Introduction
Arthropods account for more than 80% of animal species, among which litter-dwelling invertebrates account for a large proportion of the world’s biodiversity (Ødegaard Reference Ødegaard2000). They represent important food sources for many vertebrates and other predatory invertebrates (Pianka and Parker Reference Pianka and Parker1975; Redford Reference Redford and Genoways1987; McNabb et al. Reference McNabb, Halaj and Wise2001). Beetles (Coleoptera) are among the most diverse group of arthropods (Hammond Reference Hammond1992; Rosenzweig Reference Rosenzweig1995), live in a variety of habitats, and have a wide diet spectrum, from plant leaves, tree sap, animals, fungi to dead or decaying wood (Cott Reference Cott1940; Ruppert et al. Reference Ruppert, Barnes, Fox, Ruppert, Barnes and Fox2004). They play an important role in forest ecosystem services, recycling organic components and nutrients, affecting energy flow, and so on (Giller Reference Giller1996; Artz et al. Reference Artz, Anastasiou, Arrouays, Bastos, Bendetti and Bispo2010; Roy et al. Reference Roy, Roy, Jaiswal and Baitha2018).
Leaf litter is an important environment, harbouring many organisms, where interactions between litter-dwelling insects and the environment are ecologically important (Moore et al. Reference Moore, Walter and Hunt1988; Hughes et al. Reference Hughes, Daily and Ehrlich2000; Jeffery and Gardi Reference Jeffery and Gardi2010). As leaf litter decomposes, it releases nutrients and moisture, promoting fungal growth and sporulation (Kerekes et al. Reference Kerekes, Kaspari, Stevenson, Nilsson, Hartmann, Amend and Bruns2013; Krishna and Mohan Reference Krishna and Mohan2017). Litter-dwelling arthropods use this nutrient-rich environment and interact with each other (Seastedt and Crossley Reference Seastedt and Crossley1984). Leaf litter creates a variety of soil microhabitats that contain food resources, and it protects organisms from poor weather conditions and predators (Hamilton Reference Hamilton2015). These microhabitats vary throughout the year and affect the availability of nutritional resources for species belonging to different feeding guilds (Fittkau and Klinge Reference Fittkau and Klinge1973; Hättenschwiler et al. Reference Hättenschwiler, Tiunov and Scheu2005).
Changes in season or temperature affect the diversity and abundance of adult beetles that reside in leaf litter and soil (Pinheiro et al. Reference Pinheiro, Diniz, Coelho and Bandeira2002). The response of beetles to these changes differs worldwide, especially between temperate and tropical zones (Wolda Reference Wolda1978a, Reference Wolda1978b, Reference Wolda1980; Wolda and Broadhead Reference Wolda and Broadhead1985; McElravy and Resh Reference McElravy and Resh1987). In the temperate zone, where there are four seasons, for example, different species are active in different seasons (Scott and Epstein Reference Scott and Epstein1987). Summer in temperate regions is hot and humid, and the days are long. These environmental conditions are expected to increase the activity of adult beetles and increase their diversity and abundance (Whittaker and Tribe Reference Whittaker and Tribe1998; Frazier et al. Reference Frazier, Huey and Berrigan2006; Deutsch et al. Reference Deutsch, Tewksbury, Huey, Sheldon, Ghalambor, Haak and Martin2008). Conversely, temperate-zone winters are cold and dry, with a short photoperiod, leading to decreased activity by adult beetles and a decline in species diversity and abundance (Mellanby Reference Mellanby1939; Taylor Reference Taylor1963; Wolda Reference Wolda1988). Unfavourable cold environments also cause many insects to delay their development (Tauber et al. Reference Tauber, Tauber and Masaki1986; Saunders Reference Saunders2002), and many beetles enter diapause in an adult stage for winter (Danks Reference Danks1987). Most adult beetles are sensitive to these environmental changes, and thus monitoring changes in diversity, abundance, and community structures of these organisms is highly important for biodiversity conservation (Agosti et al. Reference Agosti, Majer, Alonso and Schultz2000; Hilty and Merenlender Reference Hilty and Merenlender2000; Kime and Golovatch Reference Kime and Golovatch2000; Longcore Reference Longcore2003; Bouyer et al. Reference Bouyer, Sana, Samandoulgou, Cesar, Guerrini, Kabore-Zoungrana and Dulieu2007; Siddig et al. Reference Siddig, Ellison, Ochs, Villar-Leeman and Lau2016).
Several studies in Korea have investigated seasonal changes in insect biodiversity, including adult beetles. However, these studies have limited sampling periods and usually exclude the winter season. Furthermore, pitfall traps, light traps, or sweeping methods were typically used to collect insects (Lee et al. Reference Lee, Jeong, Choi and Kwon2005; Byun et al. Reference Byun, Lee, Park, Kim and Jo2009a, Reference Byun, Lee, Park, Lee and Jo2009b, Reference Byun, Lim, Park, Kim, Lee and Jo2010; Lee et al. Reference Lee, Byun, Park, Lim and Jo2009; Park et al. Reference Park, Cho, Kim, Han, Lim, Park and Hong2010; Park et al. Reference Park, Lim, Jo and Lee2011; Lim et al. Reference Lim, Park, Lee and Jo2012; Kim and Kwon Reference Kim and Kwon2016). In the present study, we combined a sifting technique with Berlese funnel extraction to collect samples of litter-dwelling arthropods during all seasons because of the limited capability of other trapping methods for sampling overwintering arthropods. Because Berlese funnels direct constant high temperature and light towards litter samples, they can provide a strong signal to awaken insects from diapause (Saunders Reference Saunders2002). In this way, the use of Berlese funnels has the potential to collect insects and other soil arthropods in the winter season.
Few studies have addressed the full seasonal spectrum of litter-dwelling animals and their relationships with the environment. The present study investigated seasonal variations and diversity of litter-dwelling adult beetle assemblages. It is the first study to sample beetles using a sifting method in all seasons, including winter, in the temperate forests of Korea. For this study, we predicted that (1) adult beetle species richness and abundance would be highest in summer and lowest in winter because many adults may not overwinter in leaf litter (Leather et al. Reference Leather, Walters and Bale1995) and (2) the adult beetle assemblage composition would differ among seasons because different species have developed various life-history strategies to deal with seasonality (Tauber and Tauber Reference Tauber and Tauber1976; Wolda Reference Wolda1988). The results will provide important baseline data related to seasonal variations in the biodiversity of litter-dwelling beetles.
Materials and methods
Study sites
This study was conducted in Bukpyeong-myeon, Jeongseon-gun, Gangwon-do, South Korea, which is located in the southeastern part of Gangwon-do and the centre of the Mt. Taebaek Range. The average annual temperature in Jeongseon-gun is about 11 °C. August is the hottest month, with an average temperature of about 30 °C, and January is the coldest month, with an average temperature of –9.6 °C. Annual rainfall averages about 1100 mm, most of which falls in the summer (Korea Meteorological Administration 2020).
We selected three sites (site 1: Joldeulu-gil (Jol), 384 m elevation, 37° 26' 53.8" N, 128° 38' 20.9" E; site 2: Najeon-ri (Na), 782 m elevation, 37° 26' 02.0" N, 128° 36' 57.0" E; and site 3: Sukam-ri (Suk), 450 m elevation, 37° 29' 43.0" N, 128° 35' 00.0" E; Fig. 1). All sample areas were mixed-wood forests containing five dominant tree species: Quercus mongolica Fischer ex. Ledebour (Fagaceae), Q. serrata Murray (Fagaceae), Pinus densiflora Siebold & Zuccarini (Pinaceae), P. koraiensis Siebold & Zuccarini (Pinaceae), and Betula platyphylla Sukaczev (Betulaceae) (Heo and Lee Reference Heo and Lee2015). At each site, we sampled soil and deciduous leaf litter near stream margins. The largest distance between sites was 6 km (between Jol and Suk), and the two closest sites were 2 km apart (Jol and Na).

Fig. 1. Study sites in Bukpyeong-myeon, Jeongseon-gun, Gangwon-do, South Korea: A, Joldeulu-gil (37° 26' 53.8" N, 128° 38' 20.9" E, 384 m elevation); B, Najeon-ri (37° 26' 02.0" N, 128° 36' 57.0" E, 782 m elevation); and C, Sukam-ri (37° 29' 43.0" N, 128° 35' 00.0" E, 450 m elevation).
Sampling methods
We sampled leaf litter once each season, March (spring), August (summer), October (autumn), and December (winter) 2019. Each seasonal collection was carried out for 1∼2 days. A total of 31 samples were collected: seven samples each in spring, summer, and fall, and 10 samples in winter. To sample the frozen ground in winter, we first sifted the upper leaf litter and then tried to dig up the upper part of frozen soil that enabled the soil sample to be sifted. Although we collected more litter samples in winter than in the other seasons, the total volume of the samples was similar for each season and each location.
To standardise sifting, 3–4 L of mixed litter samples from Pinus and Quercus species were collected during each sampling using a sifter, which consisted of a 25 × 25-cm-square, 7-cm-deep, 1 × 1-cm-mesh sieve that fed into a fabric bag (Fig. 2). Small fragments of leaves and soil that included arthropods dropped into the fabric bag when leaf litter was shaken in the sifter. The sieved samples were brought to the laboratory in the fabric bags and placed in Berlese funnels to collect arthropods.

Fig. 2. Sifter and fabric bag: A, top view of the 25 × 25-cm-square, 1 × 1-cm-mesh sieve and B, side view.
Berlese funnels take advantage of soil arthropods’ behaviour to avoid light and dry environments, which, in the case of the funnel method, causes both overwintering and nonoverwintering arthropods to move earthwards out of litter samples and into a collection chamber below. The Berlese funnels used in the present study were constructed of tin, which does not rust, and measured 42 cm wide, 57 cm long, and 36 cm high. A piece of 30 × 40-cm rectangular 0.1 × 0.1-mm-mesh gauze was attached to a 1 × 1-cm metal sieve and placed inside the funnel. The funnel’s top lid contained a 100-Watt incandescent bulb to emit light and heat. We operated the Berlese funnel for about a week for each litter sample. Arthropods dropped to the bottom of the funnel and were placed in a collecting jar with 70% ethanol (Fig. 3).
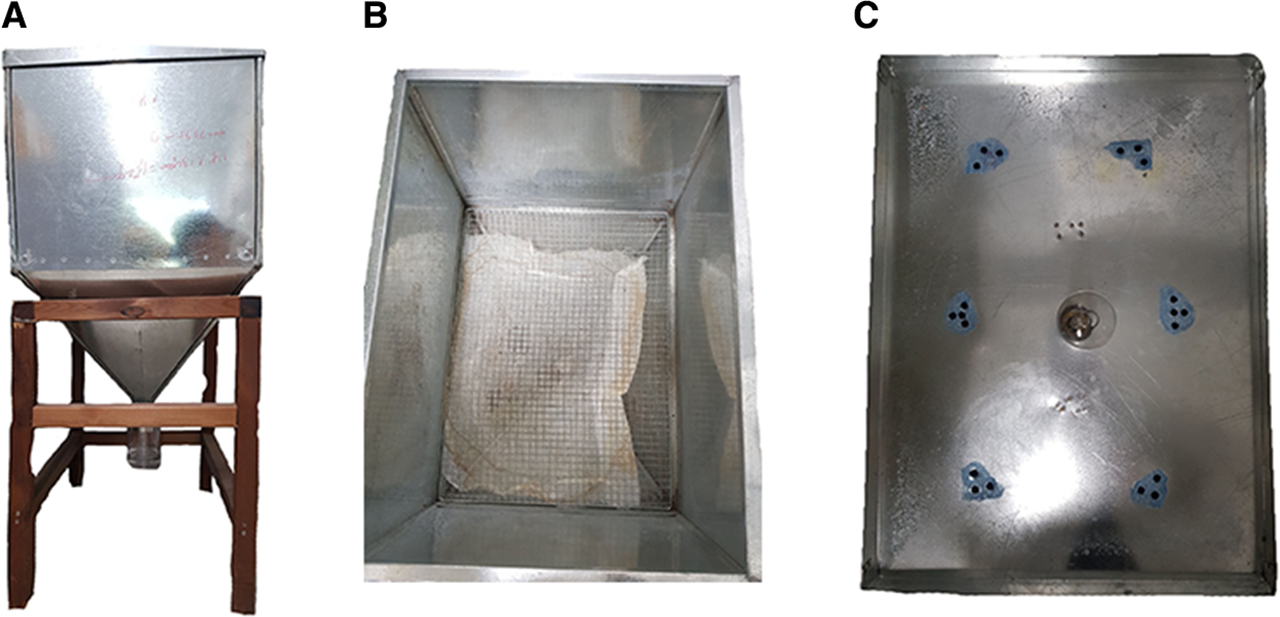
Fig. 3. Berlese funnel: A, external shape of the Berlese funnel attached to the wooden support frame; B, inside of the 42-cm-wide, 57-cm-long and 36-cm-high funnel; and C, the funnel’s inner lid connected to a 100-Watt light bulb.
Whenever possible, adult beetles were identified to species level. However, due to the lack of taxonomic information on litter-dwelling beetles in Korea, some species were identified only to the genus level or multiple species were combined as a single species group and treated as one species for analysis (e.g., Aleocharinae spp.). Adult beetles were identified using the keys in taxonomic literature (e.g., Hayashi et al. Reference Hayashi, Morimoto and Kimoto1984; Kurosawa et al. Reference Kurosawa, Hisamatsu and Sasaji1985; Ueno et al. Reference Ueno, Kurosawa and Sato1985; Kurbatov Reference Kurbatov1991; Cho and Ahn Reference Cho and Ahn1999, Reference Cho and Ahn2001; Arnett and Thomas Reference Arnett and Thomas2000; Kim and Ahn Reference Kim and Ahn2000a, Reference Kim and Ahn2000b; Park et al. Reference Park, Kwon, Park, Bae, Bae and Byun2012; Lee and Ahn Reference Lee and Ahn2015, Reference Lee and Ahn2019; Ahn et al. Reference Ahn, Cho, Kim, Yoo and Newton2017; Kim et al. Reference Kim, Min, Jeong, Ahn and Baek2017; Jeong and Ahn Reference Jeong and Ahn2018; Choi et al. Reference Choi, Park, Kim and Park2020; Hoshina and Park Reference Hoshina and Park2020). We used a Garmin GPSmap 60CSx (Garmin Ltd., Schaffhausen, Switzerland) to measure geographic position and altitude, a Tenmars TM-183 meter to measure temperature and humidity (Tenmars Electronics Co., Taipei, Taiwan), and a Bosch Professional GIS 500 thermo detector (Bosch, Gerlingen, Germany) to measure soil temperature at each sampling site. Most specimens used for the study were deposited in the Chungbuk National University Insect Collection (Cheongju, South Korea).
Statistical analyses
We used permutational multivariate analysis of variance to test differences in species composition among season × location combinations (Anderson et al. Reference Anderson, Gorley and Clarke2008). Bray–Curtis distances were calculated on square root transformed data, with 9999 permutations for the main test. We partitioned variation using the default type III sum of squares (Anderson et al. Reference Anderson, Gorley and Clarke2008). When the main tests were significant (P < 0.05), we performed 999 permutations for an a posteriori pairwise comparison. We used the PERMANOVA+ add-on package for Primer, version 7, for these analyses (Anderson et al. Reference Anderson, Gorley and Clarke2008; Clarke and Gorley Reference Clarke and Gorley2015).
We used redundancy analysis (1) to visually understand the structures of adult beetle assemblage among season × location combinations and (2) to determine the significant environmental variables underlying the variations in adult beetle assemblages among the above combinations. This method represents constrained ordination; thus, environmental variables directly influence the ordination process (Legendre and Legendre Reference Legendre and Legendre2012). The environmental variables measured for this study were altitude, atmospheric temperature, humidity, and soil surface temperature. We included these variables in the initial model including the main factors (i.e., season and location) and then selected the best-fit model to perform redundancy analysis with this final model. We used a Hellinger transformation on the species data to minimise the weight of rare species (Legendre and Gallagher Reference Legendre and Gallagher2001).
We used rarefaction and extrapolation to standardise uneven sampling efforts and to estimate species richness among seasons and locations. Rarefaction and extrapolation better characterise species richness than conventional individual-based rarefaction because it compares assemblages at a minimum coverage level in contrast with a minimum number of individuals (Chao et al. Reference Chao, Chiu and Jost2014a, Reference Chao, Gotelli, Hsieh, Sander, Ma, Colwell and Ellison2014b). By doubling the reference sample size, extrapolation better predicts the presence of rare species (Budka et al. Reference Budka, Łacka and Szoszkiewicz2019). We visualised estimated species richness using the “ggiNEXT” function in R (Hsieh et al. Reference Hsieh, Ma and Chao2016).
We used a generalised linear model, with residuals following a negative binomial distribution to analyse differences in abundance of adult beetles under different seasons and locations. We used Tukey’s honestly significant difference test for multiple comparisons of species richness and abundance when results from the generalised linear modelling were significant.
We used indicator species analysis to identify species associated with specific seasons and locations (Dufrêne and Legendre Reference Dufrêne and Legendre1997). Indicator species analysis is an analytical method used to evaluate the statistical significance of relationships between species occurrence or abundance and any defined group (De Cáceres et al. Reference De Cáceres, Legendre and Moretti2010). We generated the “IndVal” function using 4999 permutations and calculated P-values for each species to ensure the results were different from random (α = 0.05).
All analyses, except for permutational multivariate analysis of variance, were conducted using R, version 3.6.3 (R Core Team 2020), using the “Vegan” (Oksanen et al. Reference Oksanen, Blanchet, Friendly, Kindt, Legendre and McGlinn2019), “MASS” (Venables and Ripley Reference Venables and Ripley2002), “multcomp” (Hothorn et al. Reference Hothorn, Bretz and Westfall2008), “iNEXT” (Hsieh et al. Reference Hsieh, Ma and Chao2016), and “indicspecies” packages (De Cáceres and Legendre Reference De Cáceres and Legendre2009).
Results
General results
A total of 5820 arthropods, representing six classes, were collected over four seasons (Supplementary material, Table S1). These arthropods included 4125 insects representing 11 orders (Supplementary material, Table S2). Among these insects, 1422 adult beetles representing 24 families and a minimum of 141 species were collected, with 73 singletons and 18 doubletons (Table 1, Supplementary material, Table S3). The minute hooded beetle, Lewisium japonicum (Corylophidae), was the dominant species collected among the beetles, with 376 individuals, and accounted for 26% of the total beetle catch. Two aleocharine rove beetles (Staphylinidae), Atheta koreana and A. pasniki, were the second- and the third-most common species, with 80 and 70 individuals, respectively (Table 1).
Table 1. List of adult beetles with the number of individuals collected in four seasons in Jeongseon-gun, Gangwon-do, Korea, using a sifting method followed by Berlese funnel extraction.

Beetle assemblage composition
Results of the permutational multivariate analysis of variance showed that adult beetle–assemblage composition differed significantly in terms of season (pseudo-F = 2.35, P = 0.001) and location (pseudo-F = 1.50, P = 0.018), but the results showed no interaction between season and location (pseudo-F = 0.94, P = 0.676; Table 2). Pairwise comparisons showed that beetle assemblage composition differed among seasons, except between spring and winter. In terms of location, assemblage composition was similar between Na and Suk, whereas the assemblage composition in Jol differed significantly from that of Na and Suk (Table 2).
Table 2. Results of permutational multivariate analysis of variance testing for adult beetle species richness by season and location.

Significant differences (P < 0.05) are highlighted in bold.
Abbreviations: df, degrees of freedom; Lo, location; MS, mean squares; PERM, permutational multivariate analysis of variance; Se, season; SS, sum of squares.
According to the results of the redundancy analysis, the final model revealed significant differences across seasons (F = 2.09, P = 0.005) and locations (F = 1.35, P = 0.035). Overall assemblage structure was significantly correlated with soil surface temperature (F = 2.74, P = 0.005) and atmospheric temperature (F = 2.16, P = 0.005) along axis 1 (Table 3; Fig. 4). The model explained 31.6% of the total variance, with axes 1 and 2 accounting for 10.4% and 5.7% of the variance, respectively (Fig. 4). The permutational multivariate analysis of variance results were strongly supported by the redundancy analysis ordination, indicating that the assemblage composition differed among seasons except for a slight overlap during spring and winter. Also, the ellipse for summer assemblages was the largest, and the ellipse for winter assemblages was the smallest, suggesting that assemblages in summer and winter were the most heterogeneous and homogeneous, respectively (Fig. 4a). Two temperature variables seemed to drive seasonal differences of beetle assemblage structure along axis 1 (Fig. 4a). In terms of location, the redundancy analysis ordination showed that the assemblage composition was similar between Na and Suk (Fig. 4b). Furthermore, the ellipse for Jol assemblages was smallest, suggesting that the assemblages in Jol were more homogeneous than those in Na and Suk. Although it was not significant, altitude and humidity tended to drive regional variations of beetle assemblages along axis 2 (Fig. 4b).
Table 3. Results of redundancy analysis testing for adult beetle assemblages by factors.

Significant differences (P < 0.05) are noted in bold.
Abbreviations: df, degrees of freedom; AIC, Akaike information criteria; S.Temp, soil surface temperature; Temp, atmospheric temperature; Hum, humidity; Alt, altitude.
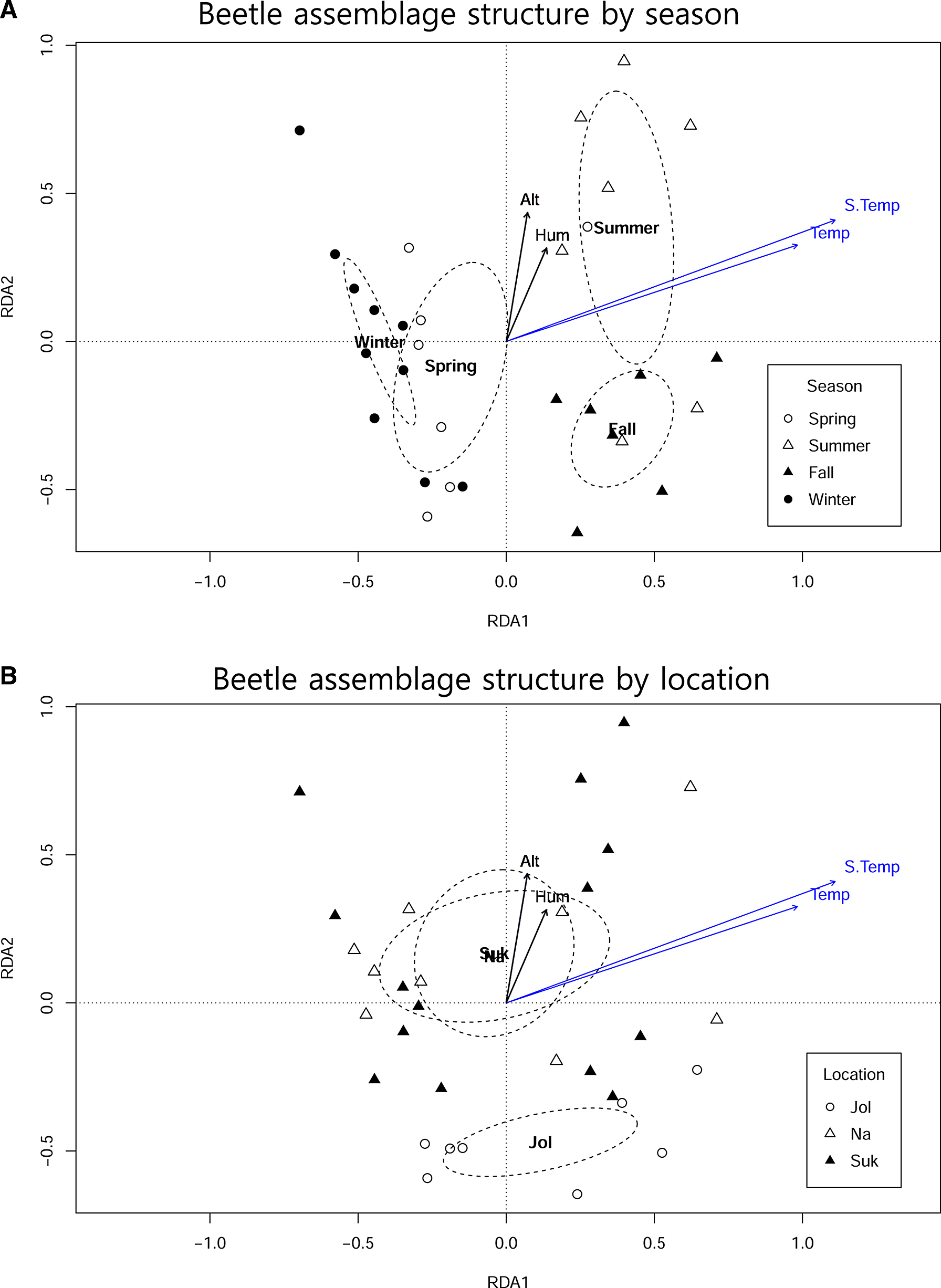
Fig. 4. Redundancy analysis (RDA) ordination of adult beetle assemblages by A, season and B, location. The model explained 31.6% of the total variance, and axis 1 and axis 2 explained 10.3% and 5.5% of the total variance, respectively. Arrows indicate environmental variables, with significant factors coloured blue. Abbreviations: Alt, altitude; Hum, humidity; S.Temp, soil surface temperature; Temp, atmospheric temperature.
Species richness and number of individuals
Estimated species richness of adult beetles was highest in spring and lowest in summer at 67.8% and 96.3% sample coverages, respectively, based on extrapolated curves (Fig. 5a). Estimated species richness was similar between fall and winter, both at 93.4% sample coverages, showing higher species richness than that in summer. However, species richness in fall and winter did not differ significantly from that in spring (Fig. 5a). Estimated species richness did not differ among locations, at 90.8%, 95.0%, and 92.8% sample coverages for Jol, Na, and Suk, respectively (Fig. 5b). However, Suk tended to be highest in species richness, whereas Jol tended to be lowest (Fig. 5b).

Fig. 5. Estimated species richness for A, season and B, location. Solid lines indicate interpolation, and dotted lines indicate extrapolation. Shaded areas indicate 95% confidence intervals.
Adult beetle abundance differed significantly among seasons (deviance = 50.7, P < 0.001), with the lowest abundance occurring in spring (Fig. 6a). However, beetle abundance did not differ significantly among locations (deviance = 37.8, P = 0.108; Fig. 6b).

Fig. 6. Adult beetle abundance for A, season and B, location. Error bars represent the standard errors (SE) for each location. Different lowercase letters above error bars show significant post hoc results. N.S., nonsignificant post hoc result (Tukey’s honestly significant difference test, P < 0.05).
Indicator species analysis
Nine and three species were identified as significant indicators of season and location, respectively. All indicators of season were members of Staphylinidae, except for the featherwing beetle, Ptinella sp.1 (Ptiliidae). In terms of season, Aleocharinae spp. showed the highest indicator value in fall (IndVal = 0.830), followed by Sepedophilus germanus (Sharp) in summer (IndVal = 0.782). Philonthus sp.1 was the only indicator in winter (IndVal = 0.579). No indicator species were found in spring (Table 4). Based on location, Perigona nigriceps Dejean showed the highest indicator value in Jol (IndVal = 0.612), followed by Cryptophagus sp.1 and Meligethes flavicollis Reitter, showing the same indicator value in Na (IndVal = 0.577; Table 4).
Table 4. Significant indicator species of adult beetles for season and location.

* Indicator value
P-values were calculated after 4999 permutations.
Discussion
Seasonal variations of adult beetle assemblage structure
The present study clearly showed that adult beetle assemblages differed among seasons, except between spring and winter, which partly overlapped. This similarity in assemblage structure between spring and winter is interesting. It is plausible that a large number of adult species overwintering under leaf litter emerged and were collected in spring, thereby displaying a similar assemblage structure between spring and winter (Burgess Reference Burgess1981; Bale and Hayward Reference Bale and Hayward2010). In addition, the dissimilarity of beetle assemblages between spring, summer, and fall emphasises that each species has a different developmental plan, thereby emerging as adults in a different season. The distinct assemblage structure among seasons suggests the importance of sampling in different seasons to understand the total diversity of litter-dwelling beetles. A transition from dry to rainy seasons in summer may have produced different adult beetle assemblage structures due to environmental changes (Anu et al. Reference Anu, Sabu and Vineesh2009). Our study also revealed that the summer beetle assemblage was the most heterogeneous. Because of the high temperature in summer, activity and dispersibility of insects increase (Mellanby Reference Mellanby1939; Taylor Reference Taylor1963), leading to structurally diverse summer communities. Winter assemblages were the most homogeneous, possibly due to low insect dispersibility and immobility or diapause (Bertram Reference Bertram1935; Mellanby Reference Mellanby1939; Bale and Hayward Reference Bale and Hayward2010).
Seasonal changes in species richness and abundance
Previous studies have shown the highest species richness and abundance of adult beetles occurred in either summer or fall in Korea (Byun et al. Reference Byun, Lee, Park, Kim and Jo2009a, Reference Byun, Lee, Park, Lee and Jo2009b, Reference Byun, Lim, Park, Kim, Lee and Jo2010; Park et al. Reference Park, Lim, Jo and Lee2011; Lim et al. Reference Lim, Lee, Park and Jo2011, Reference Lim, Park, Lee and Jo2012). Contrary to our prediction, the results of the present study showed that species richness was lowest in summer and that richness in winter was as high as that in spring and fall. In addition, abundance in winter was similar to values found for summer and fall, suggesting that winter sampling using a sifting method was highly effective for sampling adult beetles. Some species found in litter samples in winter may not be directly related to the soil and leaf-litter environment, as they use this habitat only for overwintering. The lowest abundance of adult beetles in spring may be attributed to dried leaf litter and soils that may cause beetles to move into more humid habitats, such as under logs or under bark (Janzen and Schoener Reference Janzen and Schoener1968; Pinheiro et al. Reference Pinheiro, Diniz, Coelho and Bandeira2002). Most biodiversity studies in temperate forests use sweeping, light traps, Malaise traps, window, or pitfall traps to sample arthropods (Gadagkar et al. Reference Gadagkar, Chandrashekara and Nair1990; Recher et al. Reference Recher, Majer and Ganesh1996; Hébert et al. Reference Hébert, Jobin, Fréchette, Pelletier, Coulombe, Germain and Auger2000; Kai and Corlett Reference Kai and Corlett2002; Work et al. Reference Work, Buddle, Korinus and Spence2002; Park and Cho Reference Park and Cho2007; Santos et al. Reference Santos, Cabanas and Pereira2007; Vasconcellos et al. Reference Vasconcellos, Andreazze, Almeida, Araujo, Oliveira and Oliveira2010; Park et al. Reference Park, Lim, Jo and Lee2011; Lim et al. Reference Lim, Park, Lee and Jo2012; Dadmal and Khadakkar Reference Dadmal and Khadakkar2014; Saji and Al Dhaheri Reference Saji and Al Dhaheri2014; Kim and Kwon Reference Kim and Kwon2016; Wardhaugh et al. Reference Wardhaugh, Stone and Stork2018). Because of this, it was difficult to directly compare the results for arthropod diversity and abundance of the present study with those of other studies due to the different sampling methods used by prior studies to collect biological data in the winter.
Only a few studies have used a combined sifting–Berlese funnel method to investigate insect biodiversity (Carlton and Robison Reference Carlton and Robison1998; Ferro et al. Reference Ferro, Gimmel, Harms and Carlton2012a, Reference Ferro, Gimmel, Harms and Carlton2012b). For example, Carlton and Robison (Reference Carlton and Robison1998) observed the highest abundance of litter-dwelling beetles in spring and fall and the lowest abundance in early summer and winter. Ferro et al. (Reference Ferro, Gimmel, Harms and Carlton2012b) reported that both species richness and abundance of adult beetles were higher in spring than in fall. Differences between the present study and other studies were: (1) the types of material used during sifting, as they also sifted rotten deadwood together with leaf litters and soils; (2) the operation time for the funnel extraction (one week in the present study versus two days in the earlier studies); and (3) environmental factors, such as temperature, humidity, and rainfall in each sampling region.
Effects of environmental factors on beetle assemblage
Temperature affects insect abundance and assemblages (Wagner et al. Reference Wagner, Wu, Feldman, Sharpe and Coulson1985; Gilbert and Raworth Reference Gilbert and Raworth1996; Régnière et al. Reference Régnière, Powell, Bentz and Nealis2012). In our study, assemblage structures of adult beetles were affected by both soil surface and atmospheric temperatures, supporting previous findings that litter-dwelling beetles were sensitive to both soil surface temperature (Robinson et al. Reference Robinson, McLaughlin, Marteinsdóttir and O’ Gorman2018) and atmospheric temperature (Ruggiero et al. Reference Ruggiero, Sackmann, Farji-Brener and Kun2009). Other factors, such as humidity and soil pH, showed no significant effects on variation in the assemblage structure.
Precipitation and humidity also significantly affect insect communities (Robinson and Robinson Reference Robinson and Robinson1970; Smythe Reference Smythe1973; Wolda Reference Wolda1978a, Reference Wolda1978b; Rees Reference Rees, Sutton, Whitmore and Chadwick1983; Frith and Frith Reference Frith and Frith1990; Pinheiro et al. Reference Pinheiro, Diniz, Coelho and Bandeira2002). However, in the present study, beetle assemblages were not affected by humidity. Characteristics of litter samples in our study areas were similar to each other, with wet soils and deciduous leaf litters. Soil pH in our study areas also did not affect beetle assemblage structure, supporting the results of Cameron and Leather (Reference Cameron and Leather2012), who suggested that soil pH was unrelated to insect and carabid abundance. In our study areas, soil pH ranged between 6.7 and 7.0, showing no significant difference among seasons or locations. Additional environmental factors, such as vegetation, soil composition, and presence of deadwood, are better predictors of the structure of litter-dwelling arthropods and their environmental relationships (Ulyshen and Hanula Reference Ulyshen and Hanula2009; Ashford et al. Reference Ashford, Foster, Turner, Sayer, Sutcliffe and Tanner2013).
Regional variations in adult beetle diversity and community structure
Both Na and Suk showed similar adult beetle assemblage structure despite these sites being the farthest apart. Beetle assemblages in Na and Suk were similarly heterogeneous, whereas those in Jol showed the maximum homogeneity. The lack of differences in annual average temperatures and soil surface temperatures at Na and Suk may help explain the similar beetle assemblage structures in these areas. Although we did not investigate plant species as an environmental variable, the different species composition of plants at each sampling area may have influenced the beetle assemblage composition (Andow Reference Andow1991; Koricheva et al. Reference Koricheva, Mulder, Schmid, Joshi and Huss-Danell2000; Scherber et al. Reference Scherber, Mwangi, Temperton, Roscher, Schumacher, Schmid and Weisser2006).
The Jol site had lower species richness and abundance of adult beetles compared to the other sites. Since the sample area at Jol was narrower compared to those at Na and Suk, the least richness and abundance at Jol were expected. Conversely, Na tended to show high species richness and abundance of beetles. The average elevation at Na (782 m) was higher than at Jol (384 m) and Suk (450 m), and this difference likely affected the diversity and assemblage structure. Previous studies have reported changes in diversity and assemblages along an elevational gradient (Lawton et al. Reference Lawton, MacGarvin and Heads1987; Wolda Reference Wolda1987; Olson Reference Olson1994; Sánchez-Reyes et al. Reference Sánchez-Reyes, Niño-Maldonado and Jones2014).
Advantages of sampling winter assemblages using a sifting method
Sampling litter-dwelling arthropods in winter is challenging because many of them enter diapause to survive the cold. Therefore, few collecting methods, such as sifting and pitfall traps, can be used to sample these overwintering arthropods. The sifting method has a great advantage in collecting higher species richness and individuals of insects than any other trapping method in winter because it enables collection of sedentary and overwintering invertebrates in diapause. In contrast, pitfall traps collect only arthropods that are winter active (Hamilton et al. Reference Hamilton, Wiedenmann, Skvarla, Sathyamurthy, Fisher, Fisher and Dowling2018). As shown by our results, a sifting method combined with a Berlese funnel extraction can be used to effectively collect diverse arthropod taxa in winter, during which the biodiversity of beetles in leaf litter is surprisingly high. Furthermore, a sifting–Berlese funnel method allows sampling of very small invertebrates (less than 1 mm) and yields relatively undamaged and rare specimens, thereby providing better opportunities to find new species in this less-explored environment.
Acknowledgements
The authors thank Yeon-Jae Choi, Min-Sang Jang, Jun-Young Kang, Ji-Won Kang, Su-Ho Choi, Tae-Young Jang, and Ji-Wook Kim, who helped collect arthropods in the field, and Dr. Boo-Hee Jung, who helped provide ecological information of Tenebrionidae. They also thank Jeffrey Battigelli and three anonymous reviewers who provided valuable suggestions to improve the manuscript. This study was supported by two grants from the National Institute of Biological Resources (NIBR), funded by the Ministry of Environment (MOE) of the Republic of Korea (NIBR202002112 and NIBR202130202).
Author contributions
U.-J.B. and S.-I.L. contributed equally to this work.
Conflicts of interest
The authors declare that they have no known competing financial interests or personal relationships that could have appeared to influence the work reported in this paper.
Supplementary material
To view supplementary material for this article, please visit https://doi.org/10.4039/tce.2021.54.