Dietary fibre is the part of plant foods or complex carbohydrates (e.g. cellulose, lignin) that is not digestible or absorbable by humans. Despite its minimal contribution to total energy, dietary fibre is believed to provide important health benefits including protection from colorectal cancer. Cumulative evidence, especially those from large prospective cohort studies including the European Prospective Investigation into Cancer and Nutrition (EPIC)(Reference Bingham, Day and Luben1,Reference Bingham, Norat and Moskal2) and the Prostate, Lung, Colorectal, and Ovarian Cancer Screening Trial (PLCO)(Reference Kunzmann, Coleman and Huang3), supports an inverse association between total dietary fibre intake and colorectal cancer risk(Reference Park, Hunter and Spiegelman4–Reference Aune, Chan and Lau6). A previous meta-analysis of sixteen prospective studies estimated a 10 % lower colorectal cancer risk associated with every 10 g/d intake of total dietary fibre(Reference Aune, Chan and Lau6). In the World Cancer Research Fund/American Institute for Cancer Research 2018 Report(7), the evidence for dietary fibre (defined as including both naturally occurring and added fibres) and colorectal cancer was considered ‘probable.’ Dietary fibre may influence colorectal cancer risk by increasing stool bulk, diluting faecal carcinogens in the colonic lumen, shortening transit time of faeces through the bowel(Reference Lupton and Turner8,Reference Cummings, Bingham and Heaton9) and binding secondary bile acids which may otherwise act as tumour promoters(Reference Harris and Ferguson10,Reference Kritchevsky11) . Fibre can also undergo bacterial fermentation to lower luminal pH(Reference Walker, Walker and Walker12) and produce SCFA with anticarcinogenic properties(Reference Kritchevsky11,Reference Sengupta, Muir and Gibson13–Reference Gamet, Daviaud and Denis-Pouxviel15) . Moreover, recent studies suggest that dietary fibre increases microbiota diversity in the gut(Reference Segata16) and that gut microbiota are associated with colorectal cancer risk(Reference Feng, Liang and Jia17–Reference Wang, Cai and Qiu19). The protective effects of fibre may act upon different stages of carcinogenesis: the initial formation of adenomas (precancerous lesions), the growth of adenomas and the development of cancer. Although little is known about the effect of fibre on early stages of transformation to malignancy, some studies have reported a lower incidence of adenoma associated with highest (v. lowest) levels of total dietary fibre intake(Reference Martinez, McPherson and Annegers20–Reference Peters, Sinha and Chatterjee23). In a previous meta-analysis(Reference Ben, Sun and Chai24), a 34 % lower adenoma risk was associated with total dietary fibre intake in the analysis of case-control studies but no association was found in the analysis of cohort studies.
Major food sources of dietary fibre are cereals/grains, vegetables, fruits and legumes. Dietary fibres from different food sources are heterogeneous in respect to chemical composition, physicochemical properties and solubility and thus are hypothesised to present varying degrees of anticarcinogenic properties. However, the evidence on the relationships of dietary fibre intake from different food sources with colorectal cancer and adenoma risks is mixed. Further, little is known about the shape of the relationships (e.g. linear, U-shape) as well as the relative importance of fibre source. A previous meta-analysis that evaluated fibre intakes from different food sources (cereals, vegetables, fruits and legumes) reported a statistically significant inverse association with cereal fibre only(Reference Aune, Chan and Lau6). It is unknown whether the variation in significant associations observed by fibre source was due to differences in statistical power (e.g. number of studies included) and participant characteristics among studies included, or due to putative biological differences between fibres from different food sources. In the present study, we conducted a meta-analysis by restricting analyses to publications that reported all fibre sources (cereal/grain, vegetable, fruit, legume) to increase the comparability between the results. We also included additional studies that were not part of the previous meta-analysis. Further, we performed linear and non-linear dose–response meta-analyses to explore the shapes of the relationships and to quantify the risk associated with specific levels of fibre intake.
Methods
The Meta-analysis Of Observational Studies in Epidemiology (MOOSE) checklist(Reference Stroup, Berlin and Morton25) was closely followed for the design, analysis and reporting of this meta-analysis. H. K. conducted literature search, study selection and data extraction, and D. H. L. checked for accuracy.
Search strategy and study selection
Studies published up to August 2018 were identified by searching PubMed and Embase databases. The detailed search terms used for PubMed and Embase are provided in online Supplementary Table S1. To be included in our meta-analysis, studies had to be prospective cohorts. Language was limited to English. When multiple publications were from the same study, we used the publication that was most recently published. For dose–response meta-analysis, studies had to provide a quantitative measure of fibre intake for at least three categories with relative risk (RR) estimates (hazard ratios, OR or risk ratios) and 95 % CI, category-specific or total number of cases, and category-specific or total number of non-cases or person-years.
We initially searched for studies on fibre intake and outcomes including colorectal adenoma, colorectal cancer and colorectal cancer survival or mortality. However, because there were not enough studies that reported association of different fibre sources and cancer survival or mortality, we only included studies on adenoma and cancer outcomes. For colorectal cancer studies, we included publications that reported all four fibre sources (cereal/grain fibre, vegetable fibre, fruit fibre and legume fibre). However, for adenoma studies, we included publications that reported three fibre sources (cereal/grain fibre, vegetable fibre and fruit fibre), because there were only few studies that reported all four. There was a total of ten publications (six for colorectal cancer, five for adenoma) eligible for this dose–response meta-analysis. Study selection procedure is summarised in Fig. 1.

Fig. 1. Flow chart of study selection. RR, relative risk.
Data extraction
For each study, we extracted the following data: first author’s last name, publication year, study design, study population, sex, average follow-up period, quantity of intake, number of cases, number of non-cases, most fully adjusted RR and corresponding 95 % CI, and adjustment variables. The extracted data are shown in Tables 1 (for colorectal cancer incidence) and 2 (for colorectal adenoma incidence).
Table 1. Prospective studies of fibre intake and colorectal cancer incidence

RR, relative risk; NIH-AARP, National Institutes of Health-American Association of Retired Persons.
* Note: 4184 kJ = 1000 kcal.
Table 2. Prospective studies of fibre intake and colorectal adenoma incidence

RR, relative risk.
* Note: 4184 kJ = 1000 kcal.
Statistical analysis
Linear and non-linear dose–response meta-analyses were conducted for colorectal cancer, incident adenoma and recurrent adenoma risk. For linear dose–response meta-analyses, we used the methods described by Greenland and Longnecker(Reference Greenland and Longnecker26) to compute study-specific slopes (linear trends) and 95 % CI from the natural logs of the RR and CI extracted across categories of dietary fibre intake. In estimating study-specific linear trends, several approximations were made: the midpoint of dietary fibre intake in each category was assigned to the corresponding RR; the width of the open-ended extreme categories was assumed to be same as that of the adjacent interval; when quantile-based studies did not provide the distributions of cases and person-years (or non-cases), total cases and person-years were equally divided across the quantiles. Then, the estimated study-specific RR and variances were pooled using the DerSimonian-Laird random-effects models(Reference DerSimonian and Laird27) to estimate the summary RR and 95 % CI. For each dietary fibre source, forest plots of the linear dose–response meta-analysis were presented for RR and 95 % CI associated with each 10 g/d increment in dietary fibre intake.
To examine the potential non-linear relationships of individual fibre sources with colorectal cancer risk, non-linear dose–response meta-analysis was conducted using restricted cubic spline approach(Reference Harrell, Lee and Pollock28,Reference Orsini and Greenland29) . For each study, cubic splines were modelled with three knots fixed at 10th, 50th and 90th percentiles of the distribution of fibre intake, accounting for correlation across category-specific RR and 95 % CI within each study(Reference Harrell, Lee and Pollock28). The reference was set to the lowest value of the reported fibre intake. Then, the derived curves were combined using multivariate random-effects meta-analysis(Reference White30). The P value for non-linearity was obtained from the test of the null hypothesis that the regression coefficient of the second spline transformation was equal to zero. We performed non-linear dose–response meta-analyses when there were at least five studies. For this reason, we did not perform non-linear dose–response meta-analysis for incident and recurrent adenomas because there were few studies.
Heterogeneity in the relationship across studies was assessed by Cochran’s Q test(Reference Higgins and Thompson31) and quantified by I 2, the percentage of total variation across studies that is attributable to true heterogeneity rather than to chance(Reference Higgins, Thompson and Deeks32). To identify sources of heterogeneity and assess study quality, subgroup analyses and meta-regression were conducted based on linear dose–response meta-analysis by a priori selected variables related to etiologic heterogeneity, potential effect modifiers and methodological characteristics. Potential for small study effects(Reference Nuesch, Trelle and Reichenbach33,Reference Sterne, Gavaghan and Egger34) , such as publication bias, was tested using Egger’s test(Reference Egger, Davey Smith and Schneider35). Diverse sensitivity analyses including the influence analysis were performed to check robustness of the results.
For statistical significance, two-sided significance level was set at α = 0·05. All statistical analyses were conducted using STATA 12 (StataCorp).
Results
After screening 4632 publications, ten prospective studies (six for colorectal cancer(Reference Bingham, Norat and Moskal2,Reference Kunzmann, Coleman and Huang3,Reference Nomura, Hankin and Henderson5,Reference Schatzkin, Mouw and Park36–Reference Mai, Flood and Peters38) , four for incident adenoma(Reference Kunzmann, Coleman and Huang3,Reference Tantamango, Knutsen and Beeson21,Reference Fuchs, Giovannucci and Colditz39,Reference Platz, Giovannucci and Rimm40) , two for recurrent adenoma(Reference Kunzmann, Coleman and Huang3,Reference Jacobs, Giuliano and Roe41) ) were identified and included in this dose–response meta-analysis. Of these, nine studies were from the United States(Reference Kunzmann, Coleman and Huang3,Reference Nomura, Hankin and Henderson5,Reference Tantamango, Knutsen and Beeson21,Reference Schatzkin, Mouw and Park36–Reference Jacobs, Giuliano and Roe41) and one study was from Europe(Reference Bingham, Norat and Moskal2).
Colorectal cancer
A total of 8248 colorectal cancer cases from 1 340 841 participants were included in the meta-analyses of four different dietary fibre sources (cereal/grain, vegetable, fruit, legume fibres) in relation to colorectal cancer risk.
Cereal/grain fibre. For cereal/grain fibre, each 10 g/d increase in intake was associated with a 9 % decreased risk of colorectal cancer (RR 0·91, 95 % CI 0·82, 1·00) with no evidence of heterogeneity (I 2 = 0 %, P = 0·50) (Fig. 2(a)). Small study effects, such as publication bias, were not indicated by Egger’s test (P = 0·79). In the non-linear dose–response meta-analysis, the dose–response curve suggested no evidence of non-linearity (P non-linearity = 0·34) (Fig. 2(b)).
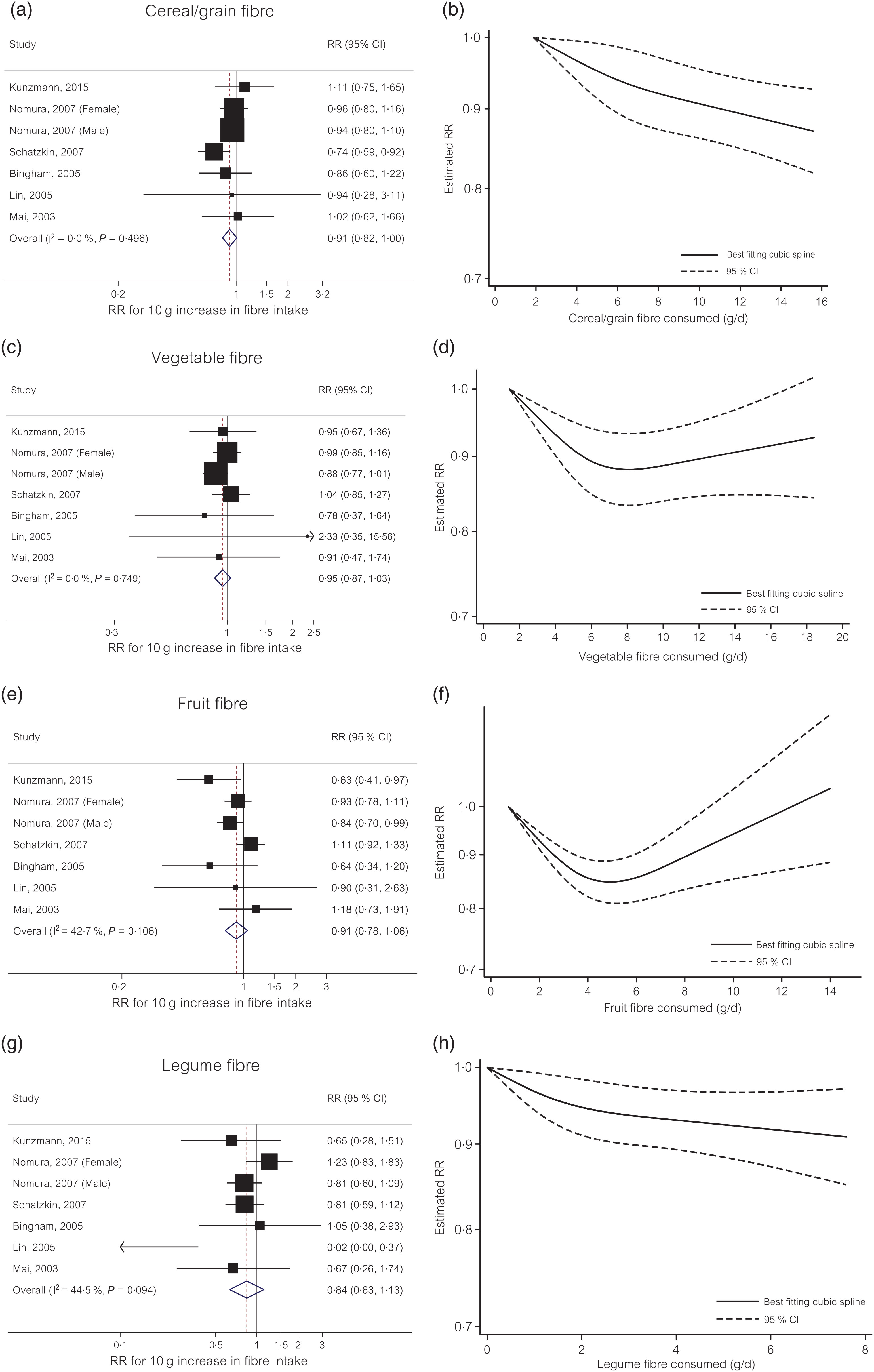
Fig. 2. Linear and non-linear dose–response meta-analyses of four different dietary fibre sources and colorectal cancer risk. (a) Linear dose–response for cereal/grain fibre; (b) non-linear dose–response for cereal/grain fibre; (c) linear dose–response for vegetable fibre; (d) non-linear dose–response for vegetable fibre; (e) linear dose–response for fruit fibre; (f) non-linear dose–response for fruit fibre; (g) linear dose–response for legume fibre; (h) non-linear dose–response for legume fibre. RR, relative risk.
Vegetable fibre. The summary RR for each 10 g/d increase in dietary intake of vegetable fibre was 0·95 (95 % CI 0·87, 1·03) with no evidence of heterogeneity (I 2 = 0 %, P = 0·75) (Fig. 2(c)). Small study effects, such as publication bias, were not evident with Egger’s test (P = 0·59). In the non-linear dose–response meta-analysis, there was evidence of non-linearity (P non-linearity = 0·001) with no further reduction in risk (e.g. plateau effect) beyond 7 g/d intake of vegetable fibre (Fig. 2(d)).
Fruit fibre. The summary RR for each 10 g/d increase in dietary intake of fruit fibre was 0·91 (95 % CI 0·78, 1·06) with moderate to high heterogeneity (I 2 = 43 %, P = 0·11) (Fig. 2(e)). Small study effects, such as publication bias, were not evident with Egger’s test (P = 0·55). In the non-linear dose–response meta-analysis, there was evidence of non-linearity for the relationship between vegetable fibre intake and colorectal cancer risk (P non-linearity<0·001) with no further evidence for risk reduction beyond 5 g/d intake (Fig. 2(f)).
Legume fibre. The summary RR of colorectal cancer for each 10 g/d increase in dietary intake of legume fibre was 0·84 (95 % CI 0·63, 1·13) with moderate to high heterogeneity (I 2 = 45 %, P = 0·09) (Fig. 2(g)). Small study effects, such as publication bias, were not evident with Egger’s test (P = 0·22). In the non-linear dose–response meta-analysis, the dose–response curve suggested no evidence of non-linearity (P non-linearity = 0·12) (Fig. 2(h)).
Subgroup and sensitivity analyses. To evaluate the role of confounding by folate intake, we conducted a subgroup analysis by folate adjustment. For cereal/grain fibre intake, the inverse association was observed in the folate-adjusted stratum only (summary RR 0·89, 95 % CI 0·80, 0·99) (online Supplementary Fig. S1). For legume and fruit fibres intake, the summary RR was slightly attenuated in the folate-adjusted stratum compared with folate-unadjusted stratum, whereas for vegetable fibre the association was similar in both strata. Because dietary fibres with different solubility and chemical properties may influence cancer risk at different location, we examined the associations between dietary fibre and colorectal cancer risk by location of cancer. The marginally significant inverse associations were observed with distal (summary RR 0·80, 95 % CI 0·62, 1·03) and rectal (summary RR 0·85, 95 % CI 0·71, 1·01) cancers but no association was found with proximal cancer (RR 1·00, 95 % CI 0·90, 1·12) (online Supplementary Fig. S2). Results did not change materially in sensitivity analyses including a large pooling project and simultaneously excluding two individual studies that contributed to the pooling project (online Supplementary Fig. S3).
Colorectal adenoma
A total of 3147 of incident adenoma (adenoma diagnosed during the follow-up among individuals with no personal history of adenoma at baseline) and 1376 recurrent adenoma (adenoma diagnosed during the follow-up among individuals who had a prior diagnosis of adenoma) cases from 66 747 participants were included in the meta-analyses of three different dietary fibre sources (cereal/grain, vegetable, fruit fibres) in relation to colorectal adenoma risk.
Cereal/grain fibre. The summary RR for each 10 g/d increase in dietary intake of cereal/grain fibre was 0·81 (95 % CI 0·54, 1·21) for incident colorectal adenoma and 1·03 (95 % CI 0·62, 1·71) for recurrent adenomas with moderate to high heterogeneity (I 2 = 65 %, P = 0·03; I 2 = 56 %, P = 0·13; respectively) (Fig. 3(a)). Small study effects, such as publication bias, were not evident with Egger’s test (P = 0·83 incident).

Fig. 3. Linear dose–response meta-analyses of three different dietary fibre sources and colorectal adenoma risk by incident and recurrent adenomas: (a) cereal/grain fibre; (b) vegetable fibre; (c) fruit fibre.
Vegetable fibre. Each 10 g/d increase in dietary intake of vegetable fibre was statistically significantly associated with a 16 % reduced risk of incident colorectal adenoma (OR 0·84, 95 % CI 0·71, 0·98) with no evidence of heterogeneity (I 2 = 0 %, P = 0·46) (Fig. 3(b)). For recurrent adenomas, the summary RR for each 10 g/d increase in dietary intake of vegetable fibre was 1·37 (95 % CI 0·79, 2·37) with moderate to high heterogeneity (I 2 = 54 %, P = 0·14). Small study effects, such as publication bias, were not evident with Egger’s test (P = 0·36 incident).
Fruit fibre. Each 10 g/d increase in dietary intake of fruit fibre was statistically significantly associated with a 22 % reduced risk of incident colorectal adenoma (OR 0·78, 95 % CI 0·65, 0·93) with no evidence of heterogeneity (I 2 = 0 %, P = 0·73) (Fig. 3(c)). For recurrent adenomas, the summary RR for each 10 g/d increase in dietary intake of fruit fibre was 0·95 (95 % CI 0·71, 1·27) with no evidence of heterogeneity (I 2 = 0 %, P = 0·91). Small study effects, such as publication bias, were not evident with Egger’s test (P = 0·49 incident).
Subgroup and sensitivity analyses. In subgroup analysis by location of cancers, the associations were generally similar between distal and rectal cancers (online Supplementary Fig. S4). Sensitivity analyses excluding one study at a time did not change the results materially (data not shown)
Discussion
Our dose–response meta-analysis of ten prospective cohort studies supports linear inverse associations between cereal/grain fibre and colorectal cancer risk and between fruit and vegetable fibres and incident colorectal adenoma risk. For vegetable and fruit fibres, our data suggested possible non-linear relationships (e.g. plateau effect) with colorectal cancer risk showing no further reduction in risk beyond 7 g/d intake of vegetable fibre and 5 g/d intake of fruit fibre.
Our findings are consistent with those from a previous meta-analysis which included eight prospective studies of cereal fibre and reported a 10 % reduced colorectal cancer risk associated with every 10 g/d increase in cereal fibre intake(Reference Aune, Chan and Lau6). Although this previous analysis included three studies that do not overlap with our analysis, the magnitude of summary estimate was almost identical with ours. Similarly, a pooled analysis of thirteen prospective cohort studies(Reference Park, Hunter and Spiegelman4) reported a suggestive inverse association of cereal fibre and whole grains with rectal cancer but no association with other fibre sources. The lack of evident association found with individual fibre sources other than cereal/grain fibres suggests that the inverse association of total dietary fibre with colorectal cancer risk shown in multiple observational studies(Reference Bingham, Norat and Moskal2–Reference Nomura, Hankin and Henderson5) may have been driven by the protective effects of cereal/grain fibre. However, it is also possible that the associations with individual fibre sources may have been masked in the analyses assuming linearity if the true underlying associations are non-linear (e.g. U-shape). In the present study, we performed a non-linear dose–response meta-analysis and observed suggestive non-linear relationships of fruit and vegetable fibres with colorectal cancer risk.
Dietary fibres are heterogeneous in respect to chemical composition, physicochemical properties, and solubility. For example, fibres may present different chemical compositions and physicochemical properties based on the degree of polymerisation(Reference Holscher42), and thus the effects of different fibre sources on colorectal cancer risk may vary depending on these properties. Certain fibre sources (e.g. cereal fibres) that are more often consumed together with starch may also contribute to glycaemic load reduction at a greater extent than other fibre sources (e.g. vegetable fibre) that are separately consumed. In addition, the location of microbial fermentation in the gastrointestinal tract is affected by the solubility of dietary fibre(Reference Holscher42). Pectin, which is naturally found in fruits, is readily metabolised by bacteria in the proximal gastrointestinal tract, whereas cellulose, which is present in grains and vegetables, is less soluble and can be fermented in the distal gastrointestinal tract(Reference Koropatkin, Cameron and Martens43). Thus, dietary fibres originated from different sources such as grains, vegetables and fruits may differentially impact microbial community diversity. In the present meta-analysis, we stratified by the tumour site (proximal, distal, rectal) as the etiology may vary by the location, and we found that the inverse association of total dietary fibre was restricted to distal and rectal cancers. This finding further supports the relative importance of cereal/grain fibre (high in insoluble fibre) over other fibre sources as these fibres are more likely to influence distal and rectal cancers. Furthermore, cereal/grain fibres, which are high in insoluble fibres, may bind carcinogens and reduce transit time of faeces through the bowel to a greater extent. However, future studies are needed to confirm the heterogeneous effects of fibres by solubility.
Colorectal adenoma is a precursor lesion of colorectal cancer and thus risk factors associated with adenomas are likely to influence the early stages in the process of malignant transformation. Randomised clinical trials of fibre supplementation (wheat-bran cereal) have reported no association with recurrent adenoma(Reference Alberts, Martinez and Roe44,Reference MacLennan, Macrae and Bain45) ; however, the evidence does not exclude the possible protective effect of fibre intake from other specific dietary source and the effect on incident adenoma. In a dietary intervention trial(Reference Lanza, Hartman and Albert46), increased fibre intake from dry beans was associated with a reduced risk of advanced adenoma recurrence and the protective effect was observed at a level much higher than the usual consumption levels reported in most observational studies. The possible threshold effect of legume fibre may partly explain the lack of association we observed in our meta-analysis of legume fibre and colorectal cancer risk as the ranges of intake levels were much lower in the studies included. Only few studies exist for legume fibre and adenoma partly due to very low levels of legume fibre intake in most populations(Reference Bingham, Norat and Moskal2,Reference Kunzmann, Coleman and Huang3,Reference Schatzkin, Mouw and Park36–Reference Mai, Flood and Peters38) . In the present meta-analysis, fruit and vegetable fibres were significantly associated with a reduced risk of incident colorectal adenoma, although it was only weakly associated with colorectal cancer risk. All fibre sources were not associated with the risk of recurrent colorectal adenoma. Fibre sources with different properties may influence different stages in the adenoma–carcinoma sequence. Our data suggest that fruit and vegetable fibres are likely to act upon the early stages in the process and cereal/grain fibre is likely to influence later stages in the process of malignant transformation. As observed in our analysis, factors that are strongly associated with adenoma are not necessarily as strongly associated with carcinoma, as most adenomas do not progress to invasive carcinoma. Future studies with longer time lag analysis are needed to clarify the effects of fibre sources on cancer v. adenoma.
Our study has several limitations. First, we cannot exclude the possibility of residual confounding by other dietary or lifestyle factors, such as folate and red meat intake, if the individual studies included in our meta-analysis did not inadequately adjust for them. However, in our subgroup analysis, the inverse association between cereal/grain fibre and colorectal cancer risk remained statistically significant in the folate-adjusted stratum. Second, publication bias can be a problem in a meta-analysis; however, all of our analyses indicated no evidence of publication bias based on Egger’s test. Third, dietary assessment of nutrients is a challenging task and thus measurement errors in the intake of dietary fibre may have influenced our results. All studies included in our meta-analysis used FFQ to assess dietary intake of fibre. In previous studies that corrected for measurement errors(Reference Bingham, Day and Luben1,Reference Park, Hunter and Spiegelman4) , the associations between fibre intake and colorectal cancer risk became stronger. These results suggest that the measurement errors in our meta-analysis are also likely to underestimate the associations. The definition and calculation of dietary fibre may also differ between studies contributing the heterogeneity in results. However, we found no evidence of high heterogeneity in our results. Dietary fibre intake in our study mainly refers to naturally occurring fibre because the original studies included in this meta-analysis did not assess the intake of added fibre (e.g. Metamucil). As the updated report published by the World Cancer Research Fund/American Institute for Cancer Research in 2018(7) defined dietary fibre as including both naturally occurring and added fibres, future studies are needed to examine the effects of added fibre on colorectal cancer and adenoma risks. Lastly, because individual fibre sources are correlated with each other, mutual adjustment for each other in regression models could have allowed us to examine the independent effects of individual fibre sources but none of the studies included has examined the independent effect.
Despite the limitations, the present study has important strengths. To the best of our knowledge, the present study is the first dose–response meta-analysis of prospective studies that examined both linear and non-linear relationships of dietary fibre intake from individual food sources in relation to colorectal cancer risk. A previous meta-analysis of dietary fibre(Reference Aune, Chan and Lau6) did not evaluate non-linear dose–response relationships separately for each individual fibre source, because their main aim was to examine the relationship of total fibre. In contrast, the main aims of our study were to examine the relative importance of fibre sources and evaluate the shape of the relationships to provide evidence for the optimal ranges of fibre intake. In order to achieve our aims, we carefully designed the criteria for study inclusion and performed various subgroup analyses (e.g. distal v. rectal, with v. without folate adjustment). By including prospective cohort studies only, we reduced recall bias and selection bias that are potential limitations in retrospective studies. In a previous meta-analysis of colorectal adenoma(Reference Ben, Sun and Chai24), there was discrepancy in results by study design possibly due to these biases in case-control studies. Further, by restricting analysis to studies that reported all fibre sources, we increased comparability between results from different fibre sources.
In summary, our dose–response meta-analysis of ten prospective cohorts supports potential protective effects of cereal/grain fibre against colorectal cancer and fruit and vegetable fibres against incident colorectal adenoma. The United States Department of Agriculture Dietary Reference Intakes recommends adults to consume 14 g of dietary fibre per 1000 kcal (4184 kJ) intake, which is equivalent to a daily intake of approximately 25 g for women and 38 g for men. Our data suggest that, although all fibre sources may provide some benefits, the evidence for colorectal cancer prevention is strongest for fibre from cereals/grains. However, further studies are needed to confirm the independent effect of each individual fibre source in relation to cancer risk.
Supplementary material
For supplementary material/s referred to in this article, please visit https://doi.org/10.1017/S0007114519001454
Acknowledgements
H. O. is supported by a Korea University Grant K1808781; N. K. was supported by funding from the National Research Foundation of Korea (NRF-2018R1C1B6008822; NRF-2018R1A4A1022589) and the Dongguk University Research Fund of 2017.
H. O. and H. K. collected, analysed and interpreted the data, and drafted the manuscript. D. L. and A. L. collected and interpreted the data and drafted the manuscript. E. G. and S. K. interpreted the data and drafted the manuscript. N. K. analysed and interpreted the data and drafted the manuscript.
None of the authors has any conflicts of interest to declare.