitude winding (Wetzel and Bromley, Reference Wetzel and Bromley1996), and from Helminthoidichnites by its meandering course (Hofmann and Patel, Reference Hofmann and Patel1989). Helminthopsis is thought to be a grazing trace (pascichnion) produced by deposit-feeding organisms in brackish to fully marine environments; polychaete annelids are regarded as potential tracemakers (Ksiazkiewicz, 1977). Helminthopsis ranges in age from Ediacaran to Holocene (Buatois et al., Reference Buatois, Mángano, Maples and Lanier1998a).
Occurrence
Lower and Upper Shale members.
Ichnogenus Palaeophycus Hall, Reference Hall1847
Palaeophycus tubularis Hall, Reference Hall1847
Materials
Seventeen slabs (P3311.1, P3311.4, P3311.7, P3311.10, P3311.12, P3311.14, P3311.19, P3311.20, P3312.1, P3313.1, P3313.3, P3313.9, P3313.10, P3314.1, P3315.1, P3316.1, P3318.1) containing thirty five specimens.
Description
Horizontal, branched and unbranched, straight to slightly curved, unornamented, thinly lined cylindrical burrows. Burrow-fill is similar to the host rock. Width is 2.6–13.4 mm; maximum preserved length is 172.5 mm. Preserved as positive hyporelief.
Remarks
Palaeophycus is distinguished from Planolites primarily by the presence of wall linings and a burrow-fill identical to the host rock. Infills of Palaeophycus represent passive, gravity-induced sedimentation within open, lined burrows; collapse features show that some segments were incompletely filled by this process. The fillings, therefore, tend to be of the same composition as the surrounding matrix (Pemberton and Frey, Reference Pemberton and Frey1982). Seven ichnospecies of Palaeophycus are currently accepted: P. tubularis Hall, Reference Hall1847; P. heberti (Saporta, Reference Saporta1872); P. striatus Hall, Reference Hall1852; P. sulcatus (Miller and Dyer, Reference Miller and Dyer1878); P. alternatus Pemberton and Frey, Reference Pemberton and Frey1982; P. bolbitermilus Kim, Pickerill, and Wilson, Reference Kim, Pickerill and Wilson2000; and P. imbricatus (Torell, Reference Torell1870). Palaeophycus tubularis is distinguished from the other Palaeophycus ichnospecies by its thin wall and the absence of striations (Pemberton and Frey, Reference Pemberton and Frey1982). Palaeophycus is interpreted as dwelling burrows (dominichnia) of suspension feeders or predators, such as polychaetes (Osgood, Reference Osgood1970; Pemberton and Frey, Reference Pemberton and Frey1982), and ranges in age from Ediacaran to Holocene (Häntzschel, Reference Häntzschel1975). Palaeophycus is a facies-crossing ichnotaxon found in almost every depositional environment (Pemberton and Frey, Reference Pemberton and Frey1982).
Occurrence
Lower and Upper Shale members.
Ichnogenus Phycodes Richter, Reference Richter1850
Phycodes isp.

Figure 4 Trace fossils from the Soltanieh Formation, Garmab section. (1) Psammichnites gigas from the Upper Shale Member (P3317.1) preserved as positive epirelief; scale bar is 0.5 cm; (2) Rusophycus avalonensis from the Upper Shale Member (P3313.9) preserved as positive hyporelief; scale bar is 0.25 cm; (3) Planolites montanus from the Upper Shale Member (P3313.8) preserved as positive hyporelief; scale bar is 0.25 cm; (4) Phycodes isp. from the Upper Shale Member (P3319.3) preserved as full relief; scale bar is 0.5 cm.
Materials
Two slabs (P3311.1, P3319.3) containing two specimens.
Description
Horizontal, broomlike structure without spreite, consisting of four branches originated from the same point of a thick, slightly curved single stem. Width of the branches is 4.2–8.2 mm, whereas the main tube is 7.1–9.0 mm in diameter. Maximum length preserved is 79.9 mm. Preserved as full relief.
Remarks
Thirteen ichnospecies of Phycodes have been described in the literature, based on their overall pattern and size, nature, style, disposition and degree of branching, and presence or absence of spreite (Han and Pickerill, Reference Han and Pickerill1994): P. antecedens Webby, Reference Webby1970; P. auduni Dam, Reference Dam1990; P. bromleyi Dam, Reference Dam1990; P. circinatum Richter, Reference Richter1853; P. coronatum Crimes and Anderson, Reference Crimes and Anderson1985; P. curvipalmatum Pollard, Reference Pollard1981; P. flabellum (Miller and Dyer, Reference Miller and Dyer1878); P. fusiforme Seilacher, Reference Seilacher2000; P. templus Han and Pickerill, Reference Han and Pickerill1994; P. parallelum Seilacher, Reference Seilacher2000; P. reniforme Hofmann, Reference Hofmann1979; P. ungulatus Fillion and Pickerill, Reference Fillion and Pickerill1990; and P. wabanensis Fillion and Pickerill, Reference Fillion and Pickerill1990. Some forms of Phycodes consist of a few main branches showing a spreite-like structure, which distally give rise to numerous free branches. In other forms, the spreite are lacking, and branches tend to be more random (Osgood, Reference Osgood1970). Phycodes reflects a variety of behavioral activities by the tracemaker (Han and Pickerill, Reference Han and Pickerill1994), but two basic interpretations are a feeding trace (fodinichnion) produced by an organism that systematically mines a nutrient-rich layer along a silt-mud surface, most likely annelids (Seilacher, Reference Seilacher1955), or a structure produced by an organism that burrowed outwards from a single point and then withdrew to a ‘home-case’ only to re-burrow outwards again through the previously excavated tunnel (Marintsch and Finks, Reference Marintsch and Finks1982; Singh et al., Reference Singh, Tovar and Ibotombi2008). Phycodes ranges in age from Cambrian (Crimes and Anderson, Reference Crimes and Anderson1985) to Miocene (Bradley, Reference Bradley1981). Phycodes has been reported from shallow-marine, deep-marine (Crimes et al., Reference Crimes, Legg, Marcos and Arboleya1977; Bradley, Reference Bradley1981; Narbonne, Reference Narbonne1984), and brackish-water (Hakes, Reference Hakes1985) environments. Non-marine examples are in need of re-evaluation (Pollard, Reference Pollard1985). Although the Soltanieh specimen supports placement in Phycodes, poor preservation prevents an ichnospecific assessment.
Occurrence
Upper Shale Member.
Ichnogenus Planolites Nicholson, Reference Nicholson1873
Planolites montanus Richter, Reference Richter1937
Materials
Five slabs (P3311.8, P3311.11, P3311.17, P3311.19, P3313.8) containing thirteen specimens.
Description
Horizontal, unbranched, straight to slightly curved, unornamented, cylindrical burrows. Burrow-fill similar to the host rock. Width is 0.6–2.8 mm; maximum preserved length is 47.1 mm. Preserved as positive and negative hyporelief.
Remarks
Four ichnospecies of Planolites are currently recognized based on size, curvature, and wall characteristics: P. beverleyensis (Billings, Reference Billings1862); P. annularis Walcott, Reference Walcott1890; P. montanus Richter, Reference Richter1937; and P. constriannulatus Stanley and Pickerill, Reference Stanley and Pickerill1994. Planolites montanus comprises small, curved to tortuous burrows. Planolites beverleyensis comprises large, straight to gently curved burrows. Planolites annularis consists of transversely annulated burrows, and Planolites constriannulatus is comprised of a burrow with both longitudinal striations and transverse annulation. Planolites is interpreted as feeding burrows (fodinichnia) of deposit-feeders (Pemberton and Frey, Reference Pemberton and Frey1982), and ranges in age from Cambrian to Holocene (Häntzschel, Reference Häntzschel1975; Mángano and Buatois, Reference Mángano and Buatois2014). Planolites is found in almost every depositional environment (Pemberton and Frey, Reference Pemberton and Frey1982).
Occurrence
Upper Shale Member.
Ichnogenus Psammichnites Torell, Reference Torell1870
Psammichnites gigas (Torell, Reference Torell1868)
Materials
Six slabs (P3313.2, P3313.5, P3313.6, P3313.7, P3317.1, P3317.2) containing fifteen specimens.
Description
Large, unbranched, horizontal, straight to slightly curved bilobate trails. The burrow-fill is finer-grained and darker in color compared to the host sediment. Width is 6.7–20.0 mm; maximum length preserved is 148.8 mm. Overlap among specimens is locally common. Preserved as negative hyporelief and positive epirelief. Negative hyporeliefs are gently concave, with a median ridge. Positive epireliefs are gently convex with a shallow, straight median groove of variable width and depth.
Remarks
There is general agreement that the ichnogenus Olivellites Fenton and Fenton, Reference Fenton and Fenton1937 is a junior synonym of Psammichnites (Chamberlain, Reference Chamberlain1971; D’Alessandro and Bromley, Reference D’Alessandro and Bromley1987; Maples and Suttner, Reference Maples and Suttner1990; Seilacher, Reference Seilacher1997; Zhu, Reference Zhu1997; Mángano et al., Reference Mángano, Buatois and Rindsberg2002a). The ichnotaxonomic status of the lower Cambrian ichnospecies Taphrhelminthopsis circularis Crimes et al., Reference Crimes, Legg, Marcos and Arboleya1977 is more uncertain. Uchman (Reference Uchman1995a) demonstrated that the ichnogenus Taphrhelminthopsis is a preservational variant of Scolicia and, therefore, its junior synonym. Taphrhelminthopsis circularis is most likely a preservational variant of Psammichnites (Mángano and Buatois, Reference Mángano and Buatois2016). Psammichnites is distinguished from Didymaulichnus by lack of median groove on the lower side and by its overall more complex internal structure (Seilacher, Reference Seilacher2007). Psammichnites gigas Torell, Reference Torell1870 is the type ichnospecies of Psammichnites; other ichnospecies include P. plummeri (Fenton and Fenton, Reference Fenton and Fenton1937); P. grumula (Romano and Meléndez, Reference Romano and Meléndez1979); P. implexus (Rindsberg, Reference Rindsberg1994); and P. saltensis (Aceñolaza and Durand, Reference Aceñolaza and Durand1973). The Soltanieh specimens are very similar in overall shape and size to Psammichnites gigas, as described and illustrated by Hofmann and Patel (Reference Hofmann and Patel1989) from the lower Cambrian of New Brunswick, Canada. Psammichnites is interpreted as a back-filled structure representing the feeding activities of a subsurface vagile animal using a siphon-like device (Mángano et al., Reference Mángano, Buatois and Rindsberg2002a; Jago and Gatehouse, Reference Jago and Gatehouse2007). The ichnogenus Psammichnites ranges in age from early Cambrian to Permian (Mángano et al., Reference Mángano, Buatois and Rindsberg2002a), and the type ichnospecies, Psammichnites gigas, is of early Cambrian age (Jago and Gatehouse, Reference Jago and Gatehouse2007). Psammichnites gigas was first recorded in the Soltanieh Formation by CiabeGhodsi (Reference CiabeGhodsi2007).
Occurrence
Upper Shale Member.
Ichnogenus Rusophycus Hall, Reference Hall1852
Rusophycus avalonensis Crimes and Anderson, Reference Crimes and Anderson1985
Materials
One slab containing one specimen (P3313.9).
Description
Short, horizontal, bilobate trace consisting of few scratch marks (6–7) arranged transversely to the median furrow. Width is 23.8 mm; maximum length preserved is 17.6 mm. Preserved as positive hyporelief.
Remarks
Rusophycus comprises several ichnospecies, including 21 listed just for the lower Cambrian (Mángano and Buatois, Reference Mángano and Buatois2016); its type ichnospecies is Rusophycus biloba (Fillion and Pickerill, Reference Fillion and Pickerill1990). Rusophycus is distinguished from Cruziana by its length-to-width ratio; only specimens with the length-to-width ratio less than two are placed in Rusophycus (Keighley and Pickerill, Reference Keighley and Pickerill1996). The specimens analyzed here are similar to Rusophycus avalonensis Crimes and Anderson, Reference Crimes and Anderson1985 because of the overall shape and the divided ridges, but the ridges are not arranged in bundles. Paleozoic marine Rusophycus are widely accepted as resting traces (cubichnia) made by trilobites (Osgood, Reference Osgood1970; Crimes, Reference Crimes1975).
Remarks
Treptichnus is a burrow consisting of segments connected at their ends, each one to the next, characteristically but not invariably in a zigzag pattern. At present, there are ten ichnospecies of Treptichnus: the type ichnospecies, T. bifurcus Miller, Reference Miller1889; T. pedum (Seilacher, Reference Seilacher1955); T. triplex Palij, Reference Palij1976; T. coronatum (Crimes and Anderson, Reference Crimes and Anderson1985); T. lublinensis Paczesna, Reference Paczesna1986; T. pollardi Buatois and Mángano, Reference Buatois and Mángano1993b; T. tripleurum (Geyer and Uchman, Reference Geyer and Uchman1995); T. rectangularis Orłowski and Zylińska, Reference Orłowski and Zylińska1996; T. meandrinus Uchman, Bromley, and Leszczyński, Reference Uchman, Bromley and Leszczyński1998; and T. apsorum Rindsberg and Kopaska-Merkel, Reference Rindsberg and Kopaska-Merkel2005. Treptichnus is interpreted as feeding structures (fodinichnia) produced by vermiform animals or insect larvae, the latter in the case of non-marine occurrences (Uchman, Reference Uchman2005). Treptichnus has been recorded from non-marine (Buatois and Mángano, Reference Buatois and Mángano1993a, Reference Buatois and Mángano1993b; Buatois et al., Reference Buatois, Mángano, Fregenal-Martinez and Gibert2000), marginal-marine (Archer and Maples, Reference Archer and Maples1984; Buatois et al., Reference Buatois, Mángano, Maples and Lanier1998a), shallow-marine (Fedonkin, Reference Fedonkin1977; Geyer and Uchman, Reference Geyer and Uchman1995), and deep-marine (Crimes et al., Reference Crimes, Goldring, Homewood, Van Stuijvenberg and Winkler1981; Uchman et al., Reference Uchman, Bromley and Leszczyński1998) environments. Treptichnus is considered to range in age from Cambrian to Holocene (Crimes, Reference Crimes1987; Geyer and Uchman, Reference Geyer and Uchman1995; Muñiz-Guinea et al., 2014). However, reports of treptichnid trace fossils from terminal Ediacaran rocks in the Nama Group of Namibia and the GSSP section in Newfoundland suggest that Treptichnus may extend back into the late Ediacaran (Jensen et al., Reference Jensen, Saylor, Gehling and Germs2000; Gehling et al., Reference Gehling, Jensen, Droser, Myrow and Narbonne2001; Högström et al., Reference Högström, Jensen, Palacios and Ebbestad2013). Further work on the relationship between the so-called treptichnids and Treptichnus is pending.
Treptichnus pedum (Seilacher, Reference Seilacher1955)

Figure 5 Trace fossils from the Soltanieh Formation, Garmab section. (1) Treptichnus pedum from the Upper Shale Member (P3311.11); scale bar is 0.25 cm; (2) Treptichnus isp. from the Upper Shale Member (P3319.2); scale bar is 0.5 cm; (3) Treptichnus pollardi from the Upper Shale Member (P3311.20); scale bar is 0.5 cm. All specimens preserved as positive hyporelief.
Materials
Five slabs (P3310.2, P3311.2, P3311.8, P3311.11, P3311.15,) containing nine specimens.
Description
Straight or curved sets of individual burrows of similar length connected to one another at their lower parts. The burrows alternate in direction, forming a zigzag pattern; where the burrows are arranged in a nearly straight succession, the segments generally are aligned, and the zigzag pattern is rarely developed. In curved portions of the burrow, the segments generally project outwards. Width is 2.9–6.7 mm; maximum preserved length is 25.2 mm. Preserved as positive hyporelief.
Remarks
Treptichnus pedum was originally described as Phycodes pedum, but Osgood (Reference Osgood1970) noted that P. pedum differs from other ichnospecies of Phycodes, such as the type ichnospecies Phycodes circinatum Richter, Reference Richter1853, and that it merited a new ichnogeneric designation. Treptichnus pedum, as described by Seilacher (Reference Seilacher2007), includes straight, sinusoidal, curved, or looping burrows constructed from upward curving segments. Treptichnus pedum is a feeding burrow (Crimes et al., Reference Crimes, Legg, Marcos and Arboleya1977) with several morphological variants (Seilacher, Reference Seilacher2007), ranges in age from the early Cambrian to Holocene (Crimes et al., Reference Crimes, Legg, Marcos and Arboleya1977; Muñiz Guinea et al., Reference Muñiz Guinea, Mángano, Buatois, Podeniene, Vintaned and Alfaro2014), and is restricted to normal-marine salinity conditions (Buatois et al., Reference Buatois, Almond and Germs2013). There is general agreement that the T. pedum tracemaker was a motile bilaterian animal that lived below the sediment-water interface, propelling itself forward in upward curving projections that breached the sediment surface (Seilacher, Reference Seilacher1955; Geyer and Uchman, Reference Geyer and Uchman1995; Jensen, Reference Jensen1997; Dzik, Reference Dzik2005). Dzik (Reference Dzik2005) argued that the T. pedum animal was a priapulid worm, a conclusion supported by Vannier et al. (Reference Vannier, Calandra, Gaillard and Żylińska2010) based on neoichnologic experiments. Although it is sometimes referred to as Trichophycus pedum (e.g., Geyer and Uchman, Reference Geyer and Uchman1995; Peng et al., Reference Peng, Babcock and Cooper2012a), the overall morphology of Trichophycus is remarkably different, consisting of a U-shaped burrow with a retrusive spreite (Mángano and Buatois, Reference Mángano and Buatois2011). Treptichnus pedum has been reported as Trichophycus pedum from the Soltanieh Formation by CiabeGhodsi et al. (Reference CiabeGhodsi, Hamdi, Abdi and Sadeghi2006) and CiabeGhodsi (Reference CiabeGhodsi2007).
Occurrence
Lower and Upper Shale members.
Treptichnus pollardi Buatois and Mángano, Reference Buatois and Mángano1993b
Materials
Six slabs (P3318.1, P3311.6, P3311.16, P3311.18, P3311.20, P3313.01) containing ten specimens.
Description
Horizontal, simple, straight burrows with knots or shafts at semi-regular intervals. Width is 1.3–4.6 mm; individual segments length is 2.6 mm. Spacing between segments is 7.5–15.9 mm. The maximum number of burrow segments is six. Preserved as positive hyporelief.
Remarks
Treptichnus pollardi is distinguished from T. bifurcus by the presence of surficial pits, absence of twiglike projections, more irregular pattern, and longer individual segments. Treptichnus pollard differs from T. lublinensis and T. triplex by the absence of terminations projecting past the zigzags, the presence of pits associated with the horizontal burrow segment, and its thinner segments (Buatois and Mángano, Reference Buatois and Mángano1993b). Although distinction from the ichnogenera Saerichnites and Ctenopholeus may be unclear depending on preservation (e.g., Buatois and Mángano, Reference Buatois and Mángano2004; Fürsich et al., Reference Fürsich, Wilmsen and Seyed-Emami2006), the overall morphology of the Soltanieh Formation favors inclusion in T. pollardi. Treptichnus pollardi has been typically, though not exclusively (e.g., Geyer and Uchman, Reference Geyer and Uchman1995), recorded from freshwater environments (Buatois and Mángano, Reference Buatois and Mángano1993a, Reference Buatois and Mángano1993b; Fregenal-Martinez et al., Reference Fregenal-Martinez, Buatois and Mángano1995; Metz, Reference Metz1995; Buatois et al., Reference Buatois, Mángano, Fregenal-Martinez and Gibert2000).
Materials
Four slabs (P3310.1, P3310.2, P3311.9, P3319.2) containing seven specimens.
Description
Horizontal, gently curved, branching burrows. The burrows occasionally exhibit a short projection. Width is 1.1–2.6 mm. Preserved as positive hyporelief.
Remarks
Lack of zigzag pattern and regular projection does not allow ichnospecific assignment.
Occurrence
Upper Shale Member.
Previous work
In order to contextualize the new trace-fossil data reported here and to define a biozonation for the Soltanieh Formation, previously published reports of trace fossils and SSFs from this unit are briefly reviewed and critically re-evaluated.
Lower Dolomite Member
Hamdi et al. (Reference Hamdi, Brasier and Zhiwen1989) reported phosphatic tubes, including Hyolithellus sp. along with others resembling Rugatotheca sp., fragments of the protoconodont Protohertzina sp., and globomorphs of the Olivooides multisulcatus Qian, Reference Qian1977 group in the upper part of the Lower Dolomite Member in the Vali-Abad area.
Lower Shale Member
Hamdi et al. (Reference Hamdi, Brasier and Zhiwen1989) and CiabeGhodsi (Reference CiabeGhodsi2007) recorded large discoidal algal vesicles assigned to Chuaria sp. from the lower interval of the Lower Shale Member in the Vali-Abad area, and the type section, respectively. Hamdi et al. (Reference Hamdi, Brasier and Zhiwen1989) compared their material with larger ellipsoidal vesicles of Shouhsienia sp. from the Sinian System in China. CiabeGhodsi (Reference CiabeGhodsi2007) reported Diplocraterion isp., Planolites vulgaris, Skolithos isp., and Treptichnus pedum from the upper interval of the Lower Shale Member. Planolites vulgaris needs re-evaluation because the type specimen of this ichnospecies has been regarded as inorganic (Pemberton and Frey, Reference Pemberton and Frey1982). Based on the illustration provided, an affinity with Helminthoidichnites cannot be disregarded. In addition, specimens attributed to Diplocraterion isp. and Skolithos isp. are based on bedding-plane expressions, so their true morphology cannot be confirmed.
Middle Dolomite Member
Hamdi et al. (Reference Hamdi, Brasier and Zhiwen1989) reported the tubular fossil Hyolithellus vladimirovae and protoconodonts of the Protohertzina anabarica Missarzhevsky, Reference Missarzhevsky1969 group from the lower interval of the Middle Dolomite Member. Less common elements include the tubular fossils Anabarites trisulcatus Missarzhevsky in Voronova and Missarzhevsky, Reference Voronova and Missarzhevsky1969; Cambrotubulus decurvatus Missarzhevsky in Rozanov et al., Reference Rozanov, Missarzhevsky, Volkova, Voronova, Krylov, Keller, Korolyuk, Lendzion, Michniak, Pykhova and Sidorov1969; siphogonuchitids; Palaeosulcachites sp.; Siphogonuchites sp.; and globomorphs. These authors mentioned that beds near the top of the Middle Dolomite Member contain a similar assemblage, but with more abundant A. trisulcatus, C. decurvatus, and Siphogonuchites sp., in addition to the primitive mollusks Maikhanella multa and Purella sp., and the tubular fossils Tiksitheca licis Missarzhevsky in Rozanov et al., Reference Rozanov, Missarzhevsky, Volkova, Voronova, Krylov, Keller, Korolyuk, Lendzion, Michniak, Pykhova and Sidorov1969; Circotheca sp.; and Ladatheca isp.; as well as hyolithids. Tashayoee et al. (Reference Tashayoee, Hamdi, Vaziri and Yousofzadeh2012) recently reported Anabarites latus Val’kov and Sysoev, Reference Val’kov and Sysoev1970; A. rectus Vasil’eva in Rudavskaya and Vasil’eva, 1984; A. tripartitus Missarzhevsky in Rozanov et al., Reference Rozanov, Missarzhevsky, Volkova, Voronova, Krylov, Keller, Korolyuk, Lendzion, Michniak, Pykhova and Sidorov1969; A. trisulcatus; Cambrotublus isp.; Conotheca subcurvata Yu, Reference Yu1974; Drepanochites dilatatus Qian and Jiang in Luo et al., Reference Luo, Jiang, Wu, Song and Ou1982; Hyolithellus vladimirovae Missarzhevsky in Rozanov and Missarzhevsky, Reference Rozanov and Missarzhevsky1966; Jakutiochrea lenta Val’kov, Reference Val’kov1987; Protohertzina anabarica Missarzhevsky, Reference Missarzhevsky1969; P. siciformis Missarzhevsky, Reference Missarzhevsky1973; P. unguliformis Missarzhevsky, Reference Missarzhevsky1973; Siphogonuchites triangularis Qian, Reference Qian1977; Siphogonuchites sp.; and Yunnanodus dolerus Wang and Jiang in Jiang, Reference Jiang.1980 from the Garmab section. CiabeGhodsi (Reference CiabeGhodsi2007) documented the ichnotaxa Bergaueria perata Prantl, Reference Prantl1945; Circulichnus montanus (Vyalov, Reference Vyalov1971); and Gordia arcuata from the lower interval of the Middle Dolomite Member at the type section. However, specimens assigned to Bergaueria perata and Circulichnus montanus are unconvincing based on the available material.
Upper Shale Member
Hamdi et al. (Reference Hamdi, Brasier and Zhiwen1989) mentioned that at the Vali-Abad section the lower interval of the Upper Shale Member contains abundant and diverse phosphatized mollusks comparable with those found in the upper interval of the Middle Dolomite Member. These authors also reported Anabarites cf. trisulcatus Missarzhevsky, Reference Missarzhevsky1969; allathecidae hyoliths; and pelagiellids from the Dalir section. The upper interval in the Vali-Abad section contains specimens of the Latouchella korobkovi (Vostokova, Reference Vostokova1962) group of monoplacophorans, including ‘close-coiled’ Yangtzespira sp., ‘lax coiled’ Bemella sp., ‘uncoiled’ Ceratoconus sp., and Obtusoconus sp., which appear approximately 20 m from the top of the unit. Other typical elements at this level include Purella tianzhushanensis Yu, Reference Yu1979 and broad monoplacophorans resembling Protowenella sp.; pelagiellids of the Pelagiella lorenzi (Kobayashi, Reference Kobayashi1939) occur in the top 10 m. CiabeGhodsi (Reference CiabeGhodsi2007) added Lopochites latazonalis Qian, Reference Qian1977; Igorella emeiensis (Yu, Reference Yu1987); Igorella sp.; Purella squamulosa Qian and Bengtson, Reference Qian and Bengtson1989; Bemella simplex Yu, Reference Yu1979; and Lapworthella sp. to this group. CiabeGhodsi (Reference CiabeGhodsi2007) described several trace fossils from the Garmab section, namely Chondrites furcatus Sternberg, Reference Sternberg1833; Didymaulichnus miettensis; Diplichnites isp.; Diplocraterion isp; Hormosiroidea isp.?; Monomorphichnus lineatus Crimes et al., Reference Crimes, Legg, Marcos and Arboleya1977; Neonereites uniserialis Seilacher, Reference Seilacher1960; Palaeophycus alternatus; Paleodictyon croaticum Uchman, Reference Uchman1995b; Plagiogmus arcuatus Glaessner, Reference Glaessner1969; Psammichnites gigas; and Protovirgularia dichotoma? M’Coy, Reference M’Coy1850. However, many of these ichnotaxa lack diagnostic features and have been excluded from the list of trace fossils used in this study. Structures assigned to Chondrites furcatus do not display the classic dichotomic branching of this ichnogenus (Fu, Reference Fu1991). The specimen attributed to Diplichnites isp. appears to consist of a string of pits rather than appendage imprints and in fact, a second string of pits is apparent on the same slab, which may suggest an affinity with Saerichnites. The specimen attributed to Diplocraterion is only seen on bedding-plane view, so their U-shaped morphology cannot be confirmed. The ichnotaxonomy of Hormosiroidea is in need of revision (Gaillard and Olivero, Reference Gaillard and Olivero2009). In addition, the specimen illustrated lacks the morphologic features typically present in this ichnogenus. Based on the illustrations provided, the presence of scratch marks cannot be confirmed in the specimens assigned to Monomorphichnus lineatus. Neonereites uniserialis has been regarded as a preservation variant of Nereites (Uchman, Reference Uchman1995a). However, the specimen illustrated from the Soltanieh Formation lacks the diagnostic features of Nereites, actually resembling a simple grazing trail, such as Helminthoidichnites. The specimen figured as Paleodictyon croaticum does not display the typical morphology of this ichnotaxon; an affinity with Multina cannot be disregarded. Plagiogmus arcuatus is now considered a preservational variant of Psammichnites gigas by some (McIlroy and Heys, Reference McIlroy and Heys1997; Mángano et al., Reference Mángano, Buatois and Rindsberg2002a). The structures figured as Protovirgularia dichotoma? do not show the diagnostic chevronate pattern characteristic of Protovirgularia (e.g., Seilacher and Seilacher, Reference Seilacher and Seilacher1994; Mángano et al., Reference Mángano, Buatois, West and Maples1998).
Upper Dolomite Member
Meyer (Reference Meyer1967) noted that the stromatolites Collenia spissa Fenton and Fenton, Reference Fenton and Fenton1939 and Hadrophycus immanis Fenton and Fenton, Reference Fenton and Fenton1939 are moderately common in the upper interval of this member. Problematic records of Salterella have also been reported (Assereto, Reference Assereto1963; Stöcklin et al., Reference Stöcklin, Ruttner and Nabavi1964). CiabeGhodsi (Reference CiabeGhodsi2007) and Tashayoee et al. (Reference Tashayoee, Hamdi, Vaziri and Yousofzadeh2012) also reported Hyolithellus filiformis Bengtson in Bengtson et al., Reference Bengtson, Conway Morris, Cooper, Jell and Runnegar1990; Conotheca subcurvata (Yu, Reference Yu1974); Igorella sp.; Latouchella krobkovi Vostokova, Reference Vostokova1962; Latouchella maidipingensis (Yu, Reference Yu1974); and Obtusoconus rostriptutea Qian in Qian et al., 1978 from this unit.
Ichnostratigraphy of the Soltanieh Formation
Several ichnostratigraphic schemes have been proposed for the Ediacaran-Cambrian boundary (Alpert, Reference Alpert1977; Crimes, Reference Crimes1987, Reference Crimes1992; MacNaughton and Narbonne, Reference MacNaughton and Narbonne1999; Jensen, Reference Jensen2003). The latter two schemes have been recently adapted (Buatois and Mángano, Reference Buatois and Mángano2011; Mángano et al., Reference Mángano, Buatois and MacNaughton2012), and two Ediacaran zones and three early Cambrian zones are considered. The lower Ediacaran zone includes simple grazing trails, such as Helminthoidichnites, Helminthopsis, Gordia, and Archaeonassa, together with Epibaion and Kimberichnus. The age of this interval is ca. 560–550 Myr (Jensen et al., Reference Jensen, Droser and Gehling2006). The upper Ediacaran zone includes the oldest branching-burrow systems, represented by treptichnids. The age of this zone is 550–541 Myr (Grotzinger et al., Reference Grotzinger, Bowring, Saylor and Kaufman1995; Jensen et al., Reference Jensen, Droser and Gehling2006; Schmitz, Reference Schmitz2012). The lowermost early Cambrian zone, or the Treptichnus pedum Zone, is of Fortunian age and defined by the first appearance of T. pedum, as well as other complex forms (Narbonne et al., Reference Narbonne, Myrow, Landing and Anderson1987; Landing et al., Reference Landing, Geyer, Brasier and Bowring2013, Reference Landing, Geyer and Buatois2015). The Rusophycus avalonensis Zone contains the oldest bilobate, trilobite-type resting traces (R. avalonensis) and the bilobate epichnial trail Taphrelminthopsis circularis (Narbonne et al., Reference Narbonne, Myrow, Landing and Anderson1987; Jensen et al., Reference Jensen, Droser and Gehling2006). The age of this zone ranges from Fortunian to Cambrian Age 2. The Cruziana problematica Zone (= C. tenella Zone), which contains the oldest bilobate, trilobite-like trails (Cruziana problematica) associated with Psammichnites gigas, is Cambrian Age 2.
The application of the lowest appearance of SSFs for global correlation of Ediacaran-Cambrian boundary was first proposed at the Bristol plenary session in 1983 (Narbonne et al., Reference Narbonne, Myrow, Landing and Anderson1987). Biozonations based on SSFs are available in Siberia and South China (Peng et al., Reference Peng, Babcock and Cooper2012a). In Siberia, SSF zonation comprises, in ascending order, the Anabarites trisulcatus and Purella antiqua assemblage zones of the Fortunian, and Watsonella crosbyi and Aldanella operosa assemblage zones of Cambrian Age 2–Age 3 (Khomentovsky and Karlova, Reference Khomentovsky and Karlova1993, Reference Khomentovsky and Karlova2002; Peng et al., Reference Peng, Babcock and Cooper2012a). The SSF zonation of south China comprises the assemblage zones Anabarites trisulcatus-Protohertzina anabarica (SSF1) of the lower Meishucunian Stage (= Fortunian), Paragloborilus subglobosus-Purella squamulosa (SSF2), and Watsonella crosbyi (SSF3) of the middle Meishucunian Stage (= lower part of Cambrian Age 2). The upper Meishucunian (= upper part of Cambrian Age 2) strata comprise the Sinosachites flabelliformis-Tannuolina zhangwentangi Assemblage Zone (SSF4) (Steiner et al., Reference Steiner, Li, Qian, Zhu and Erdtmann2007), which directly underlies the trilobitic interval of the Cambrian in the shallow-water realm of the Yangtze Platform.
Non-biostratigraphic techniques used for correlation of the Ediacaran-Cambrian boundary include chemostratigraphic techniques, such as the measurement of carbon and sulfur isotopes (Magaritz et al., Reference Magaritz, Holser and Kirschvink1986; Tucker, Reference Tucker1986; Magaritz, Reference Magaritz1989; Brasier et al., Reference Brasier, Anderson and Corfield1992; Brasier, Reference Brasier1993; Shen and Schidlowski, Reference Shen and Schidlowski2000; Ru et al., Reference Ru, ShengHui and XueLei2011). The carbon isotopic curve emerges as an increasingly important tool for intercontinental and intracontinental correlation, especially in regions where the primary biologic marker for a key horizon is absent. The results obtained by applying chemostratigraphic techniques, however, may be influenced by provenance and diagenetic alteration, and most importantly they still require biostratigraphy for final calibration (Rozanov et al., Reference Rozanov, Khomentovsky, Shabanov, Karlova, Varlamov, Luchinina, Demidenko, Parkhaev, Korovnikov and Skorlotova2008). In any case, biostratigraphy remains a cost-effective means of correlating the Ediacaran–Cambrian transition.
The biostratigraphy of the Soltanieh Formation is poorly known and largely based on shelly fossils (Hamdi et al., Reference Hamdi, Brasier and Zhiwen1989; Tashayoee et al., Reference Tashayoee, Hamdi, Vaziri and Yousofzadeh2012) with very little published on ichnostratigraphy (CiabeGhodsi et al., Reference CiabeGhodsi, Hamdi, Abdi and Sadeghi2006; Tashayoee et al., Reference Tashayoee, Hamdi, Vaziri and Yousofzadeh2012). Small shelly fossils reported from the Soltanieh Formation are comparable with skeletal assemblages recorded in China and northern Siberia (Hamdi et al., Reference Hamdi, Brasier and Zhiwen1989), containing elements of A. trisulcatus-P. anabarica and W. crosbyi assemblage zones of Meishucunian Stage, and the Pelagiella subangulata Taxon Zone of Qiongzhusian Stage (Steiner et al., Reference Steiner, Li, Qian, Zhu and Erdtmann2007).
Although the carbonate intervals (Lower, Middle and Upper Dolomite members) do not contain trace fossils, the clastic deposits of the Soltanieh Formation (Lower and Upper Shale members) are host to trace fossils of biostratigraphic utility, which have largely been overlooked. The Lower Shale Member contains ichnotaxa that are known from both Ediacaran and early Cambrian rocks and those that are only known from the Phanerozoic. The former group includes the simple grazing trail, namely Helminthoidichnites tenuis and Helminthopsis tenuis, whereas the second group consists of the grazing trail Cochlichnus anguineus, and the bilobate trail Cruziana isp. The latter occurs on the same bed as the branching burrow Treptichnus pedum. The Upper Shale Member contains fifteen ichnospecies, including Cochlichnus anguineus, Cruziana problematica, Curvolithus isp., Didymaulichnus miettensis, Gordia marina, Helminthoidichnites tenuis, Helminthopsis tenuis, Palaeophycus tubularis, Phycodes isp., Planolites montanus, Psammichnites gigas, Rusophycus avalonensis, Treptichnus pedum, Treptichnus pollardi, and Treptichnus isp.
Our study indicates that four distinctive trace-fossil zones can be recognized in the Soltanieh Formation, all belonging to the early Cambrian (Fig. 6). Ichnozone 1 characterizes the middle interval of the Lower Shale Member (157–171 m above its base). This ichnozone is of low ichnodiversity, containing only three ichnotaxa of simple horizontal trails, namely Helminthoidichnites tenuis, Helminthopsis tenuis, and Cochlichnus anguineus, collected 157 m above the base of the Lower Shale Member (Fig. 7). A wrinkled surface associated with these specimens suggests the presence of microbial mats (Fig. 2.1), which allowed the superb preservation of these delicate structures. Cochlichnus anguineus, Helminthoidichnites tenuis, and Helminthopsis tenuis commonly occur in direct association with the microbial mat and record microbial grazing as one of the most widespread feeding strategies across the Ediacaran-Cambrian boundary (Buatois et al., Reference Buatois, Narbonne, Mángano, Carmona and Myrow2014).

Figure 6 Stratigraphic column of the Soltanieh Formation, showing distribution of trace fossils, small shelly fossils, biozonation based on trace fossils and small shelly fossils, and correlation in relation to the Yangtze platform, China. Small shelly fossil data based on Hamdi et al. (Reference Hamdi, Brasier and Zhiwen1989), CiabeGhodsi (Reference CiabeGhodsi2007), and Tashayoee et al. (Reference Tashayoee, Hamdi, Vaziri and Yousofzadeh2012).
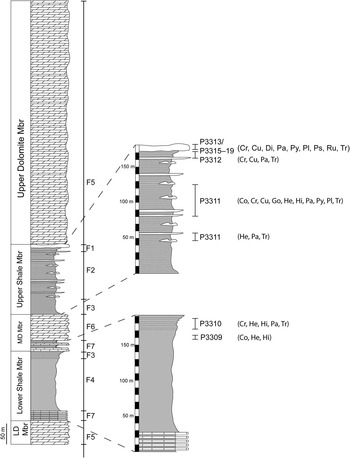
Figure 7 Distribution of sedimentary facies in the Soltanieh Formation, with a detail on trace-fossil distribution in the siliciclastic units indicating the location where the ichnofossiliferous samples were collected. Cochlichnus (Co), Cruziana (Cr), Curvolithus (Cu), Didymaulichnus (Di), Gordia (Go), Helminthoidichnites (Hi), Helminthopsis (He), Palaeophycus (Pa), Planolites (Pl), Phycodes (Py), Psammichnites (Ps), Rusophycus (Ru), Treptichnus (Tr).
Although this ichnofauna shows similarities with that of the Ediacaran (Fedonkin, Reference Fedonkin1985; Narbonne et al., Reference Narbonne, Myrow, Landing and Anderson1987; Jensen, Reference Jensen1997; MacNaughton and Narbonne, Reference MacNaughton and Narbonne1999; Gehling et al., Reference Gehling, Droser, Jensen and Runnegar2005; Buatois and Mángano, Reference Buatois and Mángano2011; Mángano et al., Reference Mángano, Buatois and MacNaughton2012), the presence of the Anabarites trisulcatus-Protohertzina anabarica Zone (Hamdi et al., Reference Hamdi, Brasier and Zhiwen1989) in the dolomites below and above the Lower Shale indicates a Fortunian age. Also, uncontroversial exmples of Cochlichnus have not been recorded in the Ediacaran (Buatois and Mángano, Reference Buatois and Mángano2016). The most likely interpretation is to consider this ichnofauna as the distal expression of those that characterize the Treptichnus pedum Zone (see Discussion). Previous reports of Chuaria sp. from the lower interval of the Lower Shale Member at Vali-Abad and Soltanieh Mountains (Hamdi et al., Reference Hamdi, Brasier and Zhiwen1989; CiabeGhodsi, Reference CiabeGhodsi2007) indicate that Chuaria persisted into the lowermost Cambrian. A similar situation has been observed in the Heziao and Jijiapo sections, Hubei province, southern China, where Chuaria sp. has been reported from siltstone and chert from the lower Cambrian Yanjahe Formation (Steiner, Reference Steiner1994). Amard (Reference Amard1997) also suggested an early Cambrian age for Chuaria from the Pendjari Formation of West Africa. These findings question the utility of Chuaria for global correlation of Proterozoic successions. If this is the case, then either the whole Soltanieh Formation is of early Cambrian age, or the Ediacaran-Cambrian boundary is placed within the Lower Dolomite Member below the oldest occurrence of SSF.
Ichnozone 2 represents the uppermost interval of the Lower Shale Member, the Middle Dolomite Member, and the lower interval of the Upper Shale Member (171 m above the base of the Lower Shale Member to 80 m above the base of the Upper Shale Member). A total of six ichnospecies occur in this zone including, Cruziana isp., Gordia arcuata, Helminthoidichnites tenuis, Helminthopsis tenuis, Palaeophycus tubularis, and Treptichnus pedum, indicating higher ichnodiversity than in the underlying ichnozone 1. The presence of Cruziana isp. in these strata is particularly significant because it reinforces evidence for the presence of arthropod-produced trace fossils in the Treptichnus pedum Zone. Most of the trace fossils appear in the lowermost interval of the zone (171–173 m above the base of Lower Shale Member) (Fig. 7). This ichnozone is defined by the first appearance of T. pedum, and is regarded as Fortunian in age (Jensen, Reference Jensen2003; Buatois and Mángano, Reference Buatois and Mángano2011), being referred to as Ichnozone II in the global ichnostratigraphic scheme or T. pedum Zone (Narbonne et al., Reference Narbonne, Myrow, Landing and Anderson1987; MacNaughton and Narobonne, 1999; Jensen, Reference Jensen2003). This is consistent with the presence of the Anabarites trisulcatus-Protohertzina anabarica Zone (Hamdi et al., Reference Hamdi, Brasier and Zhiwen1989). In strict ichnologic terms, the first occurrence of Treptichnus pedum together with the first bilobate trace Cruziana isp. may be taken as evidence that the Ediacaran-Cambrian boundary is placed within the Lower Shale Member, 171 m above its base. As discussed above, however, integration with small shelly fossils and evaluation of environmental constraints suggest placing the Ediacaran-Cambrian boundary at the base of the Soltanieh Formation or within the Lower Dolomite Member, rather than within the Lower Shale Member.
Ichnozone 3 characterizes the middle interval of the Upper Shale Member (80–178 m above its base; Fig. 7). This ichnozone is defined by a sudden increase in the abundance of trace fossils and the occurrence of more complex burrows, including Cruziana problematica, Phycodes isp., and Treptichnus pollardi. The other elements of this ichnozone are Cochlichnus anguineus, Curvolithus isp., Gordia marina, Helminthoidichnites tenuis, Helminthopsis tenuis, Palaeophycus tubularis, Planolites montanus, Treptichnus pedum, and Treptichnus isp. This ichnozone corresponds to ichnozone III of the global ichnofossil scheme, encompassing the lower half of the Rusophycus avalonensis Zone of the Chapel Island Formation, which represents the upper half of Member 2 and higher strata of the Chapel Island Formation, Burin Peninsula (Narbonne et al., Reference Narbonne, Myrow, Landing and Anderson1987), and the lower half of the Rusophycus avalonensis Zone of the Mackenzie Mountains, which characterizes the Backbone Ranges Formation and the lower interval of the Vampire Formation (MacNaughton and Narbonne, Reference MacNaughton and Narbonne1999). However, R. avalonensis has not been found at comparable levels in the Soltanieh Formation. Ichnozone 3 is interpreted as late Fortunian–Cambrian Age 2 (Buatois and Mángano, Reference Buatois and Mángano2011).
Ichnozone 4 represents the uppermost interval of the Upper Shale Member (178 m above its base to the base of the Upper Dolomite Member; Fig. 7). This zone is based on the first appearance of large back-filled trace fossils (Psammichnites gigas), together with bilobate, trilobite-like resting trace fossils (Rusophycus avalonensis), and large locomotion trace fossils (Didymaulichnus miettensis). The other elements of this ichnozone are Cruziana isp., Curvolithus isp., Palaeophycus tubularis, Phycodes isp., Planolites montanus, and Treptichnus pedum. Ichnozone 4 corresponds to ichnozones III and IV of the global scheme, encompassing both the upper half of the Rusophycus avalonensis Zone of Burin Peninsula (Narbonne et al., Reference Narbonne, Myrow, Landing and Anderson1987) and the Mackenzie Mountains (MacNaughton and Narbonne, Reference MacNaughton and Narbonne1999), and the Cruziana tenella Zone of the latter region, which characterizes the upper interval of the Vampire Formation (MacNaughton and Narbonne, Reference MacNaughton and Narbonne1999). This zone is regarded as Cambrian Age 2–3 (Buatois and Mángano, Reference Buatois and Mángano2011). This is supported by the presence of Pelagiella lorenzi (Pelagiella subangulata; Steiner et al., Reference Steiner, Li, Qian, Zhu and Erdtmann2007).
Discussion
The Ediacaran-Cambrian boundary is globally defined by a point placed with specific reference to the first appearance of T. pedum in the global stratotype section in Newfoundland (Narbonne et al., Reference Narbonne, Myrow, Landing and Anderson1987) and in other regions, including the Mackenzie Mountains of Canada (MacNaughton and Narbonne, Reference MacNaughton and Narbonne1999; Carbone and Narbonne, Reference Carbone and Narbonne2014), the Flinders Ranges of South Australia (Jensen et al., Reference Jensen, Gehling and Droser1998), eastern Finnmark, Norway (Føyn and Glaessner, Reference Føyn and Glaessner1979; Högström et al., Reference Högström, Jensen, Palacios and Ebbestad2013), Sonora, Mexico (Stewart et al., Reference Stewart, McMenamin and Morales-Ramirez1984; Sour-Tovar et al., Reference Sour-Tovar, Hagadorn and Huitrón-Rubio2007), and Death Valley, Eastern California (Jensen et al., Reference Jensen, Droser and Heim2002), among many other areas. In the Alborz Mountains of northern Iran, however, the first appearance datum of Treptichnus pedum occurs stratigraphically higher in the section. Although ichnozone 1 consists of grazing trails, which are common in Ediacaran strata elsewhere, these trace fossils are also abundant in Fortunian deposits in Burin Peninsula, in connection with microbially stabilized surfaces (Buatois et al., Reference Buatois, Narbonne, Mángano, Carmona and Myrow2014). In contrast to the Fortunian of Burin Peninsula, arthropod trackways are absent in ichnozone 1 in the Soltanieh Formation. We hypothesize that the presence of an ichnofauna of “Ediacaran aspect” and the late appearance of Treptichnus pedum in the Soltanieh Formation is due to environmental constraints. Buatois et al. (Reference Buatois, Almond and Germs2013) noted that this ichnotaxon has a broad environmental tolerance, albeit displaying a preference for sandy substrates. The shelf shales of the Soltanieh Formation may have represented the seaward limit of the T. pedum producer. Alternatively, because T. pedum is typically preserved along lithologic interfaces, its absence in the shelfal shale may simply reflect a taphonomic constraint. Therefore, ichnozone 1 in the Alborz Mountains may be understood as a distal expression of the Treptichnus pedum Zone, which is commonly recognized in settings above storm wave base (Buatois et al., Reference Buatois, Almond and Germs2013). A similar situation has been noted in the Ediacaran–Cambrian succession of eastern Yunnan Province, South China (Zhu, Reference Zhu1997), western Mongolia (Smith et al., Reference Smith, Macdonald, Petach, Bold and Schrag2015), Lesser Himalaya, India (Singh et al., Reference Singh, Lokho, Kishore and Virmani2014), and southeastern Kazakhstan (Weber et al., Reference Weber, Steiner, Evseev and Yergaliev2013), where the first appearance of the trace-fossil T. pedum postdates the Ediacaran–Cambrian transition.
Ichnozone 2 of the Soltanieh Formation corresponds in part to ichnozone III of global ichnofossil zones and the Treptichnus pedum Zone of the Chapel Island Formation, Burin Peninsula, which characterizes the lower part of Member 2, from 2.4 to 133 m above its base (Narbonne et al., Reference Narbonne, Myrow, Landing and Anderson1987; Landing, Reference Landing1996). This ichnozone is also represented in the upper interval of the Ingta Formation in the Mackenzie Mountains (MacNaughton and Narbonne, Reference MacNaughton and Narbonne1999) and the Nomtsas Formation of Namibia (Crimes and Germs, Reference Crimes and Germs1982; Geyer and Uchman, Reference Geyer and Uchman1995), among other areas. Integration of trace fossils and small shelly faunas suggests that ichnozone 2 of the Soltanieh Formation is best regarded as the upper half of the global Treptichnus pedum Zone to accommodate the shelf deposits of early Fortunian age that make up the lower interval of the Lower Shale Member.
In sections worldwide, Cruziana and Rusophycus commonly occur stratigraphically below the first occurrence of trilobite body fossils, and above the first appearance of Treptichnus pedum (Crimes, Reference Crimes1987). In the Chapel Island Formation in Newfoundland, for example, Rusophycus avalonensis appears ~250 meters up-section from T. pedum, and in current global ichnostratigraphic schemes this ichnotaxon first appears below the Cruziana problematica Zone. In the Soltanieh Formation, however, R. avalonensis has been collected above Cruziana problematica and together with Psammichnites gigas within ichnozone 4. In this formation, the first appearance of bilobate cruzianid traces occurs at the base of the ichnozone 2, whereas Cruziana problematica first appears in ichnozone 3, considerably above (172 m) the first appearance of T. pedum. In strict sense, because Cruziana and Rusophycus are both produced by trilobites or trilobitomorph arthropods, there is no a priori reason why Cruziana should occur stratigraphically above Rusophycus.
Finally, Hamdi et al. (Reference Hamdi, Brasier and Zhiwen1989) noted that the phosphatic layers near the base of the Upper Shale Member might be part of a contemporaneous event across the Palaeotethyan belt, underscoring similarities with successions in China, India, Pakistan, Kazakhstan, and Mongolia. However, correlations suggest a more complicated pattern. In South China, the phosphate layers of the Upper Phosphate Member occur within the R. avalonensis and Paragloborilus subglobosus-Purella squamulosa biozones (Zhu, Reference Zhu1997; Steiner et al., Reference Steiner, Li, Qian, Zhu and Erdtmann2007). In southeastern Kazakhstan, the phosphate deposits of the Aksai Member occur below the R. avalonensis Zone, and within the Purella antigua assemblage zone (Weber et al., Reference Weber, Steiner, Evseev and Yergaliev2013). In western Mongolia, the phosphate layers at the base of Member 2 of the Bayangol Formation occur within the Anabarites trisulcatus-Protohertzina anabarica Assemblage Zone and below the R. avalonensis Zone (Smith et al., Reference Smith, Macdonald, Petach, Bold and Schrag2015). The Chert-Phosphate Member of Northern India occurs within the Anabarites trisulcatus-Protohertzina anabarica Assemblage Zone, below the R. avalonensis Zone (Desai et al., Reference Desai, Shukla and Saklani2010). In northern Pakistan, the phosphate-bearing deposits at the top of Abbottabad Formation are believed to be of earliest early Cambrian age (Latif, Reference Latif1972; Hasan, Reference Hasan1986). However, due to lack of biostratigraphic and geochronological constraints, the age of the Abbottabad Formation is in dispute.
Therefore, the phosphatic layers of the Upper Shale Member are most likely coeval with those in the Upper Phosphate Member in Yunnan and the Karatau Member in Southeastern Kazakhstan, whereas Member 2 of the Bayangol Formation of western Mongolia, the Chert-Phosphate Member in India, and the upper Abbottabad Formation in Pakistan seem to be older (Fig. 8). To summarize, the integration of stratigraphic, sedimentologic, and biostratigraphic data suggests two phosphatic events (early Fortunian and late Fortunian) rather than one.

Figure 8 Stratigraphic column of the Soltanieh Formation, showing distribution of trace fossils, small shelly fossils, biozonation based on trace fossils and small shelly fossils, and correlation in relation to the Yangtze platform, China. Sources: Latif (Reference Latif1972), Hasan (Reference Hasan1986), Hamdi et al. (Reference Hamdi, Brasier and Zhiwen1989), Zhu (Reference Zhu1997), Steiner et al. (Reference Steiner, Li, Qian, Zhu and Erdtmann2007), Desai et al. (Reference Desai, Shukla and Saklani2010), Weber et al. (Reference Weber, Steiner, Evseev and Yergaliev2013), and Smith et al. (Reference Smith, Macdonald, Petach, Bold and Schrag2015).
Conclusion
Trace-fossil assemblages recorded from the Soltanieh Formation in northern Iran are characterized by low to moderate diversity, dominance of very simple forms, and a combination of locomotion, grazing, and dwelling structures. Ichnodiversity increases towards the top of the unit. An analysis of the Soltanieh ichnofauna indicates that four ichnozones may be recognized. Ichnozone 1 is of early Fortunian age and is characterized by low diversity of simple grazing traces. Ichnozone 2 is of middle Fortunian age, and is defined based on the first appearance of Treptichnus pedum and the earliest bilobate trace Cruziana isp. Ichnozone 3 is late Fortunian–Cambrian Age 2, and is marked by sudden change in abundance and complexity of trace fossils and the first appearance of Cruziana problematica. Ichnozone 4 is Cambrian Ages 2–3, and is characterized by the first appearance of Psammichnites gigas, Rusophycus avalonensis, and Didymaulichnus miettensis. An integration of trace fossils with small shelly fossils and evaluation suggest that the Ediacaran-Cambrian boundary should be placed at the base of the Soltanieh Formation or within the Lower Dolomite Member.
Acknowledgments
Financial support for this study was provided by Natural Sciences and Engineering Research Council (NSERC) Discovery Grants 311727–08/15 and 311726–13 awarded to Mángano and Buatois, respectively, as well as a University of Saskatchewan President Fund to Mángano. A. CiabeGhodsi is thanked for introducing us to the area and providing valuable feedback. A. EbrahimNejad and H. Behmaram helped the first author during fieldwork. Reviewers S. Jensen and M. Laflamme and Associate Editor L. Tapanila provided very useful comments that helped to improve the manuscript.