INTRODUCTION
Many attempts have been made to improve the utilization of feeds by dairy cattle. In recent years, use of feed additives and enzymes in ruminant nutrition have gained elevated interest, e.g. yeast (Elghandour et al. Reference Elghandour, Vázquez Chagoyán, Salem, Kholif, Martínez Castañeda, Camacho and Cerrillo-Soto2014), phytogenic extracts (Cedillo et al. Reference Cedillo, Vázquez-Armijo, González-Reyna, Salem, Kholif, Hernández-Meléndez, Martínez-González, De Oca Jiménez, Rivero and López2014; Salem et al. Reference Salem, Kholif, Elghandour, Buendía, Mariezcurrena, Hernandez and Camacho2014) and fibrolytic enzymes (Alsersy et al. Reference Alsersy, Salem, Borhami, Olivares, Gado, Mariezcurrena, Yacuot, Kholif, El-Adawy and Hernandez2015; Salem et al. Reference Salem, Alsersy, Camacho, El-Adawy, Elghandour, Kholif, Rivero, Alonso and Zaragoza2015; Valdes et al. Reference Valdes, Salem, Lopez, Alonso, Rivero, Elghandour, Domínguez, Ronquillo and Kholif2015). Furthermore, feeding dairy animals on diets supplemented with fibre-degrading enzymes has been shown to improve feed utilization and animal performance (Khattab et al. Reference Khattab, Gado, Kholif, Mansour and Kholif2011; Salem et al. Reference Salem, Gado, Colombatto and Elghandour2013). However, the mode of action of these enzymes has not been fully elucidated.
Hydrolysis of dietary fibre before ingestion (Khattab et al. Reference Khattab, Gado, Kholif, Mansour and Kholif2011), provision of readily fermentable substrates for ruminal micro-organisms (McAllister et al. Reference McAllister, Hristov, Beauchemin, Rode, Cheng, Bedford and Partridge2001) and synergistic enhancement of microbial enzyme activity in the rumen (Morgavi et al. Reference Morgavi, Nsereko, Rode, Beauchemin, McAllister and Wang2000) have been suggested as possible modes of action. In addition, alteration of ruminal fermentation (Salem et al. Reference Salem, Alsersy, Camacho, El-Adawy, Elghandour, Kholif, Rivero, Alonso and Zaragoza2015) and enhanced ruminal micro-organism attachment to and colonization of the plant cell wall (Wang et al. Reference Wang, McAllister, Rode, Beauchemin, Morgavi, Nsereko, Iwaasa and Yang2001) are other possibilities.
Although the results of applying fibrolytic enzymes in dairy cattle rations have been encouraging, they have also been inconsistent. Some studies showed elevated milk production and improved composition (Gado et al. Reference Gado, Salem, Robinson and Hassan2009; Khattab et al. Reference Khattab, Gado, Kholif, Mansour and Kholif2011) while others did not (Elwakeel et al. Reference Elwakeel, Titgemeyer, Johnson, Armendariz and Shirley2007; Dean et al. Reference Dean, Staples, Littell, Kim and Adesogan2013). Although the reasons for this are unknown, some factors such as differences in enzyme activity, application rate and composition, stage of lactation, time of enzyme delivery, ruminal activity and enzyme stability, enzyme-feed specificity and the portion of the diet in which enzymes are applied have been implicated (Dean et al. Reference Dean, Staples, Littell, Kim and Adesogan2013).
The objective of the present study, therefore, was to determine the effects of adding a fibrolytic enzyme cellulase in goat rations on feed intake, ruminal fermentation, nutrient digestibility, milk production and milk composition of French Alpine dairy goats fed a high fibre basal diet.
MATERIALS AND METHODS
All procedures involved in handling animals during the experimental period were conducted according to the official Mexican standards of animals care (NORMA Oficial Mexicana 1995).
Study location
The experiment was performed at the metabolic area of the Animal Science farm, and laboratory of animal nutrition of the Centro Universitario UAEM-Temascaltepec, Universidad Autónoma del Estado de México, México (19°02′04″N, 100°02′14″W, 1720 m a.s.l.). The climate is moderately humid with an average temperature of 15–18 °C and annual rainfall of 950–1000 mm.
Animals, housing and feeding
Twenty-four French Alpine dairy goats (39 ± 2·0 kg initial live weight, 9 months old, first calving) were housed in individual pens provided with automatic feeders and waterers. Immediately after kidding, the goats were adapted to the pens and the basal diet (Table 1) for 10 days before starting the experiment, which lasted for 60 days. Goats were injected with 3 ml/animal of fat-soluble vitamins (A, D, E; Vigantol, Bayer, Mexico City, Mexico) and treated with 0·5 ml Ivermectin (Laboratory Sanfer, Mexico City, Mexico) per animal for internal and external parasites and the health of the animals monitored throughout the experiment.
Table 1. Ingredients and chemical composition of the basal diet, offered to the French Alpine dairy goats during the first third of lactation
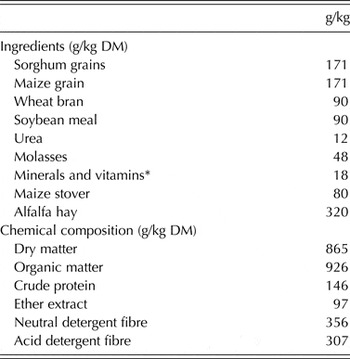
* Mineral/vitamin premix contained: vitamin A (12 000 000 IU), vitamin D3 (2 500 000 IU), vitamin E (15 000 IU), vitamin K (2·0 g), vitamin B1 (2·25 g), vitamin B2 (7·5 g), vitamin B6 (3·5 g), vitamin B12 (20 mg), pantothenic acid (12·5 g), folic acid (1·5 g), biotin (125 mg), niacin (45 g), iron (50 g), zinc (50 g), manganese (110 g), copper (12 g), iodine (0·30 g), selenium (200 mg), cobalt (0·20 g).
A week before the start of the experiment, goats were weighed individually and assigned randomly to two treatments (12 goats per treatment) in a completely randomized design.
The treatments were: CTRL: basal diet without addition of cellulase; CELL: basal diet + Celluase® plus (Dyadic® PLUS, Dyadic international, Inc., Jupiter, FL, USA) at 2 ml/kg dry matter (DM) of feed. The activity of the exogenous fibrolytic enzyme Celluase® plus was determined and contained 30 000–36 000 units of cellulase/g and 7500–10 000 units of beta-glucanase/g.
Goats were offered a total mixed ration (TMR; Table 1) three times daily at 07.00, 13.00 and 19.00 h. The TMR was balanced for minerals and vitamins and formulated to cover the nutrient requirements of goats according to NRC (1981) recommendations plus a margin of 0·15. The daily allocation of enzyme for each goat was mixed individually with the TMR and fed at 07.00 h. Throughout the experimental period, the amount of feed offered was recorded and orts were collected daily and weighed for determination of daily feed intake. Additionally, feeds were sampled daily, composited weekly and dried at 60 °C to constant weight and stored for later chemical analysis.
Nutrient digestibility, rumen fermentation and milk production
All goats from each group were housed individually in stainless steel metabolic cages at room temperature with free access to water during the last 5 days of the experimental period for nutrient digestibility and ruminal fermentation determinations. Beneath each cage were 4 mm stainless steel screens to retain faeces but let the urine through to enable separate and total collection of faeces and urine. Total faeces was collected once daily from each goat before the morning feeding, according to the methodology proposed by Stock et al. (Reference Stock, Brink, Britton, Goedeken, Sindt, Kreikemier, Bauer and Smith1987), and stored at −10 °C for later analysis. A sample of about 150 g of faeces from each animal was taken daily and composited weekly. The composited samples were dried in a forced air oven at 65 °C for 72 h then ground through a 1 mm screen using a Wiley mill grinder (Wiley mill, Model 4, Thomas Scientific, Swedesboro, NJ, USA) and kept for later determination of compositional analysis. Additionally, rumen fluid was taken from the ventral sac of the rumen of each goat using a stomach tube 3 h after the morning feeding. The rumen samples (50 ml/goat) were filtered immediately using four layers of cheesecloth, strained and stored in 45 ml glass bottles. The ruminal pH was then determined using a digital pH meter (GLP 22, Crison Instruments, Barcelona, Spain) and a few drops of toluene and paraffin oil added to each bottle to cover the surface and the bottles stored at −18 °C for later individual volatile fatty acids (VFA) analyses.
Goats were hand-milked daily: milk production was measured in kg (electronic scale; OHAUS Mod. 5603, Mexico City, Mexico) and recorded. Milk was sampled twice weekly and preserved with potassium dichromate. The samples were stored at 4 °C before being sent to the laboratory for compositional analysis.
Chemical analysis and calculations
Dried samples were ground (sieve of 1 mm diameter, Wiley mill, Model 4, Thomas Scientific, Swedesboro, NJ, USA) and analysed for DM (ID 930.15), ash (ID 942.05), ether extract (ID 945.16) and crude protein (CP; ID 984.13) according to AOAC (1997). Collected feed, orts and faecal samples were analysed for neutral detergent fibre (NDF) and acid detergent fibre (ADF) contents (Van Soest et al. Reference Van Soest, Robertson and Lewis1991) using an ANKOM200 Fibre Analyser unit (ANKOM Technology Corporation, Macedon, NY, USA).
The concentrations of acetic, propionic and butyric acids in rumen fluid were quantified as described in Hernandez et al. (Reference Hernandez, Salem, López, Sun, Rojo, Camacho, Elghandour and Ronquillo2014) using crotonic acid as the internal standard using gas chromatography (model 5890, Hewlett Packard, Little Falls, DE, USA) with a capillary column (30 m × 0·25 mm internal diameter, 1 m phase thickness, Supelco Nukol; Sigma–Aldrich, Mississauga, ON, Canada), and flame ionization detection. Oven temperature was adjusted to 100 °C for 1 min, which was then increased by 20 °C/min to 140 °C, and then by 8 °C/min to 200 °C, and held at this temperature for 5 min. The injector temperature was at 200 °C and the detector temperature at 250 °C. The carrier gas was Helium.
Ruminal methane (CH4) production was calculated according to the equation of Moss et al. (Reference Moss, Jouany and Newbold2000) as:
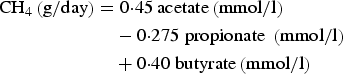
Milk samples were analysed for fat, crude protein and lactose using Milk-O-Scan 605 (Foss Electric, Hillerod, Denmark), which is based on infrared technology. For milk fatty acid profile determination, 150 ml of milk from each goat (experimental unit) were taken and stored at −20 °C and then are lyophilized to make a composited sample for fatty acid profile determination by gas chromatography (model 5890, Hewlett Packard, Little Falls, DE, USA) according to Erwin et al. (Reference Erwin, Marco and Emery1961).
Average fat, crude protein and lactose yields (g/day) were calculated by multiplying milk yield by fat, crude protein and lactose content (g/kg) of milk of each individual goat. The gross energy concentration in milk was calculated according to Tyrell & Reid (Reference Tyrell and Reid1965) as described in Kholif et al. (Reference Kholif, Gouda, Morsy, Salem, Lopez and Kholif2015) as:

The milk energy output was then calculated as milk energy output (MJ/day) = milk energy (MJ/kg) × milk yield (kg/day).
Energy corrected milk (ECM) was calculated according to Sjaunja et al. (Reference Sjaunja, Baevre, Junkkarinen, Pedersen, Setala, Gaillon and Chabert1991) as:

Statistical analyses
The nutrient intake and digestibility, ruminal fermentation parameters and milk yield and composition data were analysed with the PROC MIXED procedure of SAS (SAS Institute 2006) in which a completely randomized design was used with the statistical model:

where Y ij is every observation of the jth goat assigned to ith treatment, T i is the treatment effect and E ij is the residual error.
Comparisons of results were performed using Tukey's test at P < 0·05 (Steel & Torrie Reference Steel and Torrie1980).
RESULTS
Feed intake, digestion and ruminal fermentation
Feed intake (kg/day), digestion (g digested/g ingested) and ruminal fermentation data are summarized in Table 2. Goats fed the CELL diet had greater DM intake (DMI; P = 0·049), organic matter (OM) intake (P = 0·049) and NDF intake (P = 0·049) than CTRL goats (Table 2). The digestibility of DM, OM and NDF for CELL goats was also greater (P < 0·001) than for CTRL goats. Additionally, the ruminal pH for CELL goats was greater (P = 0·013) than for CTRL goats. CELL goats had greater concentration (mmol/l) of acetic acid (P < 0·001) and concentration (mmol/l) of propionic acid (P = 0·007) than the CTRL goats. However, the concentration of ruminal butyric (mmol/l) was lower (P < 0·001) in CELL goats compared with CTRL goats (Table 2).
Table 2. Feed intake, nutrient digestibility and ruminal fermentation of French Alpine dairy goats fed on the basal diet in the absence (CTRL) or presence of cellulase (CELL) enzyme (2 ml/kg DMI) during the first third of lactation (60 days) (n = 12 per treatment)

Milk production and composition
Milk yields (kg/day) and composition (g/kg) are presented in Table 3. CELL goats had greater milk yield (P = 0·042), ECM (P = 0·001), milk energy content (MJ/kg, P = 0·008), milk energy output (MJ/day, P = 0·001) and milk density (g/ml, P = 0·003) than CTRL goats. Additionally, the milk content for total solids (P = 0·008), fat (P = 0·016), protein (P = 0·003) and lactose (P = 0·05) were greater for CELL goats than for the CTRL goats. The same trends were noted for daily total solids (P = 0·001), fat (P = 0·001), protein (P = 0·001) and lactose (P = 0·002) yields. Feeding efficiency expressed as ECM/DMI tended to be greater (P = 0·066) for CELL goats than for CTRL goats; however, when expressed as milk yield/DMI, there were no differences (Table 3).
Table 3. Milk yield and composition of French Alpine dairy goats fed on the basal diet in the absence (CTRL) or presence of cellulase (CELL) enzyme (2 ml/kg DMI) during the first third of lactation (60 days) (n = 12 per treatment)

Milk fatty acid profiles
The milk fatty acid profiles (g/100 g of total esterified fatty acids) are summarized in Table 4. The milk of CELL goats had greater palmitoleic acid (P < 0·001), cis-10-heptadecanoic acid contents (P = 0·021) and mono-saturated fatty acid (P = 0·043) concentrations than the milk of CTRL goats, and lower linoleic acid (P = 0·035), linolenic acid (P = 0·023) and saturated fatty acid content (P = 0·047) than the milk of CTRL goats. CELL goat milk tended to have lower oleic acid (P = 0·073), greater (P = 0·072) capric acid and lower short-chain fatty acids (P = 0·097) than CTRL goat milk (Table 4).
Table 4. Milk fatty acid profile (g/100 g of total fatty acids esterified) of French Alpine dairy goats fed on the basal diet in the absence (CTRL) or presence of cellulase (CELL) enzyme (2 ml/kg DMI) during the first third of lactation (60 days) (n = 12 per treatment)

DISCUSSION
Feed intake
Goats supplemented with CELL consumed about 11% more DM, OM and NDF compared with CTRL goats. Greater nutrient intake was mirrored by greater nutrient (i.e., DM, OM and NDF) digestibility. These results are consistent with the previous results of Salem et al. (Reference Salem, Gado, Colombatto and Elghandour2013), which can be explained by the greater nutrient digestibility, particularly fibre, and Gado et al. (Reference Gado, Salem, Robinson and Hassan2009) obtained 13% more DMI due to enzyme addition in dairy cow diets. This is in contrast to Dean et al. (Reference Dean, Staples, Littell, Kim and Adesogan2013) who observed no difference in feed intake of dairy cows supplemented with fibrolytic enzyme.
Nutrient digestibility
One of the main aims of adding cellulolytic enzymes in ruminant diets is to improve fibre digestion (Alsersy et al. Reference Alsersy, Salem, Borhami, Olivares, Gado, Mariezcurrena, Yacuot, Kholif, El-Adawy and Hernandez2015; Salem et al. Reference Salem, Alsersy, Camacho, El-Adawy, Elghandour, Kholif, Rivero, Alonso and Zaragoza2015). In the present study, greater DM (~4%), OM (~5%) and NDF (~9%) digestibility were obtained with CELL addition compared with CTRL diets. This is consistent with previous studies, which reported greater nutrient digestibility due to enzyme addition (Khattab et al. Reference Khattab, Gado, Kholif, Mansour and Kholif2011; Salem et al. Reference Salem, Gado, Colombatto and Elghandour2013). Although the exact mode of action of the enzyme has not been elucidated, it has been suggested that higher rate of ruminal digestion of the potentially digestible NDF fraction (Yang et al. Reference Yang, Beauchemin and Rode1999), changes in the site of nutrient digestion (Kung et al. Reference Kung, Treacher, Nauman, Smagala, Endres and Cohen2000), changes in gut viscosity (Hristov et al. Reference Hristov, McAllister and Cheng2000), altered ruminal fermentation (Khattab et al. Reference Khattab, Gado, Kholif, Mansour and Kholif2011), enhanced attachment and colonization to the plant cell wall by ruminal micro-organisms (Wang et al. Reference Wang, McAllister, Rode, Beauchemin, Morgavi, Nsereko, Iwaasa and Yang2001) and complementary actions with ruminal enzyme (Morgavi et al. Reference Morgavi, Nsereko, Rode, Beauchemin, McAllister and Wang2000) are possible causes of greater nutrient digestibility.
However, greater fibre digestion is unlikely to be the result of supplemental enzyme activity alone because the contribution of added exogenous enzymes to total ruminal activity is relatively small (Beauchemin et al. Reference Beauchemin, Colombatto, Morgavi, Yang and Rode2004). Moreover, elevated numbers of non-fibrolytic and fibrolytic bacteria in rumen fluid, which elevates microbial biomass, and provides more total polysaccharidase activity to digest feedstuffs (Wang et al. Reference Wang, McAllister, Rode, Beauchemin, Morgavi, Nsereko, Iwaasa and Yang2001; Giraldo et al. Reference Giraldo, Tejido, Ranilla, Ramos and Carro2008) has not been explained. The Celluase® plus product used in the present study contained cellulase and beta-glucanase, which has been shown to have positive effects on digestion of NDF in TMR, although the results are inconsistent. Some studies (Gado et al. Reference Gado, Salem, Robinson and Hassan2009; Khattab et al. Reference Khattab, Gado, Kholif, Mansour and Kholif2011; Salem et al. Reference Salem, Gado, Colombatto and Elghandour2013) reported greater nutrient digestibility, particularly fibre, with enzyme addition to the diets of dairy goats, dairy cows and feedlot cattle, but others did not (Giraldo et al. Reference Giraldo, Tejido, Ranilla, Ramos and Carro2008; Dean et al. Reference Dean, Staples, Littell, Kim and Adesogan2013). Dean et al. (Reference Dean, Staples, Littell, Kim and Adesogan2013) showed that total tract apparent digestibility of DM, NDF and CP were unaffected when the diet of dairy cows was supplemented with fibrolytic enzyme.
Ruminal fermentation
Goats fed the CELL diet showed higher ruminal pH compared with the CTRL goats. Ruminal pH is one of the most important determinants of nutrient digestion particularly fibre digestion, which is modulated via the effect it has on bacterial attachment to fibre substrates because fibrolytic bacteria are very sensitive to ruminal pH changes (Sung et al. Reference Sung, Kobayashi, Chang, Ha, Hwang and Ha2007). Higher ruminal pH is more suitable for fibrolytic microbial activity than low ruminal pH, which changes the rumen microbial population from fibrolytic to amylolytic (Tajima et al. Reference Tajima, Aminov, Nagamine, Matsui, Nakamura and Benno2001). In contrast, Khattab et al. (Reference Khattab, Gado, Kholif, Mansour and Kholif2011) reported no change in ruminal pH due to enzyme addition to the diet of dairy goats.
In the present study, ruminal concentrations of propionic and acetic acids were ~12 and ~6% higher, respectively, whereas butyric acid was ~31% lower for the CELL goats compared with CTRL goats, suggesting improved ruminal fibre fermentation. Gado et al. (Reference Gado, Salem, Robinson and Hassan2009) and Salem et al. (Reference Salem, Gado, Colombatto and Elghandour2013) obtained higher total and individual VFA concentrations when animals were supplemented with enzymes in their diets, suggesting that enzyme addition may alter ruminal fermentation (Khattab et al. Reference Khattab, Gado, Kholif, Mansour and Kholif2011; Salem et al. Reference Salem, Gado, Colombatto and Elghandour2013). The shift in ruminal fermentation may be a result of altered fibre structure, which could stimulate microbial colonization (Giraldo et al. Reference Giraldo, Tejido, Ranilla, Ramos and Carro2008) or a shift in the species profile of fibre-colonizing bacteria as a response to enzyme addition (Wang et al. Reference Wang, McAllister, Rode, Beauchemin, Morgavi, Nsereko, Iwaasa and Yang2001).
The higher concentration of propionic acid in the rumen of CELL goats may also suggest synergism between exogenous enzymes in the feed and lactic acid bacteria in the rumen (Gado et al. Reference Gado, Salem, Robinson and Hassan2009). Higher propionic acid concentration could elevate precursor availability and improve nutrient utilization, particularly for dairy goats in early lactation when nutrient intake lags behind nutrient demand (Eun et al. Reference Eun, Beauchemin and Schulze2007), possibly due to fermentation of sugars released via cell wall hydrolysis by enzymes. The results of the present study suggest that addition of cellulase enzyme in the diet made the fermentation more gluconeogenic, and thereby improved the energetic efficiency of ruminal fermentation. Moreover, the greater concentration of acetic acid in the rumen fluid of CELL goats suggests that CH4 production was also greater, since acetic acid production is associated with the release of hydrogen, which can be used by methanogens to form CH4 (Stewart et al. Reference Stewart, Flint, Byrant, Hobson and Stewart1997) and results in a loss of energy to the host animal (Gado et al. Reference Gado, Salem, Robinson and Hassan2009). However, calculated CH4 production data do not support this hypothesis. Although only a few studies have investigated the effects of exogenous enzymes on CH4 production, the results are conflicting (Togtokhbayar et al. Reference Togtokhbayar, Cerrillo, Rodríguez, Elghandour, Salem, Urankhaich, Jigjidpurev, Odongo and Kholif2015). Although the concentrations of individual VFA, and by extension total VFA, were greater for the CELL goats than the CTRL goats, this result did not affect ruminal pH which was also greater. The reasons for this are unclear.
Milk production and composition
Goats fed CELL diet had 13% (3·44 v. 3·05 kg/day) greater milk production compared with CTRL goats. The same trend was observed for ECM, which was 26% greater (3·29 v. 2·61 kg/day) for CELL goats compared with CTRL goats. Additionally, milk energy content and milk energy output were greater for CELL goats than CTRL goats. The greater milk production is a reflection of greater feed intake, nutrient digestibility and ruminal fermentation activities due to the feeding of enzymes (Gado et al. Reference Gado, Salem, Robinson and Hassan2009; Khattab et al. Reference Khattab, Gado, Kholif, Mansour and Kholif2011). Gado et al. (Reference Gado, Salem, Robinson and Hassan2009) reported 23% greater milk production in dairy cows fed a TMR supplemented with enzymes at 40 g/cow/day for 12 weeks.
In the present study, ruminal concentrations of propionic acid were greater for CELL goats than for CTRL goats. Since propionic acid is the precursor of glucose and lactose, studies suggest that increasing glucogenic precursors resulted in curvilinear elevated milk yield, linear elevated milk protein content and curvilinear decrease in milk fat content (Rigout et al. Reference Rigout, Hurtaud, Lemosquet, Bach and Rulquin2003). This is because propionic acid has greater energy available for milk production (Yang et al. Reference Yang, Beauchemin and Rode1999). Moreover, greater concentration of acetic acid in the ruminal fluid of CELL goats is the main reason for greater milk fat content (Gado et al. Reference Gado, Salem, Robinson and Hassan2009). The greater milk fat content for CELL goats could also be related to the greater digestibility of NDF of this diet (Kholif et al. Reference Kholif, Khattab, El-Shewy, Salem, Kholif, El-Sayed, Gado and Mariezcurrena2014). One of the most surprising results was the greater milk lactose content with enzyme addition. The effect on milk lactose percentage is unusual because the content of milk lactose is typically very constant and changes only slightly (Elwakeel et al. Reference Elwakeel, Titgemeyer, Johnson, Armendariz and Shirley2007). Rumen acetate is the main source for milk short-chain fatty acid synthesis, thereby sparing protein and increasing milk fat and protein content (Fuller Reference Fuller2004). Kholif et al. (Reference Kholif, Khattab, El-Shewy, Salem, Kholif, El-Sayed, Gado and Mariezcurrena2014) showed that elevated milk protein content was related to greater DM, OM and fibre digestibility. In contrast, Dean et al. (Reference Dean, Staples, Littell, Kim and Adesogan2013) did not report any differences in milk production and component yields by enzyme supplementation of the dairy cow diet.
In the present study, addition of enzyme did not alter the concentration of many fatty acids. The milk of CELL goats had greater mono-saturated fatty acids than the milk of CTRL goats and lower saturated fatty acids than the milk of CTRL goats. Milk fatty acids originated mainly from plasma (~60%) or by the de novo synthesis in the mammary gland from acetate and 2-hydroxybutyrate originating as a result of rumen fermentation involving acetyl CoA carboxylase enzymes and fatty acid synthetase (Kholif et al. Reference Kholif, Khattab, El-Shewy, Salem, Kholif, El-Sayed, Gado and Mariezcurrena2014). Ruminants do not synthesize polyunsaturated acids; consequently their concentration in milk depends on the amount absorbed from the intestines. These results in the present study may be due to the altered contents of acetic and propionic acid production in rumen as a result of greater fibre digestion. The direct result of shifted VFA proportions could elevate precursor availability for fatty acid synthesis, particularly during early lactation when nutrient intake lags behind nutrient demand (Eun et al. Reference Eun, Beauchemin and Schulze2007). Gado et al. (Reference Gado, Salem, Robinson and Hassan2009) reported that enzyme supplemented diet showed greater ruminal acetate, propionate and butyrate proportions.
It can be concluded that under the study conditions in the tropical regions of Mexico, addition of exogenous fibrolytic cellulase at 2 ml/kg DM in the diet of lactating French Alpine goats resulted in greater feed intake and nutrient digestibility and improved milk production and composition.
The authors acknowledge financial support from the IAEA, Vienna (Austria), Research Contract number MEX16307 within the D3.10.27 Coordinated Research Project. Kholif, A. E. thanks the National Council for Science and Technology (CONACyT, Mexico) and The World Academy of Sciences (TWAS, Italy) to support his Postdoctoral fellowship at the Facultad de Medicina Veterinaria y Zootecnia, Universidad Autónoma del Estado de México.