The World Health Organization recommends daily iron supplementation for infants and children (6 months – 12 years) and for menstruating, non-pregnant females, particularly in settings where the prevalence of anaemia is greater than 40 %(1,2) . Although universal iron supplementation is an effective approach to prevent iron deficiency and iron deficiency anaemia, safety concerns have been raised with these recommendations in areas with a high infection burden(Reference Georgieff, Krebs and Cusick3). Based on the low cost and high bioavailability and efficacy, iron sulphate (FeSO4) is typically the first choice for supplementation and fortification; however, other inorganic iron sources are also commonly used(Reference Hurrell4). The recommended dose of iron is set high to deliver adequate absorbed iron due to the low rate of dietary iron absorption(Reference Paganini and Zimmermann5), which is typically <10 %(Reference Zimmermann and Hurrell6). Thus, the majority of dietary iron is not absorbed and travels to the colon. Unabsorbed iron in the colon may select for enteric pathogens at the expense of beneficial commensal bacteria and increase infection risk, including the clinical incidence of diarrhoea(Reference Paganini and Zimmermann5,Reference Zimmermann, Chassard and Rohner7–Reference Kortman, Mulder and Richters9) .
Acquisition of iron is important for the proliferation and virulence of most enteric Gram-negative bacteria (e.g. Salmonella, Shigella, pathogenic Escherichia coli and Campylobacter jejuni). Enteric pathogens employ several mechanisms to scavenge iron from the host, such as synthesising iron-chelating siderophores that bind iron with high affinity, and through various iron uptake and efflux systems. One of the main ways pathogens regulate iron homeostasis is through transcriptional regulation of genes mediated by the ferric uptake regulator (Fur)(Reference Seo, Kim and Latif10). When intracellular iron concentrations are high, the Fur protein acts as a transcriptional repressor by binding to specific sequences in the promoter and preventing transcription of Fur-regulated genes (e.g. tonB, feoB, fimA and invA). In iron deplete conditions, Fur dissociates from the Fur box in the promoter and allows transcription of Fur-regulated genes to overcome iron limitation. In vitro studies consistently show greater proliferation of Gram-negative bacteria when cultured with inorganic iron sources(Reference Kortman, Boleij and Swinkels11,Reference Tan, Chekabab and Yu12) . Preclinical rodent studies have modelled an iron-restricted and iron-rich environment in the lower intestine by feeding varying levels of iron prior to oral inoculation with an enteric pathogen(Reference Kortman, Mulder and Richters9,Reference Ippolito, Piccolo and Robeson13) . These studies have demonstrated iron-induced shifts in the gut microbiota, greater growth of enteric pathogens and a more severe enteropathy in mice fed diets fortified with FeSO4 compared with a low iron diet(Reference Kortman, Mulder and Richters9,Reference Ippolito, Piccolo and Robeson13) . A randomised controlled trial found that iron-containing micronutrient powder provided to 6-month-old Kenyan infants adversely affected the gut microbiome and increased pathogen abundance(Reference Zimmermann, Chassard and Rohner7,Reference Jaeggi, Kortman and Moretti8) . These findings suggest the need for new approaches and alternative iron sources to correct or prevent iron deficiency and iron deficiency anaemia without increasing the risk or severity of infection.
Aspergillus oryzae (Ao) is a filamentous fungus that has the ability to accumulate and store large amounts of iron. Recent research has focused on Ao as a vehicle for iron supplementation and fortification(Reference Reddy, Armah and Stewart14–Reference Bries, Hurrell and Reddy17). In this application, Ao is grown in iron-rich media, harvested and ground into a fine powder. Ao grown in FeSO4 has been shown to have similar absorption to FeSO4 in women of reproductive age with low iron stores(Reference Reddy, Armah and Stewart14). Bries et al. demonstrated that Ao iron is absorbed more slowly and produces less non-transferrin-bound iron 2–8 h post-supplementation compared with FeSO4 (Reference Bries, Wang and Agbemafle16). In addition, the incidence of gastrointestinal side effects was lower after 3 weeks of supplementation with Ao iron compared with FeSO4. While studies have been conducted on the efficacy and safety of Ao, no study to date has determined whether Ao iron is a suitable alternative to inorganic iron in correcting or preventing iron deficiency without contributing to increased risk or severity of infection. The objective of this in vitro study was to determine the effect of Ao iron compared with FeSO4 alone on iron accumulation, growth and virulence of the Gram-negative enteric pathogen, Salmonella enterica subspecies enterica serovar Typhimurium (S. Typhimurium). S. Typhimurium is a rod-shaped, motile facultative intracellular pathogen whose greatest burden is in regions of the world where children are also affected by environmental enteric dysfunction(Reference Feasey, Dougan and Kingsley18).
Methods
Strain and growth conditions
For all experiments, S. enterica subsp. enterica serovar Typhimurium ATCC® 14028™ was cultured in Iscove’s Modified Dulbecco Medium (IMDM; Quality Biological Inc.) at 37°C. IMDM contains no added iron. S. Typhimurium were cultured in media containing IMDM (control) or IMDM containing 1 μM elemental iron as either FeSO4 (BeanTown Chemical) or Ao grown with FeSO4 (Cura Global Health). This concentration represented the lowest dose of iron that produced the greatest difference in growth (online Supplementary Fig. 1) and is consistent with that used in previous studies(Reference Kortman, Boleij and Swinkels11,Reference Tan, Chekabab and Yu12) . The researchers were not blinded to the experimental treatments.
Growth curves
S. Typhimurium were inoculated at 104 CFU/ml. The concentration of inoculated S. Typhimurium was based on a previous study(Reference Tan, Chekabab and Yu12) and optimised to maximise growth potential. S. Typhimurium were maintained in tryptic soy broth and subcultured twice in IMDM to limit residual iron from the tryptic soy broth. A sterile loop was used to transfer S. Typhimurium into a fresh culture tube containing 10 ml IMDM, vortexed and incubated overnight. The overnight culture was then vortexed, and 1 ml of overnight stock was transferred to a fresh tube containing 9 ml IMDM to create a 1:10 dilution of S. Typhimurium. IMDM alone (control) and IMDM containing FeSO4 or Ao iron were transferred into a sterile 15 ml conical tube (9·5 ml; n 6/treatment). Three tubes of each treatment were inoculated with 100 μl of S. Typhimurium using the above dilution; the other tubes were not inoculated with S. Typhimurium and served as blanks. To determine the effect of iron source on the growth of S. Typhimurium, 300 μl from each tube was pipetted into a sterile 96-well plate in triplicate. The 96-well plate was incubated at 37°C in a BioTek Synergy plate reader, and the optical density of the cultures was measured at 600 nm (OD600) every 30 min for 12 h. Plates were shaken before each reading.
Iron accumulation
S. Typhimurium were cultured for 12 h as described above. Bacteria were pelleted, the pellet was rinsed to remove exofacially bound iron and bacteria were pelleted and digested in nitric acid. The iron concentration of the digested pellet was determined using flame atomic absorption spectrometry (Buck Scientific Instruments) and adjusted for OD600 to account for differences in bacterial growth. The iron content of IMDM without added iron was below the limit of detection (<0·01 mg/l).
Real-time quantitative PCR (RT-qPRC)
Expression of Fur-regulated genes (tonB-dependent siderophore receptor, tonB-R; tonB system transport protein, tonB-TP; feoB; fimA; invA) was measured by RT-qPCR. S. Typhimurium were cultured for 12 h as described above. Bacteria were pelleted, and RNA was isolated using TRIzol Reagent (Life Technologies). RNA quality and quantity were assessed using a NanoDrop One Spectrophotometer (Thermo Fisher Scientific). RNA was reverse transcribed with the High-Capacity cDNA Reverse Transcription Kit (Applied Biosystems) according to the manufacturer’s instructions. RT-PCR reaction was performed on a LightCycler 96 instrument (Roche Diagnostics Corp.) using 2x RT2 Green qPCR MasterMix (Qiagen). The RT-PCR amplification was performed in 10 µl reaction volume, using 0·15 µM of each primer (Table 1). The amplification consisted of an initial denaturation step of 900 s, followed by forty-five cycles of denaturation at 95°C for 15 s and annealing at 60°C for 30 s. All PCR amplification was performed with 20 ng of cDNA. Data were normalised to 16S. The following equation was used to determine change in cycle quantification: ΔCq = CqGene – Cq16S. Fold change was calculated using the ΔΔCq method.
Table 1. Primer sequences
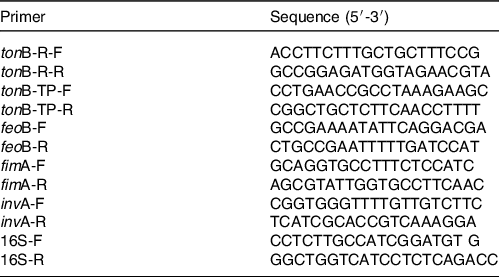
Motility
S. Typhimurium was cultured, pipetted into a 96-well plate and incubated at 37°C in a BioTek Synergy plate reader as described above. Optical density of the cultures was measured every 30 min until the point in the exponential growth phase where differences in growth between S. Typhimurium cultured in FeSO4 and Ao iron were greatest (∼7 h). Microscope slides were treated with 0·1 % TWEEN 20 (Sigma-Aldrich), dried and rinsed with ddH2O. S. Typhimurium (5 μl) was transferred from the 96-well plate to the slide (n 5 slides/treatment). A slide cover was placed on top of the slide, and the outer edges were sealed with nail polish to prevent airflow. Phase-contrast imaging was used to directly visualise bacterial motion in real time, and motion was recorded via a high-speed camera (n 5 videos/slide). The resulting videos were analysed using 2D tracking algorithms using NIS-Elements AR Analysis software (Nikon Instruments Inc.) to extract information such as velocity, acceleration and distance travelled of peritrichously flagellated S. Typhimurium. Particles tracked for less than 1 s were removed from the analysis and S. Typhimurium with a velocity greater than 3 μm/s were considered motile(Reference Tovaglieri, Sontheimer-Phelps and Geirnaert19).
Statistics
Statistics were performed using GraphPad Prism, version 9.3.0. Data are presented as means ± SD for the number of samples reported. Experiments were repeated 2–3 times. Comparisons between iron source (control, FeSO4 or Ao iron) were performed using one-way ANOVA. Two-way ANOVA was used to determine the effects of iron source or concentration, time and their interaction. If a significant main effect was observed, post hoc comparisons were made using Tukey’s test. Data were tested for normality using Shapiro–Wilk’s test, and log-transformed data were used if data were not normally distributed. The α level for statistical significance was set at P < 0·05.
Results
Ao iron limits iron accumulation in S. Typhimurium compared with FeSO4
S. Typhimurium cultured with FeSO4 accumulated ∼200 % more iron than those cultured in control media (P < 0·0001) and with Ao iron (P < 0·0001; Fig. 1). Iron accumulation did not differ in S. Typhimurium cultured in control media and with Ao iron (P = 0·64).
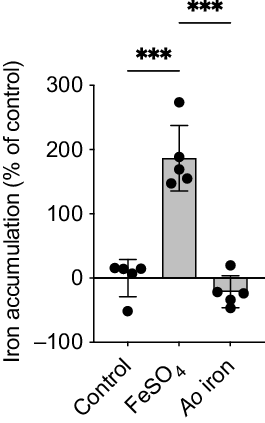
Fig. 1. Iron accumulation is reduced in S. Typhimurium cultured with Ao iron compared with FeSO4. Iron accumulation in S. Typhimurium cultured in media containing no added iron (control) or 1 μM of elemental iron as either FeSO4 or Ao iron for 12 h. Data were analysed using a one-way ANOVA. Asterisks indicate a significant post hoc comparison (*P < 0·05; **P < 0·01; ***P < 0·001). Data are means ± SD; n 5/treatment.
Fur-regulated genes are upregulated with Ao iron compared with FeSO4
Consistent with decreased iron accumulation in S. Typhimurium cultured with Ao iron, genes regulated by the iron-activated transcriptional repressor, Fur, were upregulated with Ao iron compared with FeSO4. tonB-R increased ∼1-fold with Ao iron compared with controls (P < 0·001) and decreased 48- and 26-fold with FeSO4 compared with Ao iron (P < 0·0001) and control (P < 0·0001), respectively (Fig. 2(a)). tonB-TP did not differ between Ao iron and controls (P = 0·88), but decreased ∼3- and 5-fold with FeSO4 compared with Ao iron (P = 0·02) and control (P = 0·01), respectively (Fig. 2(b)). feoB did not differ between Ao iron and controls (P = 0·99), but decreased ∼5-fold with FeSO4 compared with Ao iron (P < 0·0001) and control (P < 0·0001; Fig. 2(c)). fimA decreased ∼5- and 7-fold in S. Typhimurium cultured with FeSO4 compared with control (P < 0·0001) and Ao iron (P < 0·0001), respectively; controls and Ao iron did not differ (P = 0·42; Fig. 2(d)). invA did not differ between S. Typhimurium cultured in control media compared with Ao iron (P = 0·88; Fig. 2(e)). There was a 3-fold reduction in invA with FeSO4 compared with both Ao iron (P = 0·02) and control (P = 0·01).

Fig. 2. Fur-regulated genes are upregulated in S. Typhimurium cultured with Ao iron compared with FeSO4. Expression of: (a) tonB-R; (b) tonB-TP; (c) feoB; (d) fimA and (e) invA in S. Typhimurium cultured in media containing no added iron (control) or 1 μM of elemental iron as either FeSO4 or Ao iron for 12 h. Data were analysed using a one-way ANOVA. tonB-TP and invA were log transformed prior to analysis. Asterisks indicate a significant post hoc comparison (*P < 0·05; **P < 0·01; ***P < 0·001). Data are means ± SD; n 5/treatment.
Ao iron restricts the growth of S. Typhimurium compared with FeSO4
Growth of S. Typhimurium was greater in the presence of FeSO4 compared with controls (P < 0·0001), whereas growth was reduced in the presence of Ao iron compared with FeSO4 (P < 0·0001; Fig. 3). Time to reach peak doubling time was also greater with FeSO4 (5·5 ± 0·0 h) than Ao iron (4·6 ± 0·3 h; P = 0·0001) and control (4·5 ± 0·4 h; P < 0·0001), but did not differ between Ao iron and control (P = 0·96). Doubling time during the exponential growth phase was shorter with FeSO4 (35·5 ± 2·6 min) compared with Ao iron (57·3 ± 3·9 min; P < 0·0001) and control (49·5 ± 8·4 min; P = 0·001). Doubling time during the exponential growth phase did not differ between Ao iron and control (P = 0·06).

Fig. 3. Ao iron restricts the growth of S. Typhimurium compared with FeSO4. Growth of S. Typhimurium cultured in media containing no added iron (control) or 1 μM of elemental iron as either FeSO4 or Ao iron. Data were analysed using a two-way ANOVA; main effects: P time<0·0001, P treatment<0·0001, P interaction<0·0001. Letters indicate a significant post hoc comparison (P < 0·05): (a) P < 0·05 FeSO4 compared with control; (b) P < 0·05 Ao iron compared with control; (c) P < 0·05 FeSO4 compared with Ao iron. Data are means ± SD; n 6/treatment/timepoint.
Ao iron reduces motility of S. Typhimurium compared with FeSO4
The velocity of S. Typhimurium cultured with Ao iron was not different from controls (P = 0·07), whereas S. Typhimurium cultured with FeSO4 swam 98 % faster than controls (P < 0·0001; Fig. 4(a)). Acceleration and the distance travelled followed a similar trend. Acceleration was similar between controls and Ao iron (P = 0·46; Fig. 4(b)), but increased by 84 % in S. Typhimurium cultured with FeSO4 compared with control (P < 0·01). Controls and S. Typhimurium grown in Ao iron travelled a similar distance (P = 0·99), whereas S. Typhimurium travelled approximately 65 % further when cultured in FeSO4 compared with control (P = 0·03; Fig. 4(c)).
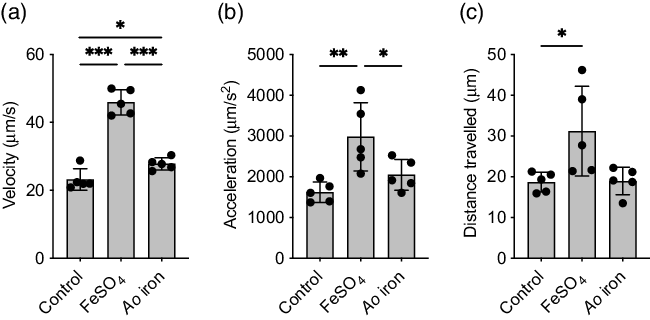
Fig. 4. Ao iron restricts the motility of S. Typhimurium compared with FeSO4. (a) Velocity; (b) acceleration; and (c) distance travelled of S. Typhimurium cultured in media containing no iron (control) or 1 μM of elemental iron as either FeSO4 or Ao iron for 7 h. Data were log transformed prior to analysis and analysed using a one-way ANOVA. Asterisks indicate a significant post hoc comparison (*P < 0·05; **P < 0·01; ***P < 0·001). Data are means ± SD; n 5/treatment.
Discussion
During the course of infection, enteric pathogens compete with the host to obtain essential nutrients, such as iron. The current study shows for the first time that Ao iron attenuates iron accumulation, growth and motility of the common enteric pathogen S. Typhimurium compared with FeSO4 alone. The current study also offers further evidence that enteric pathogens efficiently accumulate inorganic iron sources, such as FeSO4, which contributes to their growth and motility. As previous studies in human subjects have demonstrated that iron absorption is similar between Ao iron and FeSO4 (Reference Reddy, Armah and Stewart14), findings from this study provide preliminary in vitro evidence that iron-enriched Ao may be a suitable iron supplement to improve iron delivery in areas with a high infection burden.
Acquisition of iron is necessary for the growth, survival and virulence of Gram-negative enteric pathogens(Reference Andrews, Robinson and Rodríguez-Quiñones20). Multiple in vitro studies demonstrate increased growth of enteric pathogens in the presence of inorganic iron, such as ferric citrate and ferric chloride, whereas tonB− and iroN− fepA− mutants with an iron uptake defect have reduced growth when presented with iron compared with wild types(Reference Kortman, Boleij and Swinkels11,Reference Tan, Chekabab and Yu12) . Findings from the current study are consistent with these findings in that greater iron accumulation and growth was observed in the presence of FeSO4 compared with control. Importantly, we extend these findings and demonstrate that iron accumulation and growth of S. Typhimurium are restricted with Ao iron. Bries et al. reported that >90 % of the iron taken up by Ao is found within the mycelia and propose that iron from the complex fungal matrix is slowly absorbed over a longer period of time(Reference Bries, Wang and Agbemafle16). Increased iron accumulation with FeSO4 compared with Ao iron was confirmed by the observation that Fur-regulated genes were repressed with FeSO4, but were similar to control or greater with Ao iron. Fur monitors intracellular iron levels and regulates the transcription of genes involved in iron acquisition and utilisation and other cellular functions(Reference Seo, Kim and Latif10). For example, tonB interacts with iroN and energises transport of ferric iron–siderophore complexes into the periplasm of Gram-negative bacteria(Reference Braun and Hantke21,Reference Brewer, Tolley and Trayer22) , and feoAB is a ferrous iron transporter consisting of the inner membrane transporter feoB and the cytosolic protein feoA, which aids feoB activity(Reference Cartron, Maddocks and Gillingham23). Collectively, these findings suggest that iron within Ao is sequestered, perhaps in the vacuole, and potentially unavailable to enteric pathogens, and may provide a mechanism underlying the reduction in iron accumulation and growth with Ao iron.
The ability of enteric pathogens to be motile is critical for scavenging nutrients, such as iron, in a competitive environment. Motility also enables bacteria to reach the intestinal epithelium and adhere to and invade cells(Reference Jones, Richardson and Uhlman24,Reference Stecher, Hapfelmeier and Müller25) . In the current study, measures of motility (velocity, acceleration and distance travelled) were greater in S. Typhimurium cultured with FeSO4 compared with control and Ao iron, which did not differ from one another. These findings are consistent to those of Bearson et al. (2010) who reported increased motility in Salmonella cultured in media supplemented with 80 μM ferric chloride(Reference Bearson, Bearson and Lee26); however, we extend these findings to show that Ao iron restricts the motility of S. Typhimurium when compared with FeSO4.
The amount of iron provided in micronutrient powders and fortification regimens balances the need to prevent or correct iron deficiency while minimising the risk of enhanced bacterial growth and virulence. Findings from the current study indicate that iron-enriched Ao restricts iron accumulation and virulence of a Gram-negative enteric pathogen in vitro. The finding that growth and motility with Ao iron were more similar to controls cultured without iron than to those cultured with FeSO4 provide promise that Ao iron may be an effective alternative to FeSO4. However, there are limitations to our findings. First, the current study used S. Typhimurium as a model Gram-negative enteric pathogen. Whether Ao iron has similar effects on other Gram-negative pathogens remains to be determined. Similarly, examining iron accumulation, growth and motility in pathogens that do not require iron may provide further insight into the mechanism by which Ao iron is withheld. Findings from the current in vitro study also need to be validated in a more complex in vivo model. One important question is the nature of Ao iron after digestion. The slow release of iron into the blood and lower non-transferrin-bound iron with Ao iron compared with FeSO4 (Reference Bries, Wang and Agbemafle16) suggests that the fungal matrix is slowly digested and that at least some iron is still sequestered in mycelia, but this has not been specifically tested. These and other questions are important for determining whether iron-enriched Ao is a suitable supplement or fortificant to improve iron delivery in areas with a high infection burden.
Acknowledgements
The authors thank Cura Global Health, Inc. for the Ao iron.
Supported by intramural funds to S. R. H. and a Multidisciplinary Support grant through the Council on Research and Creativity at Florida State University to S. R. H., J. A. and P. S. J. A. acknowledges support from the National Science Foundation (HDR-2000202 and CMMI-2000330).
S. R. H. conceived and designed the study; J. A., P. S. and S. R. H. obtained funding for the study; K. M. M. led the study as part of her M.S. Thesis; K. M. M., D. Q. and F. J. V. conducted the experiments; K. M. M., M. B. R., P. S. and S. R. H. interpreted the data; K. M. M. and S. R. H. wrote the manuscript with input from all authors. S. R. H. had primary responsibility for final content. Bacterial motility experiments were performed at the National High Magnetic Field Laboratory, which is supported by National Science Foundation Cooperative Agreement Nos. DMR-1157490 and DMR-1644779 and the State of Florida.
M. B. R. is a Scientific Advisor to Cura Global Health, Inc.; K. M. M., D. Q., F. J. V., J. A., P.S. and S. R. H. report no conflicts.
Supplementary material
For supplementary material/s referred to in this article, please visit https://doi.org/10.1017/S000711452200335X