Introduction
During the Mesozoic, plesiosaurs constituted a diverse and widely distributed group of marine reptiles. The Jurassic–Cretaceous transition witnessed a faunal turnover amongst marine reptiles, and the plesiosaurian clade, Xenopsaria, radiated rapidly during the Early Cretaceous (Benson & Druckenmiller, Reference Benson and Druckenmiller2014). Xenopsarians comprise two plesiosaur clades, namely the Elasmosauridae Cope, Reference Cope1869a and the Leptocleidia Ketchum & Benson, Reference Ketchum and Benson2010, with the latter including the Polycotylidae (see Benson & Druckenmiller, Reference Benson and Druckenmiller2014).
Members of the family Elasmosauridae were still widespread and diverse during the latest Maastrichtian, as exemplified by Zarafasaura from phosphatic strata of Morocco (Lomax & Wahl, Reference Lomax and Wahl2013; Vincent et al., Reference Vincent, Bardet, Houssaye, Amaghzaz and Meslouh2013), as well as by material from Chile (Otero et al., Reference Otero, Soto-Acuña, O’Keefe, O’Gorman, Stinnesbeck, Suárez, Rubilar-Rogers, Salazar and Quinzio-Sinn2014), Argentina (O’Gorman et al., Reference O’Gorman, Gasparini and Salgado2014), Antarctica (O’Gorman & Coria, Reference O’Gorman and Coria2016) and Arctic regions of Eurasia (Zverkov et al., Reference Zverkov, Rogov, Zakharov, Danilov, Grigoriev and Košťák2023) and North-America (Russell, Reference Russell1967). Until recently, only two polycotylid clades appeared to have ranged into the early Maastrichtian, namely Occultonectia and Polycotylinae (Mulder et al., Reference Mulder, Bardet, Godefroit and Jagt2000; Sato, Reference Sato2005; O’Gorman & Gasparini, Reference O’Gorman and Gasparini2013; Fischer et al., Reference Fischer, Benson, Druckenmiller, Ketchum and Bardet2018). Recently, a new polycotylid with an elongated snout and remarkably long neck from the lower Maastrichtian of the United States has been added to the latest Cretaceous record of this family (Persons et al., Reference Persons, Street and Kelley2022), while Clark et al. (Reference Clark, O’Keefe and Slack2024) additionally introduced a new clade of derived polycotylids that ranged into the Maastrichtian, the Dolichorhynchia. Representatives of the family Polycotylidae Williston, Reference Williston1908 in general had short, stout necks and an elongated rostrum (Druckenmiller & Russell, Reference Druckenmiller and Russell2008; Frey et al., Reference Frey, Mulder, Stinnesbeck, Rivera-Sylva, Padilla-Gutiérrez and González-González2017; Fischer et al., Reference Fischer, Benson, Druckenmiller, Ketchum and Bardet2018). Similar to polycotylids, elasmosaurids possessed numerous pointy teeth, but had extremely long necks and a short rostrum (Everhart, Reference Everhart2006; Kubo et al., Reference Kubo, Mitchell and Henderson2012; Brum et al., Reference Brum, Simões, Souza, Pinheiro, Figueiredo, Caldwell, Sayão and Kellner2022). Both groups had four morphologically similar flippers that enabled them to move efficiently through the water using all limbs simultaneously, a way of propulsion also used by marine turtles and penguins often referred to as subaqueous flight or ‘flying under water’ (Robinson, Reference Robinson1975; Massare, Reference Massare, Maddock, Bone and Rayner1994; Caldwell, Reference Caldwell1997; Carpenter & Sanders, Reference Carpenter and Sanders2010; Muscutt et al., Reference Muscutt, Dyke, Weymouth, Naish, Palmer and Ganapathisubramani2017). However, alternative modes of locomotion such as rowing have been suggested for plesiosaurs as well (Araújo et al., Reference Araújo, Polcyn, Schulp, Mateus, Jacobs, Gonçalves and Morais2015b).
The Maastrichtian type area (south-east Netherlands and contiguous regions in Belgium and Germany; Fig. 1) has long been known to yield both articulated and dissociated skeletal remains of mosasaurs and cheloniid turtles (Kuypers et al., Reference Kuypers, Jagt, Peeters and De Graaf1998; Dortangs et al., Reference Dortangs, Schulp, Mulder, Jagt, Peeters and de Graaf2002; Janssen et al., Reference Janssen, Van Baal, Schulp, Jagt, Jagt-Yazykova and Schins2011; Bastiaans et al., Reference Bastiaans, Kroll, Cornelissen, Jagt and Schulp2020). Compared to the common occurrence of mosasaur and marine turtle remains throughout the upper part of the Gulpen Formation (Lanaye Member) and all members of the overlying Maastricht Formation, the extreme paucity of plesiosaur remains in the area is remarkable (Mulder et al., Reference Mulder, Bardet, Godefroit and Jagt2000; Schulp et al., Reference Schulp, Janssen, Van Baal, Jagt, Mulder and Vonhof2016; Miedema et al., Reference Miedema, Schulp, Jagt and Mulder2019). To date, only a handful of teeth and eight vertebrae from the Maastrichtian type area have been assigned to the Elasmosauridae (Roos, Reference Roos1966; Mulder, Reference Mulder1990; Mulder et al., Reference Mulder, Bardet, Godefroit and Jagt2000; Renkens, Reference Renkens2023). A small caudal vertebra from the upper portion of the Maastricht Formation (Emael Member) in the Sibbe area (near Valkenburg aan de Geul, the Netherlands) is the most recent addition (Miedema et al., Reference Miedema, Schulp, Jagt and Mulder2019). As far as we are aware, no remains of polycotylids have yet been identified in these strata.

Figure 1. Map of southern Limburg (the Netherlands) and contiguous areas in northeastern Belgium and the Aachen area (Germany) with localities that have yielded elasmosaur skeletal remains to date (data from Mulder et al., Reference Mulder, Bardet, Godefroit and Jagt2000; Miedema et al., Reference Miedema, Schulp, Jagt and Mulder2019; Renkens, Reference Renkens2023; J.W.M. Jagt, pers. obs.).
Here we add an additional plesiosaur find from the Maastrichtian type area, consisting of a single, isolated, yet well-preserved phalanx from the basal Emael Member (Maastricht Formation) at the former ENCI-HeidelbergCement Group quarry (Sint-Pietersberg, Maastricht). We refer the specimen to a generically and specifically indeterminate plesiosaur, adding to the meagre plesiosaur record previously consisting of isolated teeth and vertebrae only.
Geological setting and stratigraphy
The phalanx described herein (collections of the Natuurhistorisch Museum Maastricht, NHMM 2022 009, leg. J.H.M. Barten), discovered in April 2016, originates from the fossiliferous basal lag of the Emael Member (Maastricht Formation), which rests on the Romontbos Horizon, as exposed in the north-western corner of the former ENCI-HeidelbergCement Group quarry (Fig. 2; Table 1). According to the most recent chemostratigraphical age model for the type Maastrichtian (Vellekoop et al., Reference Vellekoop, Kaskes, Sinnesael, Huygh, Déhais, Jagt, Speijer and Claeys2022), NHMM 2022 009 is around 66.55 million years old. Strata assigned to the Emael Member were laid down in a fully marine environment, although there must have been nearby landmasses, as is indicated by the discovery of terrestrial plants (Jagt & Jagt-Yazykova, Reference Jagt and Jagt-Yazykova2012; Vellekoop et al., Reference Vellekoop, Kaskes, Sinnesael, Huygh, Déhais, Jagt, Speijer and Claeys2022).

Figure 2. Photograph of the north-western corner of the former ENCI-HeidelbergCement Group quarry (Sint-Pietersberg, Maastricht) with good exposures of nearly all members of the Maastricht Formation and site of provenance of NHMM 2022 009 (indicated by a blue arrow). Photograph: M.J.M. Deckers.
Table 1. Local lithostratigraphy (after Jagt & Jagt-Yazykova, Reference Jagt and Jagt-Yazykova2012), with the indication of levels that have yielded elasmosaurid finds (and numbers of specimens) to date and dating in millions of years (Ma) after Vellekoop et al. (Reference Vellekoop, Kaskes, Sinnesael, Huygh, Déhais, Jagt, Speijer and Claeys2022). Note: the Kunrade Formation is currently regarded to correspond to the uppermost Gulpen Formation (Lanaye Member) up to the basal Emael Member

Institutional abbreviations
NHMM: Natuurhistorisch Museum Maastricht, Maastricht, the Netherlands.
Systematic palaeontology
Sauropterygia Owen, Reference Owen1860
Plesiosauria de Blainville, Reference Blainville1835
Plesiosauroidea Welles, Reference Welles1943
Plesiosauroidea gen. et sp. indet
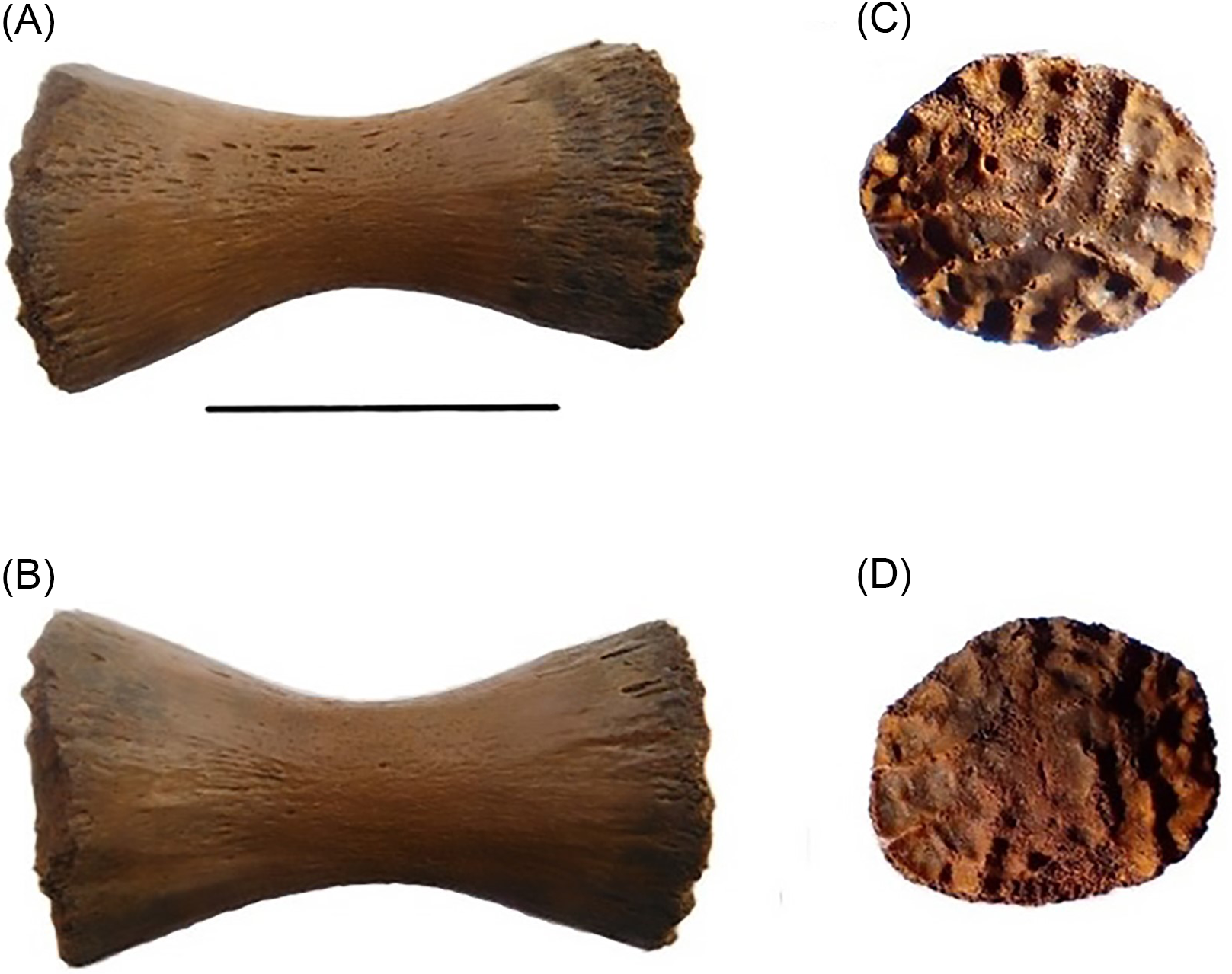
Figure 3. NHMM 2022 009, a plesiosaur phalanx from the basal Emael Member (Maastricht Formation) of the former ENCI-HeidelbergCement Group quarry (Sint-Pietersberg, Maastricht). Dorsal or ventral views (A, B) and proximal and distal views of the articular facets (C, D). Scale bar equals 40 mm. Photographs: L.P.J. Barten.

Figure 4. Comparison of phalanx NHMM 2022 009 (Q–T) from the basal Emael Member (Maastricht Formation; see also Fig. 3) of the former ENCI-HeidelbergCement Group quarry (Sint-Pietersberg, Maastricht) with a selection of mosasaur phalanges (A–P) of a subadult individual of Mosasaurus hoffmanni Mantell, Reference Mantell1829 (NHMM 2015 027) from the same member (above Lava Horizon). Scale bar equals 40 mm. Photographs: L.P.J. Barten.
Material
A single isolated phalanx (NHMM 2022 009) from the basal Emael Member (Maastricht Formation) at the former ENCI-HeidelbergCement Group quarry, south of Maastricht, the Netherlands.
Description
Specimen NHMM 2022 009, here identified as an isolated phalanx of a generically and specifically indeterminate plesiosaur, measures 63 mm in proximo-distal length and 34 and 32 mm in extension at the articular facets (Fig. 3). In fact, these articular facets are rough articular subchondral surfaces (compare Valente et al., Reference Valente, Marco, Zamora, Parga, Lavín, Alegre and Cuenca2007, figs 2, 4; Snover & Rhodin, Reference Snover, Rhodin, Wyneken, Godfrey and Bels2008, figs 2.3, 2.23B), which could indicate osteological immaturity of the animal from which NHMM 2022 009 originated. The morphology of the phalanx could also reflect a state of paedomorphism, as often seen in elasmosaurids (compare Araújo et al., Reference Araújo, Polcyn, Lindgren, Jacobs, Schulp, Mateus, Olímpio Gonçalves and Morais2015a, p. 106). Lastly, NHMM 2022 009 might represent one of the distalmost, poorly ossified phalanges because distal limb bones in general show delayed ossification (Caldwell, Reference Caldwell1997).
The circumference around the mid-shaft measures 17 mm (Fig. 3). The bone is hourglass-shaped and well-preserved and does not reveal any traces of transport and erosion, with the exception of minor damage near the articular facets (articular subchondral surfaces). Both these surfaces are near circular in outline and are slightly convex with a highly rugose bone structure consisting of irregularly distributed bony ridges (Fig. 3C, D). Between these ridges, some less ossified areas are seen with a more open bone structure, and both surfaces display foramina. The latter are here interpreted to indicate transphyseal vascular channels that penetrated into the covering cartilage in vivo, comparable to the phalangeal anatomy as is seen in some extant reptiles, for example, leatherback turtles (Snover & Rhodin, Reference Snover, Rhodin, Wyneken, Godfrey and Bels2008). The bone surface of the shaft of NHMM 2022 009 is smooth, but does contain numerous small pores, as well as a few larger ones. Since phalanges of the adjoining finger and toe bones were closely connected in plesiosaurs and were likely held together in a layer of tendons and ligaments (Everhart, Reference Everhart2017), these pores might indicate foramina of vascular canals and attachment points of ligaments (see Snover & Rhodin, Reference Snover, Rhodin, Wyneken, Godfrey and Bels2008). Towards the edges of the articular subchondral surfaces, the bone surface of the shaft becomes slightly rougher.
Discussion and conclusions
Identification of the phalanx
In stark contrast to teeth, phalanges of the majority of Mesozoic marine reptiles (with the exception of the elongated and slender phalanges of sea turtles and circular or elliptical phalanges of ichthyosaurs) tend to look quite similar and are therefore comparatively undiagnostic. In view of this, very detailed descriptions of elasmosaurid digits are few (although they are depicted in some papers, for example, Williston, Reference Williston1906; Welles, Reference Welles1943, Reference Welles1952, Reference Welles1962; Sato & Storrs, Reference Sato and Storrs2000; Everhart, Reference Everhart2006; Sato et al., Reference Sato, Hasegawa and Manabe2006; Otero et al., Reference Otero, Soto-Acuña, O’Keefe, O’Gorman, Stinnesbeck, Suárez, Rubilar-Rogers, Salazar and Quinzio-Sinn2014, Sachs & Kear, Reference Sachs and Kear2017) and they do not serve as a key element in generic and specific identification. However, robust plesiosaur phalanges displaying convex articular subchondral surfaces, including a rugose bone texture with foramina, have been observed in plesiosaurs of Jurassic age, for instance in the genus Cryptoclidus Seeley, Reference Seeley1892, and also in taxa from the Lower Cretaceous of Russia and the Upper Cretaceous of Angola (Hawthorne et al., Reference Hawthorne, McMenamin and De la Salle2019, fig. 12; Fischer et al., Reference Fischer, Zverkov, Arkhangelsky, Stenshin, Blagovetshensky and Uspensky2021, fig. 8J, K; Araújo et al., Reference Araújo, Polcyn, Lindgren, Jacobs, Schulp, Mateus, Olímpio Gonçalves and Morais2015a, fig. 3E, G). Nevertheless, to our knowledge, juvenile and/or paedomorphic features such as the subchondral texture on the articular facets of NHMM 2022 009 have not yet been observed in mosasaur and extinct turtle digits. In consideration of its size, the presence of these features in phalanx NHMM 2022 009 is unique and hints at a plesiosaurian origin. Moreover, it is less constricted in the median portion than a mosasaur phalanx of the same size (Fig. 4), and the articular facets are near circular in outline, in contrast to the articular surfaces of phalanges of Mosasaurus hoffmanni Mantell, Reference Mantell1829 (NHMM collections) that have a more rectangular or elliptical outline (Fig. 4).
During the late Maastrichtian, the sole surviving lineages of Plesiosauroidea comprised members of the families Polycotylidae and Elasmosauridae, both belonging to the Xenopsaria (Ketchum & Benson, Reference Ketchum and Benson2010; Benson & Druckenmiller, Reference Benson and Druckenmiller2014). During the evolution of the Polycotylidae, the podials (including phalanges) became increasingly shorter and wider over time; early polycotylids tend to have longer and slenderer limb elements than the highly derived species that possessed short and blocky phalanges (Schumacher, Reference Schumacher2007). Phalanges from a polycotylid found in Mexico and illustrated and described in detail by Frey et al. (Reference Frey, Mulder, Stinnesbeck, Rivera-Sylva, Padilla-Gutiérrez and González-González2017) are characterised by an hourglass shape and furthermore possess an almost straight interphalangeal articulation surface. The length/breadth ratio of the phalanges is around 1.8 on average and the appearance of the bones is robust and short. Phalanges of other polycotylids such as Trinacromerium bonneri Adams, Reference Adams1997 and Polycotylus latipinnis Cope, Reference Cope1869b from the Western Interior Seaway are also much shorter, more robust and less constricted than NHMM 2022 009 (Adams, Reference Adams1997, figs 11B, 13; Schumacher & Martin, Reference Schumacher and Martin2015, fig. 13). The polycotylid Palmulasaurus quadratus (see Albright et al., Reference Albright, Gillette and Titus2007b) from the Upper Cretaceous (lowermost Turonian) Tropic Shale of southern Utah shows short and blocky proximal phalanges as well, with concave anterior and posterior surfaces (Albright et al., Reference Albright, Gillette and Titus2007a, fig. 5). However, the distal, more elongated phalanges of Palmulasaurus quadratus do resemble the morphology of NHMM 2022 009.
NHMM 2022 009 has convex interphalangeal articulation surfaces and is more elongate in shape and less constricted in the middle portion than most of the above-mentioned polycotylid phalanges. NHMM 2022 009 is of late Maastrichtian age and does not match the typical blocky morphology of highly derived polycotylid phalanges well. O’Gorman (Reference O’Gorman2020) noted that phalanges in typical elasmosaurs such as Albertonectes vanderveldei Kubo et al. (Reference Kubo, Mitchell and Henderson2012) were quite short with a length/breadth ratio smaller than 2. This corresponds to the dimensions of NHMM 2022 009. In addition to this, there is no firm evidence of polycotylids in the Maastrichtian type area (Miedema et al., Reference Miedema, Schulp, Jagt and Mulder2019). Taking this into consideration, we regard the present specimen to show a closest affinity to autopodial elements of members of the family Elasmosauridae. However, caution is called for in assigning a relatively undiagnostic bone, such as this isolated phalanx, with certainty to any particular family within the Xenopsaria, especially since the morphology of autopodial elements in (xenopsarian) plesiosaurs remains an understudied aspect of most skeletal descriptions in the literature.
Structure-function relationship of rugose articular surfaces
As noted above, the articular surfaces (articular subchondral surfaces) of the present phalanx are characterised by a highly rugose bone structure consisting of irregular ridges (Fig. 3C, D). These features imply an in vivo cartilaginous cover structure, conducive to the smooth functioning of interphalangeal joints, that had not yet attached to the bone properly – such would have occurred in juvenile animals (Hall, Reference Hall2005). Propodials and distal limb bones of juvenile plesiosaurs show a lack of articular facets and a low degree of ossification of the articular surfaces (Wahl, Reference Wahl2006; Kear, Reference Kear2007). This does not apply to (sub-)adult plesiosaurs. One of the most notable examples of a subadult Early Cretaceous plesiosaur is the holotype of Brancasaurus brancai Wegner, Reference Wegner1914 from western Germany (Geologisch-Paläontologisches Museum Münster, A3.B4). The specimen possesses well-defined epipodial facets, which can be interpreted as an adult (or subadult) osteological feature (Sachs et al., Reference Sachs, Hornung and Kear2016). NHMM 2022 009 does not possess well-defined articulation facets. The ossification is clearly incomplete and hence the articular surfaces lack full definition.
It should be noted in this respect that several authors have reported osteologically immature plesiosaur individuals that had reached maturity (O’Gorman et al., Reference O’Gorman, Gasparini and Salgado2014; Araújo et al., Reference Araújo, Polcyn, Lindgren, Jacobs, Schulp, Mateus, Olímpio Gonçalves and Morais2015a; Araújo & Smith, Reference Araújo and Smith2023). This paedomorphism hampers any assessment of the ontogenetic stage of plesiosaurs, especially where isolated finds such as the present phalanx are concerned, under consideration of its size.
The paradox that becomes evident when dealing with paedomorphic plesiosaur individuals might be explained by rapid growth towards maturity. Such a phenomenon is seen in the extant leatherback, Dermochelys coriacea (Vandellius, Reference Vandellius1761). The ‘phenomenally rapid growth’ (Snover & Rhodin, Reference Snover, Rhodin, Wyneken, Godfrey and Bels2008, p. 23) of this turtle is, amongst other skeletal characters, connected with the chondro-osseous development of the phalanges, which show anatomical structures that are identical to those seen in NHMM 2022 009 (Snover & Rhodin, Reference Snover, Rhodin, Wyneken, Godfrey and Bels2008, pp. 22, 23, figs 2.3, 2.23 B). Proximal and distal ends of limb bones of young leatherbacks are covered with a thick, well-vascularised cartilaginous structure. The supply of nutrients via the well-vascularised cartilaginous joint capsules allows rapid accretion of new bone tissue (Rhodin et al., Reference Rhodin, Ogden and Conlogue1981; Snover & Rhodin, Reference Snover, Rhodin, Wyneken, Godfrey and Bels2008). When the animal has reached an advanced age and full body size, most of the ossification of the articular surfaces takes place, replacing the cartilage structure (Rhodin et al., Reference Rhodin, Ogden and Conlogue1981; Snover & Rhodin, Reference Snover, Rhodin, Wyneken, Godfrey and Bels2008). The presence of convex articular subchondral surfaces, including a rugose bone structure with foramina as seen in autopodials of some plesiosaurids (e.g. Araújo et al., Reference Araújo, Polcyn, Lindgren, Jacobs, Schulp, Mateus, Olímpio Gonçalves and Morais2015a; Hawthorne et al., Reference Hawthorne, McMenamin and De la Salle2019; Fischer et al., Reference Fischer, Zverkov, Arkhangelsky, Stenshin, Blagovetshensky and Uspensky2021), might be indicative of some sort of chondro-osseous bone formation in these plesiosaurs.
Caldwell (Reference Caldwell1997) recorded delayed ossification of distalmost limb bones in plesiosaurs, which could also have led to the remarkable morphological features observed in NHMM 2022 009. In obligatorily aquatic marine mammals such as odontocete cetaceans, paedomorphic features such as a delayed ossification of carpals, metacarpals and phalanges have also been reported (Mellor et al., Reference Mellor, Cooper, De La Torre and Brownell2009). In that respect, the morphology of the articular subchrondral surfaces of NHMM 2022 009 might also reflect the presence of fully active epiphyses (growth discs) positioned on top of the shaft, as seen in both juveniles and adult mammals showcasing paedomorphosis. Ossification patterns observed in the limbs of odontocete mammals may thus be similar to those of extinct marine reptiles such as plesiosaurs, as mentioned by Dawson (Reference Dawson2003). However, this particular phalanx is of a rather large size for a distal location in the flipper; when compared to paedomorphic phalanges from Angolan elasmosaurids (Araújo et al., Reference Araújo, Polcyn, Lindgren, Jacobs, Schulp, Mateus, Olímpio Gonçalves and Morais2015a, fig. 3E, G) or to the proximal phalanges of a juvenile elasmosaurid from New Zealand (Otero et al., Reference Otero, O’Gorman, Moisley, Terezow and McKee2018, fig. 9E), the relatively large dimensions of the phalanx from Maastricht are striking. The present specimen with its typical articular subchrondral surfaces thus most likely originates from either a large juvenile animal or a paedomorphic adult plesiosaur. In order to assess the relative age of the individual this phalanx came from, future palaeohistological and CT scan-based analysis may be called for.
Previous plesiosaur finds from the Maastrichtian type area
All currently known plesiosaurian bone material from the study area stems from the upper part of the Maastricht Formation (Table 1), specifically from the Emael and Nekum Members (Mulder et al., Reference Mulder, Bardet, Godefroit and Jagt2000; Miedema et al., Reference Miedema, Schulp, Jagt and Mulder2019). The four cervical vertebrae (NHMM 1985141, NHMM MND K 20.01.801a, NHMM MND KB 50.20.01 and NHMM MND K 20.01.801b), all from the former ‘t Rooth or Nekami quarry (now Sibelco; Bemelen, the Netherlands), probably originated from the Nekum Member, because the quarrying activities at that locality mostly concerned this unit. Thus, we conclude that the present phalanx from the basal Emael Member is in accordance with the stratigraphical origin of other known plesiosaurian bone material from the Maastrichtian type area.
The relative abundance of large aquatic reptiles such as mosasaurs and marine turtles (Janssen et al., Reference Janssen, Van Baal, Schulp, Jagt, Jagt-Yazykova and Schins2011, Reference Janssen, Van Baal and Schulp2017; Schulp et al., Reference Schulp, Janssen, Van Baal, Jagt, Mulder and Vonhof2016), which are both well-represented in the fossil record of the type Maastrichtian, is even more remarkable when the paucity of elasmosaurs is considered. Several species of mosasaurs are quite common, for example, Mosasaurus hoffmanni and Plioplatecarpus marshi Dollo, Reference Dollo1882, while globidensines such as Prognathodon saturator Dortangs et al., Reference Dortangs, Schulp, Mulder, Jagt, Peeters and de Graaf2002 and Carinodens belgicus (Woodward, Reference Woodward1891) are rarer in the same strata (Schulp et al., Reference Schulp, Vonhof, Van der Lubbe, Janssen and Van Baal2013). Schulp et al. (Reference Schulp, Janssen, Van Baal, Jagt, Mulder and Vonhof2016) performed a study on stable isotopes in tooth enamel of mosasaur taxa from the type Maastrichtian, as well as two elasmosaurid teeth from the area. They concluded that there was no clear difference in isotope values between teeth of elasmosaurids and of mosasaurs and, as a consequence, their diving behaviour must have been comparatively similar if not identical. The paucity of elasmosaurids in these strata probably was due to a larger-scale absence of plesiosaurs in the epicontinental sea that covered Europe during the Maastrichtian, as Strganac et al. (Reference Strganac, Jacobs, Polcyn, Mateus, Myers, Salminen, May, Araújo, Ferguson, Gonçalves, Morais, Schulp and Da Silva Tavares2015) found Angolan elasmosaurids to forage far away from shore, based on their isotopic carbon signature.
As mentioned by Miedema et al. (Reference Miedema, Schulp, Jagt and Mulder2019) and Strganac et al. (Reference Strganac, Jacobs, Polcyn, Mateus, Myers, Salminen, May, Araújo, Ferguson, Gonçalves, Morais, Schulp and Da Silva Tavares2015), Maastrichtian marine reptile assemblages in regions that are linked to oceanic upwelling, for instance because these areas were located near steep continental slopes or influenced by Hadley cells, are characterised by a relatively common occurrence of elasmosaurids, accompanied by abundant mosasaur remains. It has been suggested that oceanic upwelling of nutrient-enriched, cold water from the deep sea near continental slopes, in combination with a high primary productivity leading to abundant prey, would allow both plesiosaurs and mosasaurs to coexist (Miedema et al., Reference Miedema, Schulp, Jagt and Mulder2019), as is illustrated by bone beds rich in both plesiosaurs and mosasaurs from the Maastrichtian of Angola (Strganac et al., Reference Strganac, Jacobs, Polcyn, Mateus, Myers, Salminen, May, Araújo, Ferguson, Gonçalves, Morais, Schulp and Da Silva Tavares2015; Jacobs et al., Reference Jacobs, Polcyn, Mateus, Schulp, Gonçalves and Morais2016). Several taxa of mosasaurs (e.g. Prognathodon and Mosasaurus) often were the dominant apex predators in shallower, epicontinental seas where oceanic upwelling did not occur, such as the Maastrichtian type area (Schulp et al., Reference Schulp, Janssen, Van Baal, Jagt, Mulder and Vonhof2016).
There are localities in the Northern Hemisphere that apparently were more suitable for plesiosaur inhabitation during the Late (or latest) Cretaceous, as is illustrated by occurrences of elasmosaur species in Campanian strata of southern Sweden (Einarsson et al., Reference Einarsson, Lindgren, Kear and Siverson2010; Sørensen et al., Reference Sørensen, Surlyk and Lindgren2013) and Canada (Kubo et al., Reference Kubo, Mitchell and Henderson2012) and also in Maastrichtian sedimentary rocks in Morocco (Mulder et al., Reference Mulder, Bardet, Godefroit and Jagt2000; Lomax & Wahl, Reference Lomax and Wahl2013), the US states of Montana (Serratos et al., Reference Serratos, Druckenmiller and Benson2017) and California (Welles, Reference Welles1943; O’Gorman, Reference O’Gorman2016).
The extreme paucity of plesiosaur remains in the shallow epicontinental chalk sea of the Maastrichtian type area and the isolated nature of currently recovered bones and teeth demonstrate that plesiosaurs preferred other habitats during the late Maastrichtian. Xenopsarian plesiosaurs may be considered to have been occasional guests in the shallow epicontinental sea during this time, either as live animals or as floating carcasses. With the new chemostratigraphical calibration of the type Maastrichtian strata (Vellekoop et al., Reference Vellekoop, Kaskes, Sinnesael, Huygh, Déhais, Jagt, Speijer and Claeys2022), we may now also date the meagre plesiosaur record from the study area, close to the Cretaceous-Paleogene (K/Pg) boundary. The phalanx described here therefore provides a valuable new data point in the scant type Maastrichtian plesiosaur record that contributes to resolving the enigma surrounding the rarity of plesiosaur material from this locality.
Acknowledgements
We thank Jos Barten for donating the specimen to the Natuurhistorisch Museum Maastricht collections. Furthermore, we are grateful to Sven Sachs, José O’Gorman, Valentin Fischer and Nikolay Zverkov for their help with the identification of the present specimen; to Valentin Fischer for insightful comments on a previous version of the typescript, to Eberhard (‘Dino’) Frey and two anonymous reviewers for valuable pointers and to the management of the former ENCI-HeidelbergCement Group quarry for granting access to their quarry grounds over the past decades.
Competing interests
The corresponding author declares none, nor does the second author, Eric Mulder. The third author, John Jagt, declares that he is one of the guest editors of the present celebratory issue on the 175th anniversary of the Maastrichtian Stage.