The incidence of non-alcoholic fatty liver disease (NAFLD) has increased with a rise in the obesity epidemic, with an estimated prevalence of 24 % worldwide( Reference Younossi, Anstee and Marietti 1 ). In 2016, the annual direct medical cost of NAFLD is estimated to be $103 billion for the USA, while the total estimated cost for Germany, France, Italy and the UK is €35 billion( Reference Younossi, Blissett and Blissett 2 ). NAFLD is a spectrum disease that encompasses simple steatosis, steatohepatitis, fibrosis and ultimately cirrhosis( Reference Hardy, Oakley and Anstee 3 ). The ‘two-hit model’ for NAFLD development and progression includes simple steatosis with fat accumulation in the liver, that is, the first hit, followed by the second hit for the progression of steatosis to steatohepatitis( Reference Paschos and Paletas 4 ). The second hits include oxidative stress, lipid peroxidation, pro-inflammatory cytokines, adipokines and mitochondrial dysfunction( Reference Paschos and Paletas 4 ). Insulin resistance also plays a prominent role in the progression of NAFLD, as 70–80 % of obese and diabetic patients have NAFLD( Reference Marchesini, Brizi and Morselli-Labate 5 , Reference Loomba, Abraham and Unalp 6 ).
When liver injury occurs, macrophages secrete cytokines such as transforming growth factor β1 (TGF-β1) and TNF-α ( Reference Friedman 7 , Reference Parola and Robino 8 ), which can activate hepatic stellate cells (HSC) to produce extracellular matrix (ECM) as part of the wound healing process( Reference Pellicoro, Ramachandran and Iredale 9 ). In contrast, in NAFLD, chronic inflammation leads to a dysregulated wound healing response, resulting in excessive ECM accumulation in the liver, that is, liver fibrosis( Reference Koyama and Brenner 10 ). As such, chronic inflammation drives fibrogenesis in HSC to exacerbate the progression of non-alcoholic steatohepatitis (NASH) to liver fibrosis.
The spleen is a secondary lymphoid organ that is closely associated with the liver via the portal vein system, such that splenic secretion passes through the liver before the general circulation( Reference Li, Duan and Chen 11 ). The precise contribution of the spleen to liver inflammation and fibrosis is not entirely clear. However, in humans, it has been demonstrated that spleen volume is increased in liver fibrosis and that the liver volume and spleen volume ratio, as measured by multi-detector computed tomography, is a good indicator of fibrosis grade( Reference Liu, Li and He 12 – Reference Jeong 14 ). In rodents, it has been demonstrated that the spleen is a source of inflammatory cells that migrate to the liver upon liver injury( Reference Mandal, Gardner and Sun 15 ). Furthermore, splenectomy in rodents has been shown to attenuate liver fibrosis, at least in part, through the attenuation of inflammatory macrophage infiltration( Reference Yada, Iimuro and Uyama 16 ). Therefore, the spleen plays an important role in liver inflammation and fibrosis, and bioactive foods that reduce splenic inflammation may attenuate the development of liver fibrosis.
To date, no single therapy has been approved for treating NAFLD, and few treatments for NASH exist( Reference Younossi 17 ). Given the chronic nature of NAFLD development, lifestyle changes and dietary means appear to be important in its prevention. Spirulina platensis (SP) is a multicellular and filamentous edible blue-green alga that is found naturally in alkaline lakes. It has also been cultured in a controlled environment for human consumption and is considered a ‘safe food with no side effects’ by the United Nations Industrial Development Organization programme( Reference Samuels, Mani and Iyer 18 ). The protective effects of SP against inflammatory diseases, such as colitis, arthritis and allergic rhinitis, have been documented in several animal and human studies( Reference Mao, Van de Water and Gershwin 19 – Reference Rasool, Sabina and Lavanya 21 ), although the underlying molecular mechanisms have not been elucidated. We previously reported that IL-6 secretion from splenocytes isolated from apoE knockout mice, a model of atherosclerosis, fed SP decreased upon lipopolysaccharide (LPS) challenge and that SP supplementation reduced atherosclerotic lesion formation( Reference Ku, Pham and Park 22 , Reference Ku, Kim and Pham 23 ). Furthermore, we also found that the organic extract of Spirulina platensis (SPE) represses the expression and secretion of pro-inflammatory cytokines with concomitant increases in histone H3 acetylation in macrophages( Reference Ku, Pham and Park 22 ). Based on the findings, we sought to investigate whether SP supplementation can inhibit the development of liver fibrosis through its effect on inflammation and whole-body energy metabolism in a diet-induced mouse model of liver fibrosis.
Methods
Animal care and diets
Male C57BL/6J mice at the age of 8 weeks were purchased from Jackson Laboratories and they were housed under a 12 h light–12 h dark cycle. Mice were randomly assigned to a low-fat (LF) control (6 % fat by weight, n 15), a high-fat (HF)/high-sucrose/high-cholesterol control (34 %/38 %/2·0 % by weight, n 15) or an HF diet supplemented with SP (HF/SP; 2·5 % by weight, n 15). A 2·5 % SP diet for mouse is equivalent to about 10 g of SP/d for humans. Diet composition is listed in Table 1. SP powder (Earthrise® Natural Spirulina) was generously provided by Earthrise Nutritionals. Mice had ad libitum access to water and food throughout the 20 weeks of the feeding period. Body weight and food consumption were recorded weekly, while plasma was collected every 4 weeks through the lateral tail vein after a 6 h fast. At the end of the feeding period, mice were fasted for 6 h and anaesthetised with ketamine (110 mg/kg)/xylazine (10 mg/kg) (Henry Schein Animal Health). Serum was collected by cardiac puncture after which the mice were euthanised by cervical dislocation before collection of liver and epididymal white adipose tissue (eWAT). All tissues were snap frozen in liquid N2 or fixed in 10 % formalin. Serum and tissues were stored at –80°C until analysis. All animal procedures were approved by the Institutional Animal Care and Use Committee at the University of Connecticut.
Table 1 Diet composition (g/kg) of a low-fat (LF) control diet or a high-fat (HF) diet containing Spirulina platensis (SP)
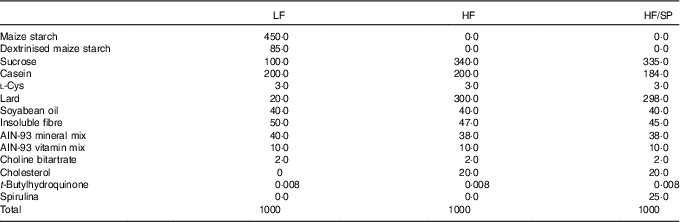
Oral glucose tolerance test
Oral glucose tolerance was assessed in the mice on an experimental diet for 18 weeks after a 6 h fast. Tail blood glucose was measured using an Accu-Chek Aviva glucometre at 0, 15, 30, 60, 120 and 180 min after administration of 2 g/kg body weight by oral gavage. The AUC was calculated for each mouse using GraphPad Prism 6.0 (GraphPad Software Inc.) and then averaged for each group.
Indirect calorimetry
After 19 weeks on one of the experimental diets, mice were subjected to indirect calorimetry to measure activity and energy expenditure using the Oxymax Comprehensive Lab Animal Monitoring System (CLAMS; Columbus Instruments). Mice were singly housed in each CLAMS unit for a total of 72 h during which they had access to their respective diet and water ad libitum. The CLAMS units were located in a temperature-controlled cabinet that was kept at 22°C on a 12 h light–12 h dark cycle. The first 48 h served as acclimation time for the mice, and data from this period were not used for analysis. Average VO2, VCO2, respiratory exchange ratio, energy expenditure, X-axis and Y-axis movement were calculated by averaging all measurements in the light and dark cycle during the last 24 h period.
Total RNA isolation and quantitative real-time PCR
Total RNA was extracted from tissues using TRIzol reagent (Thermo Fisher) following the manufacturer’s protocol. Reverse transcription for complementary DNA synthesis and quantitative real-time PCR analysis were performed as previously described( Reference Park, Rasmussen and Ehler 24 , Reference Rasmussen, Blobaum and Park 25 ) using a Bio-Rad CFX96 Real-Time System (Bio-Rad). Primers were designed according to the GenBank database using the Beacon Designer 7 software (PREMIER Biosoft International). Primer sequences are available upon request.
Histological analysis
Formalin-fixed livers were sent to the Connecticut Veterinary Medical Diagnostic Laboratory (CVMDL) at the University of Connecticut for paraffin embedding and sectioning. Sections were then stained with haematoxylin and eosin (H&E) or Gömöri trichrome by the CVMDL. Slides of stained sections were then imaged with a Zeiss Axio Vert A1 equipped with an MRc camera and 10× objectives.
Splenocyte isolation
Spleen samples were harvested from mice on experimental diets after 20 weeks at the day of killing as previously described, with minor modification( Reference Ku, Pham and Park 22 ). Erythrocytes were removed with Histopaque 1083 (MilliporeSigma) in a 15 ml conical tube and centrifuged at 300 g at 25°C for 30 min with deacceleration turned off. Splenocytes were then carefully removed from the opaque interface and washed two times with cold Roswell Park Memorial Institute (RPMI) media. Splenocytes were then plated at a concentration of 4·0×106 cells/well and treated with or without 500 ng/ml of LPS (MilliporeSigma) for 20 h in RPMI media.
Liver lipid extractions and serum chemistries
Liver lipids were extracted into chloroform–methanol (2:1) and solubilised in Triton X-100 as previously described( Reference Akahoshi, Hashizume and Tanoue 24 ). Both liver lipids and serum lipids were determined enzymatically using Cholesterol Reagent (Pointe Scientific) and an L-Type TG M Kit (Wako Chemicals) for total cholesterol (TC) and TAG, respectively. Serum alanine aminotransferase (ALT) activity was measured using liquid ALT (SGPT) Reagent Set (Pointe Scientific). Serum glucose was measured using Liquid Glucose (Oxidase) Reagent Set (Pointe Scientific).
Statistical analysis
The number of animals per group (n 15) was estimated using a power analysis based on our preliminary SP mouse study using apoE knockout mice to detect a 20 % difference in IL-6 secretion from splenocytes at 80 % power with a two-tailed level of significance at P=0·05 (159·6 (sem 61·1) pg/ml). One-way ANOVA with Tukey’s post hoc test or Student’s t test was used to detect significant differences in treatments, with P<0·05 considered significant by GraphPad Prism 6 (GraphPad Software Inc.). Data are expressed as means and standard errors of the mean.
Results
Effect of Spirulina platensis supplementation on liver fibrosis
Mice fed the experimental diets progressively gained weight over the 20-week feeding period (Fig. 1(A)). While mice fed HF and HF/SP diets gained significantly more weight than mice on the LF diet, their body weights were not significantly different from each other. At the time of killing, liver weights were significantly higher in HF and HF/SP groups compared with the LF group, while eWAT weights were not different between the groups (Fig. 1(B) and (C)).
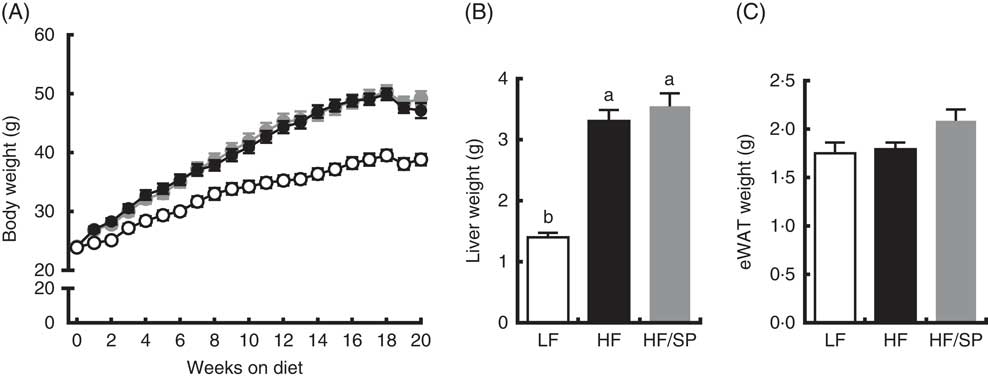
Fig. 1 Effect of Spirulina platensis (SP) supplementation on body and tissue weights in a mouse model of liver fibrosis. Mice fed a low-fat (LF, ), a high-fat (HF,
)/high-sucrose/high-cholesterol diet or an HF diet supplemented with 2·5 % (w/w) SP (HF/SP,
) for 20 weeks. (A) Body weight of mice throughout the 20-week feeding period. Liver weight (B) and epididymal white adipose tissue (eWAT) weight (C) after 20 weeks on experimental diets. Values are means (n 12–15 per group), with the standard errors of the mean represented by vertical bars. a,b Mean values with unlike letters were significantly different from each other (P<0·05).
Plasma ALT, a marker of liver damage, progressively increased in HF and HF/SP groups compared with the LF group throughout the feeding period (Fig. 2(A)). At 16 weeks, HF/SP diet-fed mice showed significantly lower plasma ALT than mice on the HF diet. The livers of mice on HF or HF/SP diets had similar levels of TC and TAG accumulation that was significantly higher than those of the LF group (Fig. 2(B)). H&E staining of liver sections corroborated that lipid accumulation in the livers of both HF and HF/SP fed mice were similar (Fig. 2(C), upper panel). Trichrome staining, which stains collagen blue, demonstrated that HF and HF/SP diets induced noticeably more hepatic collagen accumulation compared with the LF control (Fig. 2(C), lower panel). Also, mice on HF and HF/SP diets showed significantly higher mRNA expression of collagen 1α1 than the LF group, with no differences in α-smooth muscle actin expression (Fig. 2(D)).
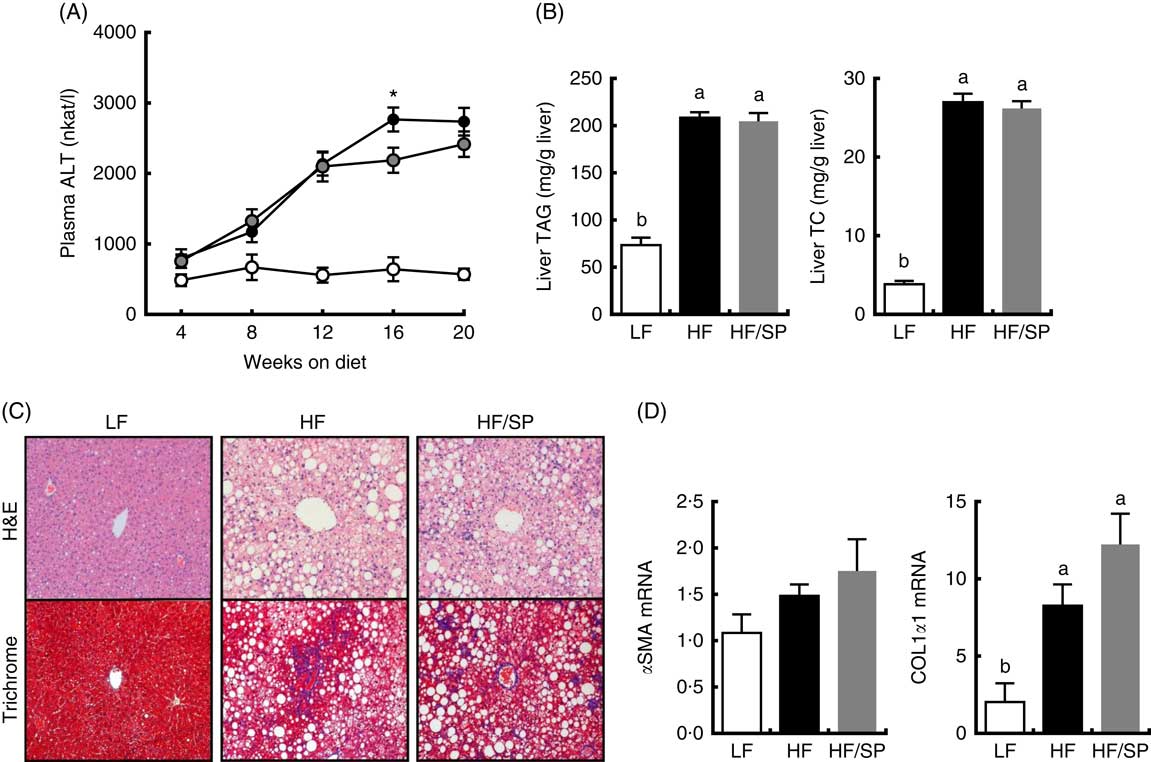
Fig. 2 Effect of Spirulina platensis (SP) supplementation on liver damage, lipid and fibrosis in a mouse model of liver fibrosis. Mice fed a low-fat (LF, ), a high-fat (HF,
)/high-sucrose/high-cholesterol diet or an HF diet supplemented with 2·5 % (w/w) SP (HF/SP,
) for 20 weeks. (A) Plasma alanine aminotransferase (ALT) levels throughout the 20 weeks’ feeding period. (B) Liver TAG (left) and total cholesterol (TC, right) levels. (C) Representative haematoxylin and eosin (H&E) staining (top) and trichrome staining (bottom) of formalin-fixed liver sections. (D) Hepatic expression of α-smooth muscle actin (αSMA) and collagen 1α1 (COL1α1). Values are means (n 12–15 per group), with standard errors of the mean represented by vertical bars. a,b Mean values with unlike letters were significantly different from each other (P<0·05). * Mean value was significantly different from that for the HF/SP group (P<0·05).
Effect of Spirulina platensis supplementation on serum lipid and glucose tolerance
Mice on HF and HF/SP diets showed significantly higher serum TC and glucose concentrations than those of the LF group; however, serum TAG levels were not different between groups (Fig. 3(A)–(C)). Mice fed HF, but not HF/SP, showed significantly impaired glucose intolerance compared with the LF control (Fig. 3(D) and (E)).
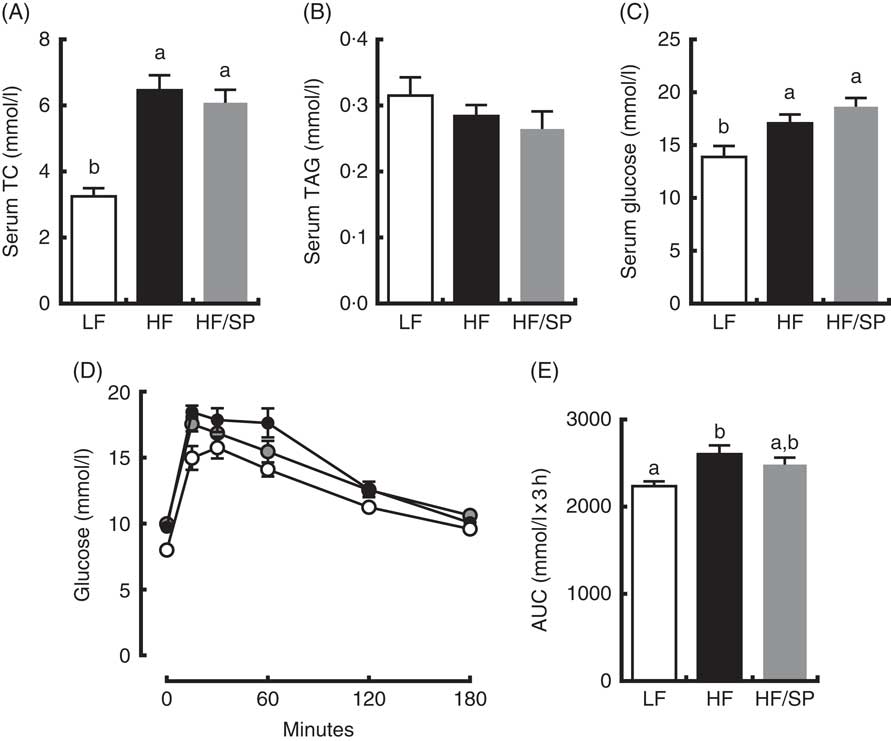
Fig. 3 Effect of Spirulina platensis (SP) supplementation on serum lipids and glucose tolerance in a mouse model of liver fibrosis. Mice fed a low-fat (LF, ), a high-fat (HF,
)/high-sucrose/high-cholesterol diet or an HF diet supplemented with 2·5 % (w/w) SP (HF/SP,
) for 20 weeks. Serum levels of total cholesterol (TC) (A), TAG (B) and glucose (C). (D) Oral glucose tolerance test was conducted 18 weeks after mice were on the experimental diets. (E) The AUC analysis of oral glucose tolerance test. Values are means (n 12–15 per group), with standard errors of the mean represented by vertical bars. a,b Mean values with unlike letters were significantly different from each other (P<0·05).
Effect of Spirulina platensis supplementation on energy metabolism
We determined metabolic rates and physical activities of mice on the experimental diets. Mice on the LF diet had a higher rate of O2 consumption and CO2 production than those of HF and HF/SP groups, despite no significant differences in their activity (data not shown), indicating that their metabolic rates are higher than HF and HF/SP fed mice (Fig. 4(A)–(C)). Also, mice on HF and HF/SP diets had significantly lower respiratory exchange ratio compared with the LF group, suggesting that they favour fat over carbohydrate as energy substrates (Fig. 4(D)). Mice on the LF diet had significantly higher energy expenditure than those of HF and HF/SP groups in the dark cycle (Fig. 4(E)).
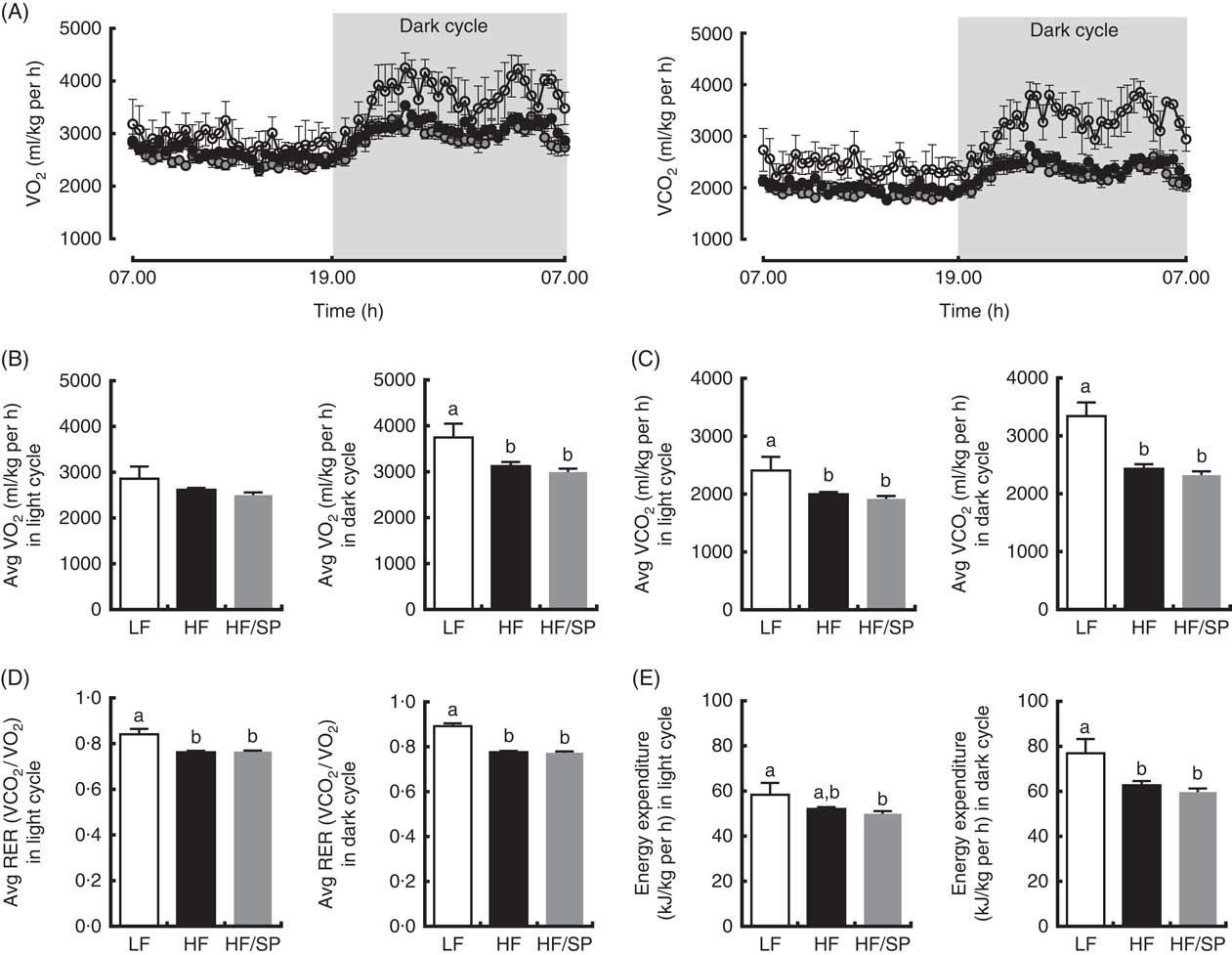
Fig. 4 Metabolic rates of mice fed Spirulina platensis (SP). Mice fed a low-fat (LF, ), a high-fat (HF,
)/high-sucrose/high-cholesterol diet or an HF diet supplemented with 2·5 % (w/w) SP (HF/SP,
) for 19 weeks and they were subjected to indirect calorimetry analysis. (A) 24 h VO2 and CO2 production rate. (B) Average VO2 rate in light and dark cycle. (C) Average CO2 production rate in light and dark cycle. (D) Average respiratory exchange ratio (RER) of mice in light and dark cycle. (E) Energy expenditure in light and dark cycle. Values are means (n 4 for LF, 8 for HF and HF/SP), with standard errors of the mean represented by vertical bars. a,b Mean values with unlike letters were significantly different from each other (P<0·05).
Effect of Spirulina platensis supplementation on basal and lipopolysaccharide-stimulated inflammatory gene expression of splenocytes
The spleen contributes to the development of liver fibrosis as a source of immune cell migration and splenic cytokine secretion via portal vein blood flow( Reference Li, Duan and Chen 11 , Reference Akahoshi, Hashizume and Tanoue 26 ). Spleen weight was significantly increased by HF and the HF/SP diets compared with the LF diet (Fig. 5(A)), suggesting that mice on the diets were likely to experience portal hypertension, which is characteristic of liver fibrosis( Reference Bolognesi, Merkel and Sacerdoti 27 , Reference Leuschner, Rauch and Ueno 28 ). Splenocytes isolated from mice on the HF/SP diet had significantly lower expression levels of basal IL-1β compared with those isolated from the HF group (Fig. 5(B)), while a decrease in IL-6 expression by SP was trending towards significance. Also, the splenocytes from mice on the HF or HF/SP diet for 16 weeks were tested ex vivo for their LPS sensitivity. The splenocytes from mice fed the HF/SP diet had significantly lower mRNA levels of LPS-induced IL-1β and TNF-α as well as TNF-α secretion compared with those from the HF group (Fig. 5(C) and 5(D)).
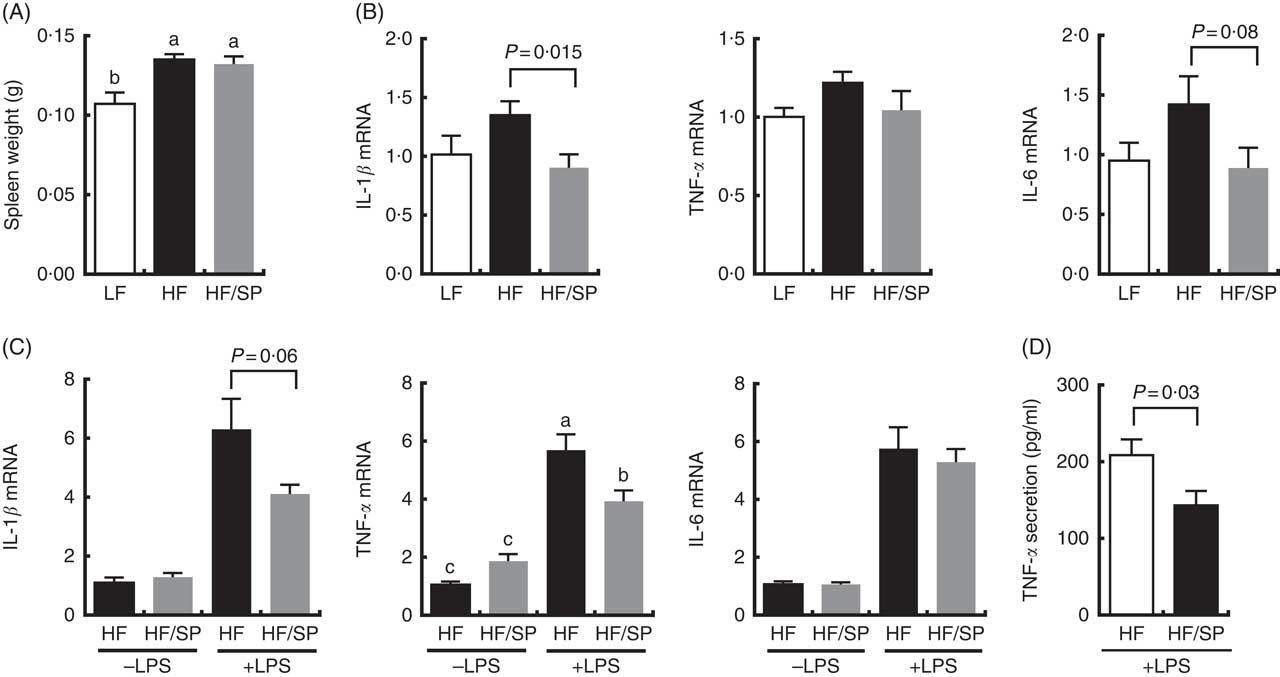
Fig. 5 Anti-inflammatory effects of SP in splenocytes from a mouse model of liver fibrosis. Mice fed a low-fat (LF), a high-fat (HF)/high-sucrose/high-cholesterol diet or an HF diet supplemented with 2·5 % (w/w) SP (HF/SP) for 16 or 20 weeks. Spleen weight (A) and mRNA expression of pro-inflammatory cytokines in the splenocytes (B) of mice on experimental diets for 20 weeks. n 8–9 per group. Splenocytes were isolated from mice on the experimental diets for 16 weeks, and subsequently, they were stimulated with 500 ng/ml of lipopolysaccharide (LPS) for 20 h to measure the expression of pro-inflammatory cytokines (C) and TNF-α secretion (D). n 8–10 per group. Values are means, with standard errors of the mean represented by vertical bars. a,b,c Mean values with unlike letters were significantly different from each other (P<0·05).
Discussion
NAFLD is a major global health problem with few available treatments( Reference Younossi, Anstee and Marietti 1 ). Therefore, prevention of NAFLD is important to minimise global health burden. In the present study, we used an obesogenic diet high in fat, sucrose and cholesterol to induce liver fibrosis for the investigation of the role of SP in the prevention of diet-induced liver fibrosis. We found that SP supplementation had a minimal effect on blood and tissue lipids, whole body energy metabolism and liver fibrosis in this mouse model. However, supplementation of SP reduced basal and LPS-induced inflammatory gene expression and cytokine secretion in splenocytes, supporting the anti-inflammatory effect of SP in vivo. Although there were no appreciable effects of SP in this mouse model, SP may be beneficial for conditions where splenocytes are the source of inflammation such as myocardial infarction( Reference Kim, Yang and Beltran 29 ), ischaemic brain injury( Reference Hsiao, Fernandez and Tanaka 30 ) and ischaemia–reperfusion injury( Reference Yang, Park and Cassada 31 ). Furthermore, conditions that involve chronic inflammation such as colitis and arthritis may also benefit from SP supplementation.
In the current study, SP supplementation did not alter blood or liver lipid levels. This is consistent with the findings from our previous studies with SP in several mouse models( Reference Ku, Kim and Pham 23 , Reference Pham, Park and Bae 32 , Reference Swirski, Nahrendorf and Etzrodt 33 ). Supplementation of 2·5 % (w/w) or 5·0 % (w/w) SP to an AIN-93G/M diet for 6 months in C57BL/6J mice did not alter plasma lipids or liver lipids( Reference Pham, Park and Bae 32 ). In apoE knockout mice, supplementation of 5·0 % (w/w) SP in an HF/high-cholesterol diet for 12 weeks did not alter plasma or liver lipids, while it reduced atherosclerosis development( Reference Ku, Kim and Pham 23 ). In another study, when C57BL/6J mice were fed an HF/high-sucrose diet supplemented with or without 0·25 % SPE for 16 weeks, there were no changes in plasma lipids by SPE( Reference Swirski, Nahrendorf and Etzrodt 33 ). However, we observed a significant reduction in inflammatory gene expression in splenocytes and peritoneal macrophages from mice fed SPE. Collectively, our studies including the present study consistently indicate that SP has a minimal effect on hyperlipidaemia, but it exerts a potent anti-inflammatory function.
The ‘two-hit model’ for NAFLD development and progression includes the accumulation of fat in the liver, that is, the first hit, followed by the second hit, which includes oxidative stress, lipid peroxidation, pro-inflammatory cytokines, adipokines and mitochondrial dysfunction( Reference Paschos and Paletas 4 ). Although in the current study, we did not observe a reduction in the first hit, that is, lipid accumulation, we found that the splenocytes from mice fed SP had significantly lower basal and LPS-induced inflammatory gene expression. This finding is of significance as the spleen has been demonstrated to be a reservoir for monocytes that rapidly mobilise to sites of tissue injury( Reference Li, Wei and Li 34 ), including the liver( Reference Mandal, Gardner and Sun 15 ). Given the association of the spleen and the liver via the portal vein system, such that splenic secretions pass through the liver and to the heart and then on to the general circulation( Reference Li, Duan and Chen 11 ), the spleen may play a significant role in the development of liver fibrosis. Indeed, it has been suggested that splenic red pulp macrophages are a major source of TGF-β1 and that splenectomy decreased serum TGF-β levels significantly while reducing liver fibrosis( Reference Rockey 26 ). Furthermore, it has been demonstrated that the spleen supports the infiltration of monocytes into the liver through the secretion of C–C motif chemokine ligand 2 and that splenectomy decreased the infiltration of monocytes to reduce liver fibrosis( Reference Zou, Lai and Zheng 35 ). Therefore, the spleen likely contributes to the development of liver fibrosis via monocyte infiltration and cytokine secretion and that SP may attenuate this liver inflammation through affecting the spleen.
The notable effect of SP supplementation observed in the present study is that SP reduced the basal and LPS-induced expression of inflammatory genes in splenocytes. This observation is consistent with the findings in our previous studies that demonstrated the anti-inflammatory effect of SP supplementation or its extract in the spleen( Reference Ku, Pham and Park 22 , Reference Swirski, Nahrendorf and Etzrodt 33 ). As mentioned previously, in apoE knockout mice, we found that SP supplementation reduced the secretion of IL-6 from splenocytes and the formation of aortic lesions without altering plasma lipids( Reference Ku, Pham and Park 22 , Reference Ku, Kim and Pham 23 ). Furthermore, SP supplementation significantly lowered the cluster of differentiation 68 (CD68)-stained area in the aortic root, suggesting reduced monocyte infiltration( Reference Ku, Pham and Park 22 ). Considering the role of the spleen as a reservoir of monocytes that deploys to the site of injury( Reference Li, Wei and Li 34 ), it is possible that SP may reduce the infiltration of monocytes to damaged tissues. It is noteworthy that we observed that SP supplementation has reduced inflammatory gene expression and cytokine secretion across several mouse models and experimental diets. This suggests that SP possesses a robust and spleen-specific effect that warrants further investigation.
Reduction of inflammatory gene expression by SP may be due to changes in the population of immune cells found in the spleen, which has been demonstrated to change in response to obesity( Reference Boi, Buchta and Pearson 36 , Reference Ronda and Lele 37 ). Alternatively, components found in SP may also reduce inflammatory gene expression in splenocytes by inhibiting the Toll-like receptor 4 signalling pathways. Indeed, SP has been demonstrated to contain high levels of γ-linolenic acid( Reference Chang, Sun and Lii 38 ), which can reduce LPS-induced inflammatory gene expression in monocytes and macrophages( Reference Chang, Sun and Lii 39 , Reference Furse, Rossetti and Seiler 40 ). In addition, SP has high levels of phycocyanin and carotenoids that can exert anti-inflammatory effects( Reference Cherng, Cheng and Tarn 41 – Reference Ciccone, Cortese and Gesualdo 43 ). It is possible that the anti-inflammatory effect of SP in the spleen is due to one of these bioactive components but could also be the net result of some or all of them. Work in our laboratory is underway to identify the anti-inflammatory bioactives in SP.
Splenic macrophages have been proposed to be a major contributor of cytokines in the development of liver fibrosis( Reference Rockey 26 ). Despite reductions in inflammatory gene expression in splenocytes from mice fed the HF/SP diet for 16 weeks, the lack of reductions in the expression of fibrogenic genes in the liver by the diet after 20 weeks suggests that the anti-inflammatory effect of SP could not overcome the deleterious effect of the HF diet. In the current diet-induced model of liver fibrosis, as there are multiple stressors for liver damage, it is possible that a mere reduction in splenocyte inflammatory gene expression may not be sufficient to overcome other sources of inflammation such as Kupffer cells and bone marrow-derived macrophages. At 16 weeks, mice on the HF/SP diet had significantly lower plasma ALT compared with the HF control, which was not observed at 20 weeks. This suggests that SP supplementation at 2·5 % (w/w), which is equivalent to about 10 g of SP a day for humans, exerted hepatoprotective effects but only up to a certain point and that a higher supplemental level of SP may be effective in reducing liver fibrosis.
In conclusion, we demonstrated that SP at the supplementation level of 2·5 % (w/w) did not prevent the development of liver fibrosis induced by a diet high in fat, sucrose and cholesterol in mice. However, SP supplementation reduced basal and LPS-induced inflammatory gene expression and secretion in splenocytes. Our data suggest that SP exerts anti-inflammatory effects, especially in the spleen. Whether a higher supplementation level of SP may prevent the development of liver fibrosis is warranted. Also, further studies are needed to evaluate the role of SP supplementation in mitigating other inflammatory diseases or conditions where spleen is the primary source of inflammation.
Acknowledgements
This study was supported by USDA NIFA (2017-67012-26096) to T. X. P. and USDA Multistate Hatch CONS 00916 to J.-Y. L.
T. X. P., Y. L, M. B., S. H., H. K., M.-B. K. and Y.-K. P. carried out experiments and performed data analysis; T. X. P., Y.-K. P. and J.-Y. L. wrote the manuscript and J.-Y. L. designed and led the study.
The authors declare that there are no conflicts of interest.