INTRODUCTION
Bovine tuberculosis (bTB) is one of the most relevant epizootics worldwide and leads to significant losses in animal production. Mycobacterium bovis and M. caprae are the primary causative agents in countries of Central and Southern Europe. Both species are zoonotic and 99·9% genetically identical to M. tuberculosis. Together with other approved and recently described TB-causing mycobacterial species, i.e. M. africanum, M. microti, M. pinnipedii, M. orygis, M. suricattae, M. mungi and the ‘dassie bacillus’, they form the M. tuberculosis complex (MTC) [Reference Alexander1–Reference Parsons7]. Bovine M. microti infections are very rarely seen and appear to be associated with high rodent densities [Reference Jahans8]. Occasionally, infections due to M. tuberculosis have been observed in cattle [Reference Lesslie9–Reference Steele and Steele12]. The prevalence of bTB caused by M. tuberculosis is low, mostly restricted to countries with a high burden of human TB, and normally does not exceed 1% of bTB herd incidents (‘breakdowns’; summarized in [Reference Ocepek13]). The route of M. tuberculosis transmission to cattle may involve aerosol inhalation from infected humans, but oral transmission by ingestion of feed or water contaminated with infective human nasal secretions, faeces or urine is also possible [Reference Kaneene, Pfeiffer, Thoen, Steele and Gilsdorf14].
In Germany, bTB prevalence as high as 59% at farm level was recorded for the first half of the last century [Reference Meyn15]. National eradication programmes were established on a voluntary basis in 1952 in Western Germany and in 1955 in Eastern Germany and later became mandatory. Programmes were based on regular tuberculin skin testing of all cattle aged >6 weeks and removal of reactor animals. Germany finally met the requirements of Article 2(d) of Directive 64/432/EEC by having 99·9% of cattle herds certified bTB-free for at least 6 consecutive years and was subsequently declared officially bTB-free (OTF), effective from 1 July 1996 (Decision 97/76/EC). Consequently, Germany abandoned the elaborate and costly regular tuberculin testing and bTB surveillance was maintained at the level of official veterinary meat inspection instead.
From 1995 to 2012, the annual number of bTB incidents in cattle herds varied between two and 23. Numbers rose to 46 in 2013 (http://tsis.fli.bund.de/Reports/Info.aspx) following an intensified regional surveillance in two counties in Southern Germany bordering Austria. In this region, alpine farming practices were suspected of fostering transmission of M. caprae between free-ranging red deer and cattle. Indeed, an apparent local endemic driven by three different molecular types of M. caprae was discovered which accounted for 73·9% of German bTB cases in 2013 [Reference Domogalla16]. Other cases of bTB notified in 2013 affected farms in North-Western Germany with a wide spatial distribution and were exclusively attributed to M. bovis [Reference Probst17]. For a deeper insight into the true prevalence of bTB, a nationwide survey was prescribed due to a revision to the German Regulation on bTB issued on 12 July 2013. Between July 2013 and 30 April 2014, approximately 3350 cattle aged >2 years were selected from each federal state for single intradermal comparative cervical tuberculin (SICCT) testing. This nationwide survey revealed a suspicious case of bTB in a dairy farm, which was eventually attributed to exposure to M. tuberculosis. This case, described here, led to significant difficulties in case declaration and implementation of appropriate legal measures and was accompanied by substantial financial losses for the affected farm.
METHODS
In September 2013 the study herd consisted of 234 cattle in total. Besides two fattening pigs for personal consumption, some cats were also kept on the farm and four persons regularly cared for the animals. Fifteen bovines aged >2 years were subjected to an intra vitam diagnosis of bTB. SICCT testing was performed with matched equipotent doses of bovine and avian purified protein derivative (PPD), respectively. Increase in skin thickness was measured 72 h after injection, and the reaction was interpreted as positive if clinical signs were observed or there was an increase in the thickness of the skin fold at the injection site together with a positive reaction to bovine PPD being >4 mm greater than the reaction to avian PPD. Inconclusive reactors (1-4 mm thickness increase) were re-tested by interferon-gamma release assay (IGRA; Bovigam®, Prionics, Germany) within 3 weeks according to the manufacturer's instructions. If the IGRA result was positive, the animal was culled. Animals testing negative by IGRA were re-tested by SICCT and culled if reacting inconclusive. Pathological examinations were performed on the carcases and organs with special regard to detection of lesions suspicious for bTB. For molecular and histological investigations, tissues of 11 prescribed organs and lymph nodes (lung, intestine, liver, spleen, kidney and respective tributary lymph nodes as well as retropharyngeal lymph nodes) were subjected to polymerase chain reaction (PCR) (duplex real time PCR for IS1081 and a hypothetical helicase (HELI) for the detection of MTC members; [Reference Anon18]) and fixed in neutral buffered formalin, paraffin embedded and routinely stained with haematoxylin and eosin, respectively. Sections showing inflammatory alterations, with granulomas in particular, were Ziehl–Neelsen (ZN)-stained for detection of acid-fast bacilli (AFB). Up to 12 tissue samples per animal were collected from cattle (n = 55), pig (n = 1) and cat (n = 1) between September 2013 and January 2014 and screened for pathological lesions, growth and/or genomic evidence for the presence of MTC members. Caseous lymphadenitis of one Ln. mandibularis accessorius was detected at regular slaughter in the pig. Mycobacterial culture was initiated in duplicate in two different laboratories using standard procedures as described in the Official Collection of Diagnostic Methods released by the Friedrich-Loeffler-Institut/Federal Research Institute for Animal Health (FLI) based on DIN 222 standard [Reference Anon18] or with minor modifications. Briefly, tissue samples were homogenized and decontaminated and then inoculated onto three solid (slant) culture tubes [Loewenstein–Jensen medium with and without glycerol, Stonebrink medium (Merck, Germany)] and one liquid medium (Kirchner or Middlebrook broth; Merck) and incubated for up to 12 weeks at 37 °C. To inhibit growth of contaminant flora, all media were supplemented with PACT (polymyxin B, amphotericin, carbenicillin, and trimethoprim). Mycobacterial cultures were verified by duplex real-time PCR (IS1081, HELI) for MTC members (according to [Reference Anon18]). Molecular differentiation of members of MTC included spoligotyping [Reference Kamerbeek19] using a recently developed microarray [Reference Ruettger20]. Results were recorded automatically according to the nomenclature of international databases (SITVIT; MIRU-VNTRplus; mbovis.org). From cows with evidence of paratuberculosis, faecal samples were screened for the presence of M. avium subsp. paratuberculosis (MAP) by culture and also directly by real-time PCR (Life Technologies, Germany). Additionally, four milk filters from the milking machine were explicitly tested for MTC members by PCR and culture. Grown colonies of presumptive mycobacteria not belonging to MTC were analysed by 16S rRNA and rpoB gene sequence analysis as described previously [Reference Weisburg21, Reference Kasai, Ezaki and Harayama22]. Additionally, presumptive mycobacterial isolates were submitted to spectroscopic analysis based on matrix-assisted laser desorption/ionization–time-of-flight mass spectrometry (MALDI–TOF MS; Biotyper, BrukerDaltonics, Germany) using a preparation method for mycobacteria according to the manufacturer's instructions.
Data on meat inspection and possible condemnations were requested from the abattoir that had slaughtered cattle from the farm under investigation between September 2013 and March 2014.
RESULTS
Herd history, intra vitam testing in bovines
During the years 2010–2012, the owner of the herd had bought one bull originating from the same federal state. No other bovines were introduced into the herd. Of 15 cattle selected for testing in the mandated nationwide survey in 2013, two animals showed inconclusive reactions upon initial SICCT testing. Both cows were re-tested with negative results by IGRA 3 weeks later. The remaining 174 bovines aged >6 weeks were tested by SICCT in mid-October. Of these, five had an inconclusive result and eight a positive result. Of the former, one reacted positively to a subsequent IGRA test and was culled, as were all of the latter. The next herd survey was conducted at end of November 2013, when 46 and 12 cows tested by SICCT were inconclusive and positive, respectively. All SICCT-positive bovines as well as 33 IGRA-positively re-tested cows were necropsied between December 2013 and January 2014. In January, one additional cow was culled after a positive SICCT test. A detailed scheme of SICCT testing reactivity and IGRA results is given in Figure 1. Altogether, 654 SICCT and 60 IGRA tests were performed on this herd producing 577 and 26 negative and 77 and 34 positive results, respectively.
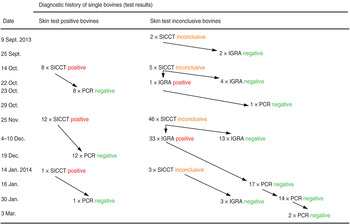
Fig. 1. Overview of test results during testing of a dairy herd (animals with negative test results throughout are not included in the Figure) for the presence of bovines having contact with members of the M. tuberculosis complex. SICCT, Single intradermal comparative cervical tuberculin test; IGRA, interferon-gamma release assay; PCR, double multiplex real-time PCR targeting IS1081 and the gene for a hypothetical helicase each in combination with the gene for β-actin as amplification control with tissue obtained during necropsy.
Abnormal findings in bovines and their environment
Necropsies on 55 (23·5%) of 234 animals revealed no evidence of TB or bTB, neither histologically nor microscopically nor by culture or nucleic acid amplification techniques. ZN staining revealed no acid-fast rod-shaped bacteria in tissue sections from cattle. Mycobacterial growth was not observed in any of the tissue samples (n = 532) from 55 culled cattle up to 12 weeks of incubation. Altogether, analyses of 452 and 543 tissue samples and nine faecal samples were performed for detection of IS1081 and HELI as well as for the detection of MAP, with negative results throughout. Meat inspection of 17 bovines sent for regular slaughter up to March 2014 failed to provide any findings indicative of bTB.
AFB were not detected in tissue samples (n = 9) from one euthanized cat; however, AFB consistent with fast-growing non-tuberculous mycobacteria could be propagated from milk filter samples (n = 4), in which few AFB were detected by microscopy. Partial 16S rRNA and rpoB gene sequencing identified all isolates as pure cultures of M. setense confirmed by mass spectrometry (data not shown).
Abnormal findings in a fattening pig raised on the farm
After regular slaughtering of one fattening pig, caseous lesions were noted in one Ln. mandibularis accessorius during meat inspection. A high-grade amount of AFB was detected in the affected lymph node tissue by direct ZN staining and histopathology. Mycobacterial culture for up to 20 weeks of incubation at 37 °C failed to grow AFB, whereas samples were positive by MTC PCR, yielding positive results with a cycle threshold (Ct) of 33·45. Molecular characterization directly carried out from extracted DNA revealed a M. tuberculosis member of the Euro-American SIT (spoligotype international type) T1 family (SITVIT database). The carcass was condemned.
DISCUSSION
None of the tests currently available for the diagnosis of bTB allow a perfectly accurate determination of M. bovis infection status of cattle. With 99·5% specificity [Reference de la Rua-Domenech23] in TB-free cattle populations, skin tests remain the primary ante-mortem diagnostic tool for bTB. Bovine TB has been eradicated effectively from many countries including Germany following the implementation of programmes of regular tuberculin skin testing and removal of reactors, coupled with slaughterhouse surveillance for undetected infections, and a variety of accompanying measures, e.g. cattle movement restrictions. The deployment of IGRA as an ancillary in vitro test has enhanced the detection of bTB-infected cattle (for a comprehensive overview of different ante-mortem diagnostic techniques for bTB, see de la Rua-Domenech et al. [Reference de la Rua-Domenech23]).
Using primary skin testing and subsequent IGRA testing of reactor cattle, a case of presumptive exposure of livestock to M. tuberculosis in a dairy holding and an infected pig as the probable proximate source of the agent has been uncovered. Although no clinical signs indicative of bTB were detected, exposure of a significant number of cattle was revealed with as many as 55 animals showing delayed type hypersensitivity to bovine PPD. The M. bovis-derived PPD test antigens licenced in Germany for both skin and IGRA testing of cattle contain antigens also present in M. tuberculosis, which are likely to re-stimulate immune cells sensitized for MTC members other than M. bovis. Bovine infections with M. tuberculosis have been sporadically reported from European countries previously and, more recently, from developing countries [Reference Lesslie9, Reference Steele and Steele12, Reference Berg24–Reference Thakur26]. Most infections in cattle seem to be attributed to intensive contact with humans in regions with high incidence of pulmonary TB in humans [Reference Lesslie9–Reference Steele and Steele12, Reference Ameni and Erkihun27–Reference Mittal30], even though the identity of human and bovine strains is only rarely established [Reference Ocepek13, Reference Romero31]. Detection of bovine M. tuberculosis infections is hampered by the absence or weak expression of clinical symptoms and by SICCT responsiveness occurring only transiently [Reference Steele and Steele12, Reference Srivastava25, Reference Romero31]. With the disappearance of the infection source and/or presumptive clearance of the agent, reactivity to tuberculin vanishes quickly [Reference Lesslie9, Reference Ocepek13], thus probably resulting in significant under-reporting. Viable M. tuberculosis bacteria could not be isolated in the context of this case. However, sufficient indication exists to conclude that the high number of reactor animals identified upon repeated testing of the herd resulted from exposure to M. tuberculosis as detected by PCR in the pig.
The majority of cattle in the herd under investigation were SICCT-negative during initial testing in September 2013. Within the following 2 months, the number of reactors markedly increased. This implies recent introduction and highly efficient spread of the causative agent on the premise or continuous presence of the source [Reference McIlroy, Neill and McCracken32]. Other authors found the spread of M. bovis within cattle herds to be relatively inefficient, but other factors, such as the general health status of infected and/or contact animals or the type of animal husbandry, may facilitate disease transmission [Reference Probst17, Reference McIlroy, Neill and McCracken32]. In order to rapidly identify and remove the possible source of infection and considering the high susceptibility of cats towards M. bovis, a cat from the farm was also euthanized [Reference LoBue, Enarson and Thoen33]. None of the cows nor the cat showed typical symptoms of TB and MTC members could neither be detected by PCR nor by bacterial culture, thus strongly arguing against the presence of M. bovis in that herd.
The PCR employed in Germany for official diagnosis targets two gene sequences, IS1081 and HELI. Using two target genes instead of a single one can be assumed to enhance sensitivity and specificity of detection, which is of particular relevance for OTF countries. Subsequent species identification and molecular characterization of the pathogen causing the granulomas in the porcine lymph node was conducted by microarray-based spoligotyping. M. tuberculosis was identified and the pattern detected was assigned to the T1 family. This lineage has previously been identified in human samples from Germany and many other countries. The microarray possesses a superior sensitivity compared to conventional spoligotyping and was able to generate signal patterns directly from the tissue's DNA extract [Reference Ruettger20]. In other suspected bTB cases associated with M. tuberculosis, the authors also failed to cultivate the causative organism [Reference Lesslie9, Reference Smith11] or only succeeded after initial passage in a guinea pig [Reference Lesslie9]. Application of highly sensitive molecular typing methods may therefore help resolve more cases of livestock infections involving MTC members other than M. bovis and M. caprae.
Since the presence of M. tuberculosis on the farm became evident, dynamics of the immunoconversion observed in the cattle herd might best be explained by the presence of an unidentified human shedder with ‘open’ TB on the farm and in proximity to susceptible animals shortly before the nationwide survey started. Quantiferon® (Cellestis Ltd, Germany) testing carried out with the farmer, his family and several contact persons including contract workers and several other contact persons indeed led to the detection of four human reactors within the farmer's family. Although all four tested negative by X-ray examination, the possibility exists that these persons had experienced recent M. tuberculosis infection. The exact route of pathogen introduction remains obscure leaving the question unanswered whether the patients were source or target of bovine exposure to M. tuberculosis. Pigs are more susceptible to M. tuberculosis infection than cattle [Reference LoBue, Enarson and Thoen33]. Thus, the possibility exists that infection of the fattening pig from a human source resulted in amplification of the agent including induction of histopathological lesions, shedding to the farm environment, as well as exposure and subsequent conversion of bovines and probably (even more likely) humans. The lack of positive culture results despite pathological lesions compatible with TB and positive PCR tests has been previously reported for wild boar [Reference Müller34, Reference Capellmann35]. These authors assumed that active TB was no longer present at the time of investigation.
Based on observations during the German bTB eradication campaign in the 1960s, Schliesser expressed the opinion that bovine infections with M. tuberculosis were principally restricted to the formation of primary complexes with limited propagation of the agent in the host [Reference Schliesser, Blobel and Schliesser36], which would result in absent or minor lesions detected at necropsy [Reference Ocepek13, Reference Srivastava25]. Infections do not develop into open stages, thereby confining the infection to single animals and leading to the assumption that cattle represent dead-end hosts for M. tuberculosis [Reference Schliesser, Blobel and Schliesser36]. Indeed, numerous reports on asymptomatic as well as clinical cases of bTB due to M. tuberculosis exist [Reference Lesslie9, Reference Steele and Steele12, Reference Ocepek13, Reference Berg24–Reference Thakur26, Reference Mittal30, Reference Romero31] that merely agree in cattle-to-cattle transmission being uncommon. However, more recent cases with clinical and morphological symptoms, indistinguishable from ‘classical’ bTB, and even detection of M. tuberculosis in bovine milk have been reported repeatedly from countries with a high TB burden [Reference Lesslie9, Reference Srivastava25–Reference Mittal30, Reference Schliesser37]. These findings might indicate cattle as a potential source of M. tuberculosis transmission to humans. The TB incidence in humans is rising by approximately 1% per year [Reference Smith38] and a continuous spillover to cattle might even cause a better adaptation of M. tuberculosis to the bovine host [Reference Mittal30]. This situation may create novel threats, especially in the light of multidrug-resistant strains being isolated from cattle [Reference Romero31]. Immunoconversion in cattle to bTB antigens, which cannot be immediately substantiated by direct detection of M. bovis, should not be dismissed easily as resulting from exposure to environmental mycobacteria and of no further relevance to animal and human health. Instead, public authorities, veterinarians and farmers should be aware of the risk resulting from introduction of M. tuberculosis into populations of susceptible ruminants. The consequences are not restricted to bTB monitoring and eradication programmes, but extend to safety issues concerning animal workers and even consumers. This is particularly relevant for countries regarded free from bTB.
In an attempt to clear the suspected herd breakdown, 55 cows were culled for diagnostic purposes, i.e. nearly a quarter of the whole herd referred to in this report. Those mycobacterial species known to interfere with bTB laboratory diagnosis [Reference Thacker39], particularly M. avium and M. kansasii, were not found in this case. MTC members other than M. bovis and M. caprae, especially M. tuberculosis, are rarely considered as a differential diagnosis for bTB, particularly in countries with low prevalence of both bTB and human TB [Reference Romero31]. Results from the current investigation imply, however, that exposure of bovines to M. tuberculosis may result in high numbers of animals immunoconverting and thus responding to bTB test antigens. In skin testing campaigns, competent authorities may then face severe difficulties in interpreting test results and, depending on the wording of the legal requirements that apply, in decision making on the status of the affected herd. The German Regulation on bTB prescribes confirmation of cases involving positive or inconclusive SICCT or positive IGRA reactors, on the basis of bacterial culture of M. bovis or M. caprae, or detection of specific gene sequences. A positive result of intradermal tuberculin testing alone does not fulfil the case definition. According to the German Regulation on Reportable Animal Diseases (as of 17 April 2014), detection of TB in pigs is classified as reportable only, irrespective of the causative MTC species. In the present study, pathological signs of mycobacteriosis and molecular detection of MTC found in one pig even on a farm with a significant number of reactor cattle did not suffice to justify the declaration of a bTB breakdown. Consequently, grading of the farm as a suspected outbreak had to be reversed after tissue samples from culled cattle were scored negative by PCR and culture and the remaining cattle proved SICCT negative at re-testing in March 2014. More importantly, epidemiological trace-back and trace-on investigations in bovine contact herds lacked a legal basis and, despite strong evidence for bTB at first, these investigations were – in the light of all-negative cattle samples and the proof of M. tuberculosis – merely focused on the human sector. Appropriate handling of such cases may require adaptations of national and international regulations to facilitate eradication measures and compensation payments. Considering the zoonotic potential of all MTC species and the relatively low likelihood of bovine infections with MTC members other than M. bovis and M. caprae being revealed, the spectrum of notifiable bovine infections should be extended to any MTC member. Furthermore, direct detection of MTC in other livestock species with epidemiological links to herds harbouring reactor cattle should be accepted as sufficient evidence to declare a bTB outbreak.
ACKNOWLEDGEMENTS
The authors thank Barbara Gamb for unsurpassed literature service. Matthias Contzen (CVUA Stuttgart) is acknowledged for gyrB analysis. We are grateful to Konrad Sachse for critically reading the manuscript.
The Hessian State Laboratory is supported by the Hessian Ministry for the Environment, Climate Change, Agriculture and Consumer Protection (HMUKLV).
DECLARATION OF INTEREST
None.