Carotenoids comprise an important class of phytochemicals in plants. Among more than 600 naturally occurring carotenoids, α-carotene, β-carotene and β-cryptoxanthin are the major provitamin A carotenoids present in foods. The bioconversion rate of β-cryptoxanthin, a mono-hydroxylated xanthophyll found in tangerine, papaya, apricot, and red pepper, to vitamin A (VA) is not known. Efforts to biofortify foods such as carrots and maize (i.e. increase the provitamin A content) have focused on β-carotene(Reference Porter Dosti, Mills, Simon and Tanumihardjo1, Reference Howe and Tanumihardjo2); however, some varieties of biofortified maize contain substantial amounts of β-cryptoxanthin(Reference Howe and Tanumihardjo2). In order to direct maize-breeding efforts, it is important to know the contribution of β-cryptoxanthin to VA status.
The structure of β-cryptoxanthin implies that one molecule will yield one molecule of retinol (Fig. 1). Unlike the hydrocarbons, α- and β-carotene, β-cryptoxanthin is bipolar because of the electronegative oxygen in the hydroxyl group on one side of the molecule. The effects of this bipolar structure on incorporation into mixed micelles and its impact on bioavailability have not been studied. As proposed by the Institute of Medicine (IOM)(3), the bioconversion of dietary β-cryptoxanthin to VA is reported to be the same as α-carotene, i.e. 24 μg β-cryptoxanthin or α-carotene to 1 μg retinol(3). This is twice the amount of β-carotene, i.e. 12 μg β-carotene to 1 μg retinol, and is based on the theoretical yield from the chemical structure. The IOM bioconversion factors were calculated from human trials, and are used to measure retinol activity equivalents of food(3). Previously, lower conversion factors were used and defined as retinol equivalents, i.e. 6 μg β-carotene to 1 μg retinol. When α-carotene in oil was fed to Mongolian gerbils (Meriones unguiculatus)(Reference Tanumihardjo and Howe4), twice the molar amount was as effective as β-carotene in maintaining VA status, but its conversion factor was higher, i.e. about 5·5 μg α-carotene to 1 μg retinol, than that proposed by the IOM for carotenoids in oil, i.e. 4 μg α-carotene to 1 μg retinol(3).
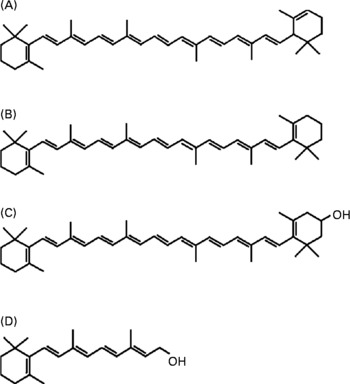
Fig. 1 The chemical structures of (A) α-carotene, (B) β-carotene, (C) β-cryptoxanthin and (D) retinol. Theoretically, 1 mol α-carotene and β-cryptoxanthin provide 1 mol retinol, and 1 mol β-carotene provides 2 mol retinol.
Besides providing VA, intake of foods containing β-cryptoxanthin has been linked to reduced risk of specific diseases(Reference Tanumihardjo, Yang, Caballero, Allen and Prentice5). Epidemiological studies show that β-cryptoxanthin is associated with reduced risk of inflammatory disorders such as rheumatoid arthritis and polyarthritis(Reference Cerhan, Saag, Merlino, Mikuls and Criswell6, Reference Pattison, Symmons, Lunt, Welch, Bingham, Day and Silman7). In vitro investigations(Reference Yamaguchi and Uchiyama8, Reference Yamaguchi and Uchiyama9) and in vivo studies in rats(Reference Yamaguchi, Uchiyama, Ishiyama and Hashimoto10, Reference Uchiyama and Yamaguchi11) suggest β-cryptoxanthin may also be important for bone health. Other studies show that a high dietary intake of β-cryptoxanthin reduced the risk of angina pectoris(Reference Ford and Giles12), cancer(Reference Chen, Tucker, Graubard, Heineman, Markin, Potischman, Russell, Weisenburger and Ward13, Reference Zeegers, Goldbohm and Brand14) and hyperglycaemia(Reference Suzuki, Ito, Nakamura, Ochiai and Aoki15). In addition to determining VA value, further studies with β-cryptoxanthin are important to understand the mechanisms of these health benefits.
The Mongolian gerbil metabolises α- and β-carotene similarly to man(Reference Porter Dosti, Mills, Simon and Tanumihardjo1, Reference Tanumihardjo and Howe4, Reference Lee, Lederman, Hofmann and Erdman16). The xanthophyll lutein is not very bioavailable in gerbils(Reference Molldrem and Tanumihardjo17, Reference Escaron and Tanumihardjo18), and β-cryptoxanthin has not been evaluated. If bioavailable, β-cryptoxanthin from maize may contribute to the VA needs of gerbils in addition to β-carotene(Reference Howe and Tanumihardjo2). Two studies were conducted to evaluate the VA value of β-cryptoxanthin using Mongolian gerbils. Study 1 compared the bioconversion of β-cryptoxanthin and β-carotene to retinol from oil supplements. Study 2 evaluated the VA value of biofortified maize with enhanced β-cryptoxanthin and β-carotene by comparing it with VA and β-carotene supplements.
Materials and methods
Animals and diet
Ninety-four male Mongolian gerbils, age 40 d, from Charles River Laboratories (Kingston, NY, USA) were used. Upon arrival, gerbils were individually housed in plastic cages under controlled temperature, humidity and light (12 h light–12 h dark cycle). The gerbils were weighed daily to monitor health for the first 2 weeks of each study and then three times per week until kill. One gerbil did not adapt to the feed and died. During the 4-week VA-depletion phase, gerbils were acclimated to oral dosing by administering 40 μl cottonseed oil every 2 d, using a 100 μl Gilson positive displacement pipette (Rainin Instruments, Woburn, MA, USA). Cottonseed oil was chosen because it is naturally devoid of carotenoids. After the depletion phase, gerbils were weight-matched and distributed among treatment groups. All animal handling procedures were approved by University of Wisconsin-Madison's Research Animal Resource Center.
In study 1, gerbils were fed a VA- and carotenoid-free powdered diet containing 45 % maize ad libitum (Reference Howe and Tanumihardjo2) (Harlan Teklad; Madison, WI, USA). For study 2, a VA-free basal diet (Harlan Teklad) was mixed with 50 % maize (Table 1). White maize was used during the depletion phase and for positive and negative controls during the treatment phase. In study 2, the maize treatment diet was prepared using maize with enhanced β-cryptoxanthin and β-carotene that was accomplished through conventional breeding (biofortified maize). The biofortified maize was KUI Orange Synthetic, a synthetic of KUI3, KUI11, KUI43 and KUI2007 inbreds from Kasetsart University (Thailand) which were chosen for high levels of β-cryptoxanthin and β-carotene, inter-mated, and selected recurrently for deep orange color. All maize kernels were ground to pass a 1 mm screen using a C&N hammer mill no. 8 (Christy-Norris Ltd, Ipswich, Suffolk, UK) and stored at − 80°C before mixing. White maize diets were stored at 4°C, and the biofortified maize diet for study 2 was stored at − 20°C during use.
Table 1 Composition of experimental diets (g/kg feed) fed to Mongolian gerbils (Meriones unguiculatus) differing by percentage maize*

* Diets provided by Harlan Teklad (Madison, WI, USA). White maize was used for depletion periods and controls. Biofortified maize with enhanced β-cryptoxanthin and β-carotene was used for one treatment group.
† AIN-93M-MX.
‡ Vitamin mix provided the following (mg/kg feed): biotin, 0·4; calcium pantothenate, 66·1; folic acid, 2; inositol, 110·1; menadione, 49·6; niacin, 99·1; p-aminobenzoic acid, 110·1; pyridoxine-HCl, 22; riboflavin, 22; thiamin-HCl, 22; vitamin B12 (0·1 % in mannitol), 29·7; ascorbic acid (97·5 %), 1016·6.
Analysis of maize and diet
The carotenoid composition of the maize and diets were determined using a published saponification method followed by HPLC using a C30 YMC carotenoid column (4·6 × 250 mm, 3 μm; Waters Corporation; Milford, MA, USA)(Reference Howe and Tanumihardjo2). Prior analysis of maize without saponification(Reference Rodriguez-Amaya and Kimura19) confirmed that β-cryptoxanthin occurs in the free form and not esterified to fatty acids. Saponification was used because it yields a lower CV during analysis(Reference Howe and Tanumihardjo20). The HPLC solvent gradient was slightly modified. Solvent A was methanol–water (92:8, v/v) with 10 mm-ammonium acetate and solvent B was 100 % methyl-tertiary-butyl ether. Samples were analysed at 1 ml/min starting with 70 % solvent A and transitioning to 40 % within 30 min. The column was equilibrated with 70 % solvent A for 10 min before the next injection. Lutein, zeaxanthin, β-cryptoxanthin, β-carotene and α-carotene were identified using HPLC purified standards. Concentrations of standards were determined spectrophotometrically using their respective extinction coefficient (E1 %1 cm). Chromatograms were generated at 450 nm. The biofortified maize diet had 9·3 nmol retinol/g, assuming 100 % bioefficacy of the provitamin A carotenoids to yield retinol on a theoretical basis, including 9- and 13-cis-β-carotene. β-Cryptoxanthin (2·9 nmol/g) and β-carotene (3·2 nmol/g) were the major contributors to the VA value of the maize diet; α-carotene was not detected.
Dose preparation
For study 1, β-cryptoxanthin was isolated from concentrated tangerine juice by repeatedly extracting with diethyl ether–hexanes (25:75, v/v). The extract was washed with water. The organic layer was dried with sodium sulfate, evaporated with a rotary evaporator, and the carotenoids were semi-purified on a 2 % water-deactivated alumina column. Distinct isolates were collected(Reference Rodriguez-Amaya and Kimura19) and saponified with potassium hydroxide–water (50:50, w/v) at 45°C for 30 min. The reaction was quenched with water and the carotenoids were extracted with hexanes and ethyl acetate. The extracts were dried and stored in hexanes. Each saponified fraction was analysed by photodiode array HPLC to verify β-cryptoxanthin content using a Resolve® 5 μm C18 column (3·9 × 300 mm; Waters Corporation, Milford, MA, USA) and further purified using an Ultrasphere® 5 μm C18 column (10 × 250 mm; Beckman, San Ramon, CA, USA) as described(Reference Tanumihardjo and Howe4). The retention time of β-cryptoxanthin was 8 min with isocratic elution of methanol–acetonitrile–dichloroethane (40:40:20, by vol.). This process yielded 2·5 mg pure β-cryptoxanthin. Because the process was time consuming, additional β-cryptoxanthin of high purity was purchased (CaroteNature, GmbH, Lupsingen, Switzerland) and mixed with that purified from tangerine juice. The combined purity of β-cryptoxanthin fed to the gerbils was >99 %, as determined by photodiode array detection with spectral analysis (Fig. 2).

Fig. 2 A chromatogram of an isopropanol dilution of β-cryptoxanthin dissolved in cottonseed oil that was administered to Mongolian gerbils (Meriones unguiculatus) to determine vitamin A value. The Waters HPLC system consisted of a guard column, Resolve™ C18 column (5 μm, 3·9 × 300 mm), 1525 binary pump, 717 autosampler and 996 photodiode array detector (Milford, MA, USA). The mobile phases were acetonitrile–water (95:5, v/v; solvent A) and acetonitrile–methanol–dichloroethane (85:10:5, by vol.; solvent B) with 10 mm-ammonium acetate. The gradient at 2 ml/min was: (1) 100 % A for 3 min, (2) a 7 min linear change to 100 % B, (3) a 15 min hold at 100 % B, and (4) a 2 min reverse gradient to 100 % A. Absorbance was monitored from 200 to 600 nm and the chromatogram was generated at 450 nm. AU, absorbance units.
To prepare the supplement, β-cryptoxanthin was dissolved into cottonseed oil using sonication. The concentration was determined spectrophotometrically using the extinction coefficient (E1 %1 cm) of 2386 at 452 nm(Reference DeRitter, Purcell and Bauerfeind21) by diluting a sample into hexanes. For both studies, VA and β-carotene doses were prepared as previously described(Reference Howe and Tanumihardjo2, Reference Tanumihardjo and Howe4). In study 2, dose volumes were adjusted based on feed intake of gerbils receiving biofortified maize, which was measured daily. Table 2 summarises the doses used for the studies.
Table 2 Oil doses prepared for two studies performed in Mongolian gerbils (Meriones unguiculatus) to determine the bioefficacy of β-cryptoxanthin as an oil supplement or from carotenoid-biofortified maize*

* The maize diet for study 2 contained 2·9 nmol β-cryptoxanthin/g and 3·2 nmol β-carotene/g for a total of 9·3 nmol theoretical retinol/g. The gerbils in that group ingested 1551 nmol theoretical retinol during the 4-week treatment phase.
† Doses were administered twice per d. For study 2, the doses were based on carotenoid intake from maize which was measured on the previous day and adjusted throughout the study.
‡ The interventions for studies 1 and 2 were 3 and 4 weeks long, respectively.
Experimental design
Study 1 was designed to be directly compared with a prior study evaluating α-carotene(Reference Tanumihardjo and Howe4), which included a 4-week VA-depletion and 3-week treatment phase. At 4 weeks, seven gerbils were killed by exsanguination while under isoflurane anaesthesia to establish baseline liver retinol reserves; blood and livers were collected. The remaining gerbils (n 40) were placed into four weight-matched groups. Treatments included daily supplementation with 35 nmol VA, 35 nmol β-cryptoxanthin, 17·5 nmol β-carotene, or cottonseed oil. Daily supplements were divided in half and administered at 09.00 and 14.00 hours. These physiological doses were based on a prior study with α-carotene(Reference Tanumihardjo and Howe4) and basal daily VA needs of gerbils (3·1 μg/100 g body weight (BW))(Reference Lee, Lederman, Hofmann and Erdman16). After 3 weeks, gerbils were killed and liver and blood were collected for retinol and carotenoid analysis.
Study 2 began with a 4-week VA-depletion period. At 4 weeks, six gerbils were killed; blood and livers were collected for baseline retinol measurements. The remaining gerbils (n 40) were placed into four weight-matched groups. One group was fed the 50 % biofortified maize diet ad libitum with daily doses of cottonseed oil. This level of dietary intake of maize would be similar to countries that consume maize as a staple food. The other three groups received VA-free, 50 % white maize diets and oil only or β-carotene or VA supplements adjusted to provide the theoretical VA intake of the biofortified maize group, determined with a subset on the previous day. Supplements were divided in half and administered at 09.00 and 16.00 hours. Similar to a prior study with biofortified maize feeding(Reference Howe and Tanumihardjo2), gerbils were killed after 4 weeks. Blood and livers were collected. Serum was prepared by centrifuging blood at 2200 g at 4°C for 15 min in BD Vacutainer™ Gel and Clot Activator tubes (Becton Dickinson, Franklin Lakes, NJ, USA). Serum and livers were stored at − 80°C to − 70°C until analysis.
Serum and liver retinol and carotenoid concentrations
Serum (500 μl) and livers (0·6 g) were analysed as previously described(Reference Howe and Tanumihardjo2, Reference Tanumihardjo and Howe4). Retinyl butyrate was used as an internal standard to determine extraction efficiency and as an external standard for quantification. Briefly, serum proteins were denatured with ethanol and the retinol was extracted with hexanes. Liver was ground with anhydrous sodium sulfate and extracted with 1,2-dichloromethane. The extract was filtered into a 50 ml volumetric flask and brought to volume. Samples were analysed by HPLC. Chromatograms were generated at 325 nm for retinol and 450 nm for carotenoids. Liver retinol, expressed as a concentration (μmol/g) and total liver content (μmol/liver), were the sum of retinol and all identifiable retinyl esters. Spectral analyses using a Waters 996 photodiode array detector (Milford, MA, USA) were used to identify retinyl esters and therefore saponification was not employed for liver total retinol analysis. Purified β-carotene was used for quantification of total liver β-carotene content (nmol/liver). Carotenoids identified in liver were not included in the calculation for retinol.
Statistical analysis and calculations
Values are means and standard deviations. Data were analysed using Statistical Analysis System software (SAS Institute Inc., version 8.2, Cary, NC, USA; 2001). Differences in the outcomes of interest (i.e. gerbil weights, liver weights, serum retinol concentration and liver retinol reserves) among groups were analysed by using one-way ANOVA with PROC MIXED or the GLM procedure. When ANOVA was significant, least significant difference tests were performed between groups. P < 0·05 was considered significant.
Retinol utilisation in study 1 was calculated for the control and VA groups(Reference Tanumihardjo and Howe4). Utilisation for the control group represents the change in liver retinol between the baseline and control groups divided by the number of sustained VA depletion days. Utilisation for the VA group was calculated by subtracting the difference in liver retinol between the VA and control groups from the 210 μg total retinol intake and dividing by the number of treatment days, i.e. 21 d. Both values were corrected to 100 g BW from the mean final BW, i.e. about 70 g. Conversion factors for β-cryptoxanthin and β-carotene in study 1 were calculated by comparing total liver retinol of the β-cryptoxanthin and β-carotene groups with the VA group and corrected for differences in formula weight. Conversion factors for β-carotene and the provitamin A carotenoids in the biofortified maize were calculated by comparing total liver retinol of the VA group with the β-carotene supplement and maize group. Because maize contains both β-cryptoxanthin and β-carotene, conversion factors were expressed as β-carotene equivalents, which equal the μg equivalent of 1 mol β-carotene +0·5 mol β-cryptoxanthin and account for the fact that only half of the β-cryptoxanthin molecule supplies VA. The mean total liver retinol of the control group was subtracted from each treatment group before calculation.
Results
Food intake and body and liver weights
In study 1, the food intake, BW and liver weight of the gerbils did not differ among treatment groups. Food intake ranged from 5·76 (sd 0·95) g in the VA group to 6·22 (sd 0·68) g in the β-cryptoxanthin group. The final BW ranged from 66·0 (sd 3·6) g in the baseline group to 71·1 (sd 5·8) g in the β-cryptoxanthin group, and the final liver weights ranged from 2·44 (sd 0·33) g in the β-cryptoxanthin group to 2·62 (sd 0·15) g in the baseline group. In study 2, food intake of the maize group was 5·94 (sd 1·0) g/d, corresponding to a mean β-carotene and VA intake of 27·7 (sd 2·7) and 55·4 (sd 3·8) nmol/d, respectively, for the supplement groups. With the exception of baseline BW (65·6 (sd 4·3) g), mean BW (74·6 (sd 7·1) g) did not differ among treatment groups. Liver weights did not differ and ranged from 2·39 (sd 0·35) to 2·73 (sd 0·36) g in the negative control and β-carotene groups, respectively.
Serum and liver vitamin A and carotenoids
In study 1, serum retinol concentrations were higher in the baseline and control groups compared with the supplement groups (P < 0·05) (Fig. 3 (A)). The serum retinol concentrations of the supplement groups did not differ. For study 2, serum retinol concentrations were higher in the baseline group than in the biofortified maize group (Fig. 4 (A); P < 0·05), but other groups did not differ. Similar to prior studies, carotenoids were not detected in the serum(Reference Porter Dosti, Mills, Simon and Tanumihardjo1, Reference Howe and Tanumihardjo2, Reference Tanumihardjo and Howe4).

Fig. 3 Study 1: serum retinol concentrations (μmol/l) (A), liver retinol concentrations (μmol/g) (B) and total liver retinol reserves (μmol/liver) (C) in Mongolian gerbils (Meriones unguiculatus). Measurements were taken at baseline (Base) and 3 weeks after daily treatment with 35 nmol vitamin A (VA), 35 nmol β-cryptoxanthin (βCX), 17·5 nmol β-carotene (βC) or oil-control (Control) supplements. Values are means (n 7 for baseline and n 10 for treatment groups), with standard deviations represented by vertical bars. a,b,c Mean values with unlike letters are significantly different (P < 0·05).

Fig. 4 Study 2: serum retinol concentrations (μmol/l) (A), liver vitamin A (VA) concentrations (μmol/g) in retinol equivalents (B) and total liver retinol reserves (μmol/liver) (C) in Mongolian gerbils (Meriones unguiculatus). Measurements were taken at baseline (Base), or after a 4-week treatment period in which the gerbils were fed a 50 % high β-cryptoxanthin maize diet (Maize) and dosed with cottonseed oil, or fed 50 % carotenoid-free maize diets with oil doses of β-carotene (βC), VA or cottonseed oil (Control). β-Carotene and VA in oil were equalised to the 50 % high-β-cryptoxanthin maize diet based on intake of provitamin A carotenoids on the previous day assuming 100 % bioefficacy. Values are means (n 10 per group except for control (n 8) and baseline (n 6)), with standard deviations represented by vertical bars. a,b,c Mean values with unlike letters are significantly different (P < 0·05).
In study 1, the retinol concentration and total retinol in the liver were affected by treatment (Fig. 3; P < 0·0006). The oil control group had a lower liver retinol concentration (Fig. 3 (B)) and total retinol content (Fig. 3 (C)) than the baseline, VA or β-cryptoxanthin groups (P < 0·05). The VA, β-cryptoxanthin and β-carotene supplement groups did not differ from baseline. Although the β-carotene supplement group had a higher liver retinol concentration and total retinol content than the control group, the difference was not significant. The VA group had a higher liver retinol concentration and total retinol content compared with the β-carotene group (P < 0·05). The total liver retinol content of the β-cryptoxanthin group was higher than the β-carotene group (Fig. 3 (C); P < 0·05), but the liver retinol concentration was not higher (Fig. 3 (B)). Interestingly, the liver retinol concentration and total retinol content of the β-cryptoxanthin group did not differ from the VA group. No carotenoids were detected in study 1 gerbil livers.
The VA group had higher liver retinol than any other group in study 2 (Fig. 4; P < 0·001). The biofortified maize group had higher retinol concentrations and total retinol than the negative control, but not the baseline or β-carotene groups (Fig. 4, P < 0·05). Liver retinol did not differ among the β-carotene, baseline, and negative control groups (Fig. 4). Carotenoids were detected in the livers of the β-carotene and biofortified maize groups. β-Carotene concentrations were 3·3 (sd 2·1) and 2·7 (sd 1·1) nmol/liver in the β-carotene and biofortified maize groups, respectively, and these values did not differ.
Retinol utilisation and conversion factors
Based on the difference in total liver retinol in the baseline and control groups, utilisation for study 1 was 4·2 μg (14·7 nmol) retinol/100 g BW per d during sustained VA depletion. For the VA group, the total retinol fed during the treatment phase was 210 μg and the final total liver retinol was 91 μg higher than the control, which led to utilisation of 8·0 μg (28 nmol) retinol/100 g BW per d. For β-cryptoxanthin and β-carotene in oil, conversion factors were 2·74 μg and 2·52 μg to 1 μg retinol (1·41 mol and 1·34 mol to 1 mol retinol), respectively. In study 2, after mitigating two high outliers in the control group, the bioconversion factor for the biofortified maize was 2·4 μg β-carotene equivalents to 1 μg retinol (1·3 mol to 1 mol retinol) and that of the β-carotene supplement was 4·6 μg β-carotene to 1 μg retinol (2·4 mol to 1 mol retinol). Conversion factors are summarised in Table 3 and compared with IOM values.
Table 3 Bioconversion factors for provitamin A carotenoids administered as either supplements dissolved in cottonseed oil (study 1) or as carotenoid-biofortified maize (study 2) fed to Mongolian gerbils (Meriones unguiculatus)

Discussion
Two studies evaluated the VA value of β-cryptoxanthin in Mongolian gerbils. Gerbils given β-cryptoxanthin or maize in studies 1 and 2 had 0·11 and 0·14 μmol more total liver retinol, respectively, than groups given β-carotene supplements. This would indicate that β-cryptoxanthin is more efficacious than or as efficacious as β-carotene when fed in supplements or food on a theoretical retinol basis (i.e. 2 mol β-cryptoxanthin to 1 mol β-carotene), respectively. The conversion factor of 2·74 μg β-cryptoxanthin in oil to 1 μg retinol in study 1 is much lower than 4 μg to 1 μg as proposed by the IOM(3) and half the published value of 5·5 μg α-carotene to 1 μg retinol(Reference Tanumihardjo and Howe4) obtained in gerbils with an identical study design. This implies that β-cryptoxanthin is better at supplying retinol to gerbils than α-carotene despite theoretically identical contributions. Study 2 confirms that biofortified maize is as effective as β-carotene supplements in maintaining VA status of gerbils(Reference Howe and Tanumihardjo2) and that β-cryptoxanthin contributes to the VA value. Furthermore, the maize group's liver retinol was significantly higher than the negative control group, whereas that of the β-carotene supplement group was not. Indeed, the relationship of total liver retinol among treatments in studies 1 and 2 mirror each other.
Compared with neutral hydrocarbon carotenoids, polar oxygenated carotenoids may be more easily incorporated into micelles allowing more efficient transfer into enterocytes from the lumen(Reference Van het Hof, Brouwer, West, Haddeman, Steegers-Theunissen, van Dusseldorp, Weststrate, Eskes and Hautvast22, Reference Yeum and Russell23). Increased micellarisation of β-cryptoxanthin would maintain efficient absorption when consumed in oil or from food. In study 1, the β-cryptoxanthin dose was twice as concentrated as the β-carotene dose, perhaps leading to more uptake. The increased bioavailability of β-cryptoxanthin from the maize, which accounted for one-third of the theoretical VA, may explain the differences observed in total liver retinol between the β-carotene supplement and maize groups in relation to the control. Although the food matrix can affect carotenoid bioavailability(Reference Yeum and Russell23–Reference Zhou, Gugger and Erdman25), ground maize does not restrict carotenoid release(Reference Howe and Tanumihardjo2).
β-Carotene 15,15′-oxygenase cleaves provitamin A carotenoids to retinol in the intestine through a mono-oxygenase mechanism(Reference Leuenberger, Engeloch-Jarret and Woggon26). Perhaps, the 15,15′-oxygenase was not saturated with the β-carotene dose and feeding twice the molar amount of β-cryptoxanthin resulted in more overall conversion to retinol. Supporting this conclusion is the fact that β-carotene was not detected in study 1 livers but was in study 2 with the higher dose. Alternatively, β-cryptoxanthin may have a greater affinity for this enzyme.
The conversion factor for β-carotene in study 1 was 2·52 μg β-carotene to 1 μg retinol, which is similar to data in VA-depleted gerbils(Reference Howe and Tanumihardjo2, Reference Tanumihardjo and Howe4) and in Indonesian children(Reference Van Lieshout, West, Muhilal, Permaesih, Wang, Xu, van Breemen, Creemers, Verhoeven and Lugtenburg27). The conversion factor of 4·6 μg to 1 μg in study 2 is not contradictory to study 1 for the following reasons. First, gerbils in study 1 were fed less β-carotene than in study 2, 17·5 v. 27·7 nmol β-carotene equivalents/d, respectively. In humans, bioconversion of β-carotene is dependent upon dose size(Reference Tang, Qin, Dolnikowski and Russell28) and inversely related to VA status(Reference Haskell, Jamil, Hassan, Peerson, Hossain, Fuchs and Brown29, Reference Ribaya-Mercado, Solon, Solon, Cabal-Barza, Perfecto, Tang, Solon, Fjeld and Russell30). A bioconversion factor for β-carotene supplements (2·25 mg) was 6·3 μg to 1 μg retinol in Bangladeshi men who had a range of total body retinol reserves(Reference Haskell, Jamil, Hassan, Peerson, Hossain, Fuchs and Brown29). Additionally, the total liver retinol reserve of study 1 was lower than study 2, i.e. 0·49 and 0·57 μmol retinol/liver, respectively. β-Carotene was found in the livers of study 2 gerbils but not study 1, which indicates more complete bioconversion in study 1 and less available for storage. β-Carotene storage probably indicates adequate VA status and β-carotene will not be stored intact until a threshold of liver reserves has been met(Reference Howe and Tanumihardjo2). In adult gerbils, this threshold occurs between 0·5 and 0·7 μmol retinol/liver. The baseline groups in both studies were at this threshold level of retinol/liver. This may explain why liver reserves were maintained and not increased during treatment with carotenoid supplements and biofortified maize.
Serum retinol concentration, which is highly controlled when liver retinol reserves are >0·07 μmol/g liver(Reference Tanumihardjo31), was normal in all groups (>0·70 μmol/l). In study 1, higher serum retinol was observed in the baseline and control groups compared with the treatment groups despite absence of dietary VA. A similar result was found in study 2, where the baseline serum retinol was higher than the biofortified maize group. Kelley & Green(Reference Kelley and Green32) demonstrated that retinol recycling is extensive before irreversible loss and a retinol molecule will recycle through plasma nine times in rats with adequate liver reserves. As VA intake drops, the number of times retinol is recycled increases(Reference Kelley and Green32), and may lead to a temporary increase in serum retinol.
In study 1, the utilisation rate for the control group was 4·2 μg retinol/100 g BW per d, which is similar to reported values (3·1(Reference Lee, Lederman, Hofmann and Erdman16) and 3·7 μg retinol/100 g BW per d(Reference Tanumihardjo and Howe4)). The utilisation rate for the VA group, which had the highest retinol reserves, was 8·0 μg retinol/100 g BW per d. As retinol reserves increase, utilisation of the vitamin increases(Reference Green, Green and Lewis33). In rats with low, marginal and high retinol status, retinol utilisation was 1·2, 8·0 and 11·8 μg/d per rat, respectively(Reference Green, Green and Lewis33). Thus, the difference in utilisation due to VA status in these gerbils agrees with data in rats. The gerbils were utilising 50–60 % of their daily supplement, which agrees with human data that showed 40 % retention of a [2H]VA dose in the liver(Reference Haskell, Handelman, Peerson, Jones, Rabbi, Awal, Wahed, Mahalanabis and Brown34). The different utilisation between the VA and control groups may have impacted study 1. In rats, plasma retinol is a major determinant of utilisation(Reference Green, Green and Lewis33). Because the serum retinol concentrations of the β-cryptoxanthin and β-carotene groups were the same as the VA group, the impact may not be significant. In addition, the conversion factor calculation is a direct comparison of the total liver retinol of the β-cryptoxanthin and β-carotene groups with the VA group, and not with the control group. Because the conversion factor for β-carotene was similar to that obtained in prior studies(Reference Howe and Tanumihardjo2, Reference Tanumihardjo and Howe4) and supported by results of study 2, the conversion factor for β-cryptoxanthin is valid (Table 3).
β-Cryptoxanthin was bioavailable from supplements and maize in gerbils and was either more efficacious than or as efficacious as β-carotene supplements when intake was equalised. The conversion factor is similar to β-carotene, lower than that for α-carotene(Reference Tanumihardjo and Howe4), and lower than that proposed by the IOM(3). These studies support conclusions drawn from a prior study with biofortified maize which asserts that provitamin A carotenoids other than β-carotene contribute to retinol stores(Reference Howe and Tanumihardjo2). Maize breeders should be encouraged to move forward with maize varieties containing substantial β-cryptoxanthin. Further studies are needed to verify the bioaccessibility of β-cryptoxanthin from additional food matrices, such as citrus fruits and papaya(Reference Breithaupt, Weller, Wolters and Hahn35, Reference O'Connell, Ryan and O'Brien36) which contain free and esterified β-cryptoxanthin, and determine the contribution of dietary β-cryptoxanthin to the retinol pool in humans.
Acknowledgements
The authors have no conflicts of interest with the information presented in this paper. The study was supported by Hatch Wisconsin Agricultural Experiment Station WIS04975 and HarvestPlus contract 2005X059.UWM. C. D. performed study 2; H. J. performed study 1; J. A. H. analysed the maize and oversaw the analytical aspects of the research; T. R. bred, grew and provided the maize; S. A. T. designed the studies and secured support. All authors contributed to manuscript writing and revising.
The authors thank Jordan Mills, Amy Petersen and Emily Nuss for assistance with gerbil care, and Peter Crump, Senior Information Processing Consultant of the University of Wisconsin-Madison College of Agriculture and Life Sciences Statistical Consulting Service, for providing statistical assistance. We are also grateful to Penny Nestel who encouraged us to study the potential of biofortified maize to support VA status and appreciated the need to separately evaluate β-cryptoxanthin.