Introduction
Wetlands are dynamic habitats that supply and store water for local and downstream ecosystems (Brandis et al. Reference Brandis, Mazumder, Gadd, Ji, Kingsford and Ramp2021), while serving as transition zones between terrestrial and aquatic ecosystems (Zedler and Kercher Reference Zedler and Kercher2005; Erwin Reference Erwin2009). Healthy wetlands with limited degradation provide ecosystem services, including water purification, carbon storage, and flood attenuation (Finlayson and Rea Reference Finlayson and Rea1999). Additionally, undisturbed wetlands generally support high biodiversity (Hu et al. Reference Hu, Niu, Chen, Li and Zhang2017; Wamiti et al. Reference Wamiti, Gichuki, Sigana, Njoroge, Morrison and Ndung’u2020). Due to land-use changes and other anthropogenic disturbances, many wetland systems are losing the ability to perform basic functions, resulting in biodiversity loss that mirrors global declines in species diversity (Sharma and Rawat Reference Sharma and Rawat2009; Sun et al. Reference Sun, Lin, Chen, Guo and Zeng2016).
The health and functionality of inland wetlands are major conservation concerns in the arid country of South Africa, with 88% of the country’s wetlands considered threatened and only 2% formally protected (Skowno et al. Reference Skowno, Poole, Raimondo, Sink, Deventer and Niekerk2019). Recognising this, the South African National Biodiversity Assessment (NBA) highlighted the urgent need to document wetland biodiversity, with an emphasis on high-elevation inland wetlands (Skowno et al. Reference Skowno, Poole, Raimondo, Sink, Deventer and Niekerk2019). Birds are amongst the most dominant vertebrate species in wetlands (Schummer et al. Reference Schummer, Palframan, McNaughton, Barney and Petrie2012) and can be used as bio-indicators of wetland health (Mistry et al. Reference Mistry, Berardi and Simpson2008). Furthermore, monitoring avian biodiversity is one of the main priorities of global nature conservation, due to what has been labelled the sixth mass extinction (Triviño et al. Reference Triviño, Kujala, Araújo and Cabeza2018; Wilson et al. Reference Wilson, Schuster, Rodewald, Bennett, Smith and La Sorte2019; Allen et al. Reference Allen, Burger and Lockwood2019).
South Africa is known for its high avian species diversity, with over 870 bird species, of which 68 are endemic or near-endemic. More than 130 of these species are globally threatened (Dowsett and Dowsett-Lemaire Reference Dowsett and Dowsett-Lemaire2001; Kopij Reference Kopij2015; Taylor and Peacock Reference Taylor and Peacock2018). Across South Africa populations of numerous species have decreased and distribution patterns have altered over the past two decades (Underhill and Brooks Reference Underhill and Brooks2014; Amar et al. Reference Amar, Cloete and Whittington2016). Wetland-dependent species such as the Eurasian Bittern Botaurus stellaris, Wattled Crane Grus carunculata, and White-winged Flufftail Sarothrura ayresi have suffered catastrophic population declines during the twentieth century, now ranking amongst the most endangered species in the region (Frommolt Reference Frommolt2017; Allen et al. Reference Allen, Burger and Lockwood2019). Such high-elevation wetland species are dependent on large, undisturbed wetland systems in the wetland–grassland mosaic that is characteristic of the eastern Great Escarpment of South Africa (Frommolt Reference Frommolt2017; Colyn et al. Reference Colyn, Campbell and Smit-Robinson2019).
South Africa’s Drakensberg mountains, part of the grassland biome, contrast sharply with those of the Global North, which tend to be dominated by coniferous forests that are frequently denuded due to human activities and climate change (Bian et al. Reference Bian, Li, Lei, Zhang and Nan2020; Shevtsova et al. Reference Shevtsova, Heim, Kruse, Schröder, Troeva and Pestryakova2020; Roebroek et al. Reference Roebroek, Melsen, van Dijke, Fan and Teuling2020). These forests are adapted to cold temperatures and have a relatively low diversity of plant species (Desyatkin et al. Reference Desyatkin, Okoneshnikova, Ivanova, Nikolaeva, Filippov and Desyatkin2022). In contrast, the vegetation of mountains in the Global South tends to be more diverse, with a greater variety of plant species and ecosystems (Chatanga et al. Reference Chatanga, Kotze, Janks and Sieben2019). Some of these high-altitude grasslands are centres of plant endemism classified as biodiversity hotspots, yet detailed descriptions of biodiversity in high-altitude wetlands within this biome remain inadequate (Brand et al. Reference Brand, Scott-Shaw and O’Connor2019; Carbutt Reference Carbutt2019; Ayambire et al. Reference Ayambire, Pittman and Olive2021). Large-scale land-use transformation through agriculture, mining, plantation forestry, alien invasive plant species, replacement of indigenous antelope with livestock, and inadequate fire management along the Great Escarpment have resulted in major wetland habitat loss, as well as intensified disturbances during the breeding season of most bird species (Zuckerberg et al. Reference Zuckerberg, Ribic and McCauley2018; Araya-López et al. Reference Araya-López, Lopatin, Fassnacht and Hernández2018; Allen et al. Reference Allen, Burger and Lockwood2019; Thomas et al. Reference Thomas, Speldewinde, Roberts, Burbidge and Comer2020). Coupled with climate change – best represented through intensifying El Niño-Southern Oscillation (ENSO) extremes – this “global change” cocktail could be fatal to remaining bird populations (Simmons et al. Reference Simmons, Barnard, Dean, Midgley, Thuiller and Hughes2004). The effects of climate change, habitat loss, and other anthropogenic activities can be seen in the differences found when comparing the Southern African Bird Atlas Project 1 (SABAP1) (1987–1991) to the second version of the same project (SABAP2) (2007–present) (Amar et al. Reference Amar, Cloete and Whittington2016; Hugo and Altwegg Reference Hugo and Altwegg2017; Little and Navarro Reference Little and Navarro2019). These differences include range expansions, contractions, distribution shifts, and altered migratory patterns (Hugo and Altwegg Reference Hugo and Altwegg2017; Underhill and Brooks Reference Underhill and Brooks2014). Increasing anthropogenic disturbances may drive more species to healthy wetlands, since these habitats provide refuge for disturbance-sensitive species (Belle et al. Reference Belle, Collins and Jordaan2018). Surveying avian biodiversity in healthy wetlands and disturbed systems is thus paramount to determine empirically changes in community composition and species distribution patterns (Finlayson and Rea Reference Finlayson and Rea1999; Colyn et al. Reference Colyn, Campbell and Smit-Robinson2017; Araya-López et al. Reference Araya-López, Lopatin, Fassnacht and Hernández2018).
Monitoring bird species’ absence/presence and collecting statistically defensible data on population trends is a challenging task, especially in remote mountainous areas (Frommolt Reference Frommolt2017; Araya-López et al. Reference Araya-López, Lopatin, Fassnacht and Hernández2018). Traditional methods, such as timed species counts, transect counts, and bird watching (Pomeroy and Dranzoa Reference Pomeroy and Dranzoa1997) are not always easy to implement in wetlands, as they are invasive, time-consuming, and influenced by observer bias (Klingbeil and Willig Reference Klingbeil and Willig2015). Cryptic species are also difficult to detect using direct observational techniques (La Sorte and Jetz Reference La Sorte and Jetz2010; Vu and Tran Reference Vu and Tran2020). However, passive monitoring techniques are less invasive and have become much more readily available to record the presence of species in any given area (Calvo and Blake Reference Calvo and Blake1998; Marsden et al. Reference Marsden, Whiffin and Galetti2001; Gasc et al. Reference Gasc, Sueur, Jiguet, Devictor, Grandcolas and Burrow2013). These methods generally include motion-triggered camera traps (Colyn et al. Reference Colyn, Campbell and Smit-Robinson2020) and passive acoustic monitoring (PAM) (Colyn et al. Reference Colyn, Campbell and Smit-Robinson2017).
PAM is one of the newer techniques that allows for recording acoustically active species with limited disturbance to a species’ activity and breeding behaviour (Aide et al. Reference Aide, Corrada-Bravo, Campos-Cerqueira, Milan, Vega and Alvarez2013; Ospina et al. Reference Ospina, Villanueva-Rivera, Corrada-Bravo and Aide2013; Alvarez-Berríos et al. Reference Alvarez-Berríos, Campos-Cerqueira, Hernández-Serna, Amanda Delgado, Román-Dañobeytia and Aide2016; Browning et al. Reference Browning, Gib, Glover-Kapfer and Jones2017). While PAM has not been intensively used to study bird communities in the wetlands of South Africa, it has been implemented in other ecosystems around the world and proven to yield rich data sets over a relatively short period compared with traditional methods (Stowell et al. Reference Stowell, Wood, Pamuła, Stylianou and Glotin2019). For example, PAM has been used to study the migration patterns (Koleček et al. Reference Koleček, Adamík and Reif2020), vocal activity (Frommolt Reference Frommolt2017), population abundance, and basic presence/absence of several bird species (Pamula et al. Reference Pamula, Pocha and Klaczynski2019; Duchac et al. Reference Duchac, Lesmeister, Dugger, Ruff and Davis2020; Bota et al. Reference Bota, Traba, Sardà-Palomera, Giralt and Pérez-Granados2020). This technique has also proved effective in monitoring ecosystem health using bio-indicator species (Stowell et al. Reference Stowell, Wood, Pamuła, Stylianou and Glotin2019) and species richness/diversity indices (Klingbeil and Willig Reference Klingbeil and Willig2015).
The aim of this study was to respond to the NBA call to document the biodiversity of South African inland wetlands (Skowno et al. Reference Skowno, Poole, Raimondo, Sink, Deventer and Niekerk2019) by surveying the avian diversity of the Kleinspruit Wetland, a previously unsurveyed 200-ha, healthy, peat-based wetland in the Golden Gate Highlands National Park (GGHNP) in Free State Province in the eastern Great Escarpment region. We specifically used PAM in this relatively isolated wetland system to monitor non-invasively the area continuously over two wet and dry seasons potentially to detect elusive species that direct observation is unlikely to discover.
Methods
Study site
We conducted the study at the GGHNP (28°27’S 28°44’E) in the mountainous eastern Free State Province of South Africa (Figure 1). This region experiences dynamic climatic conditions including heavy rainfall events and thunderstorms during the wet season (austral summer) and harsh cold conditions during the dry season (austral winter) (Koleček et al. Reference Koleček, Adamík and Reif2020). The GGHNP covers an area of 340 km2 within the fire-driven grassland biome (Mucina and Rutherford Reference Mucina and Rutherford2006). It forms part of the South African National Parks (SANParks) system and is a Key Biodiversity Area, as it supports rare and endemic grassland, wetland, and mountain bird species.
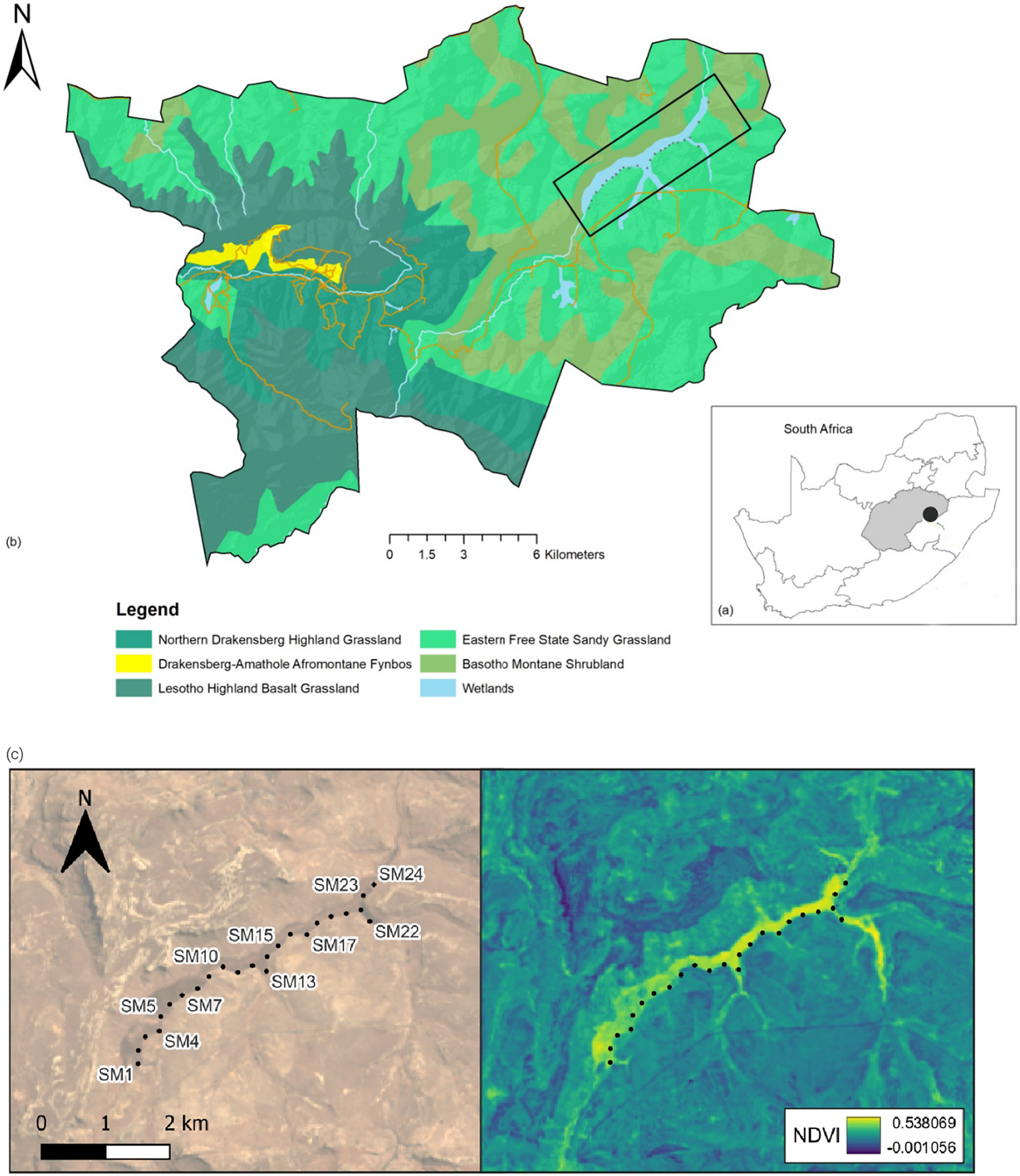
Figure 1. (a, b) Location of the Kleinspruit Wetland in South Africa and vegetation types of the wetland peripherals (Mucina and Rutherford Reference Mucina and Rutherford2006). (c) The Normalized Difference Vegetation Index (NDVI) across the sampling period ranging from -0.001056 to 0.538068. The black dots represent the location of the Song Meters.
We selected the Kleinspruit Wetland, a relatively undisturbed wetland that is part of the Wilge catchment within the GGHNP. The 200-ha Kleinspruit Wetland is roughly linear, 6 km in length, ranging from 100 m to 300 m in width. The wetland is dominated by Common Reeds (Phragmites australis), while in the upper reaches and side tributaries Carex-sedge beds and occasional bulrushes (Typha capensis) are more abundant. Wetland margins are grassy, and there is an abrupt transition from the wetland to the adjacent dryland grasslands, i.e. eastern Free State sandy grassland and Basotho montane shrubland (Mucina and Rutherford Reference Mucina and Rutherford2006). There is a steep hill on the northern side and flatter, rocky terrain on the southern side. Situated in a grassland, the wetland is prone to burning (VRC observation). Numerous paths maintained by large mammals such as Black Wildebeest (Connochaetes gnou) traverse the wetland. Water levels exhibit strong seasonality, with low dry season levels and high wet season levels, with an estimated difference of 1 m between seasons.
Passive acoustic equipment
We initially deployed four acoustic devices, Song Meter 4 (Wildlife Acoustics, Maynard, MA, USA) in a rolling grid layout, with 250 m (equivalent to 1.7 times the range of the recorders) between successive placements along the wetland’s south-western margin (Figure 1c). Every two weeks we replaced batteries, downloaded data, and redeployed equipment at the next four locations in the grid. We attached each Song Meter to a 2.5 m metal pole sunk at the edge of the wetland to protect equipment from potential flooding. The Song Meters recorded from 04h00 to 06h00 Central African Time on both channels at a sampling rate of 44,000 Hz in 16-bit mode, during peak dawn bird vocal activity (Pérez-Granados et al. Reference Pérez-Granados, Bota, Giralt and Traba2018a, Reference Pérez-Granados, Schuchmann and Marques2021). We collected data from June 2019 to December 2020, losing data and equipment between 21 October 2019 and 11 November 2019, when a fire swept through the study site in an El Niño summer. In November 2019, the Song Meters were replaced with Audio Moths (AudioMoth v1.2.0, Open Acoustic Devices, LabMaker, Berlin, Germany), recording on similar settings. The COVID-19 pandemic lockdown restrictions did not allow access to the wetland from March 2020 to June 2020, which resulted in a data gap for this period.
Acoustic analysis
We analysed the acoustic data using Kaleidoscope Pro software from Wildlife Acoustics. Kaleidoscope Pro converts all recorded vocalisations to .wav format and creates clusters that are easy to view as spectrograms. The provision of spectrograms allows fast identification of species based on their calls by creating clusters of similar calls that are matched to training calls, which we obtained from Xeno-Canto (https://www.xeno-canto.org/). According to the SABAP2 accessed in 2020, the GGHNP has 220 different bird species identified over the past 15 years (2007–2021), based on both visual identification and bird calls/songs. These formed the basis of the initial set of training calls for the classifier. We identified the vocalisations in unnamed clusters through expert consultation.
We set Kaleidoscope to the non-bat analysis mode, frequency range between 250 Hz and 10,000 Hz, call length detection between 2 s and 7.5 s, inter-syllable gap of 0.2 s, and Fast Fourier Transformation (FFT) at 5.33 ms (128 @ 0–12 kHz, 256 @ 13–24 kHz, 512 @ 25–48 kHz, 1,024 @ 49–96 kHz) to accommodate a range of species with low and high frequency calls. The maximum distance from the cluster centre was 0.5 and maximum clusters’ parameter at 1,000 (Pérez-Granados et al. Reference Pérez-Granados, Osiejuk and López-Iborra2018b).
We manually verified all automatically detected species by visual and auditory inspection and compared spectrograms from the training calls with those generated from the acoustic data set. Bird experts (Steven Segang, Bonginkosi Ndaba and David Weaver) listened to the automatically detected species for confirmation. We sent the clusters that these experts could not identify to BirdLife South Africa for further identification. We used the 2015 Eskom Red Data Book of Birds of South Africa, Lesotho and Swaziland (Taylor et al. Reference Taylor, Peacock and Wanless2015) and the International Union for Conservation of Nature (IUCN) to assign a regional conservation to each identified bird species.
Results
Through PAM, we detected 79 bird species at the Kleinspruit Wetland, constituting 35.9% of all GGHNP bird species recorded by SABAP2. Of the 79 detected species, three species are regionally listed as “Vulnerable” and one species as “Endangered” (IUCN 2020). Of the 220 species recorded in the GGHNP by SABAP2, 72 are considered obligate wetland species. However, we identified only 49 of these 72 obligate wetland species (68.1%) in the Kleinspruit Wetland. Of these species-presence detections only one, Grey Crowned Crane Balearica regulorum, is listed as “Endangered” (Figure 2).
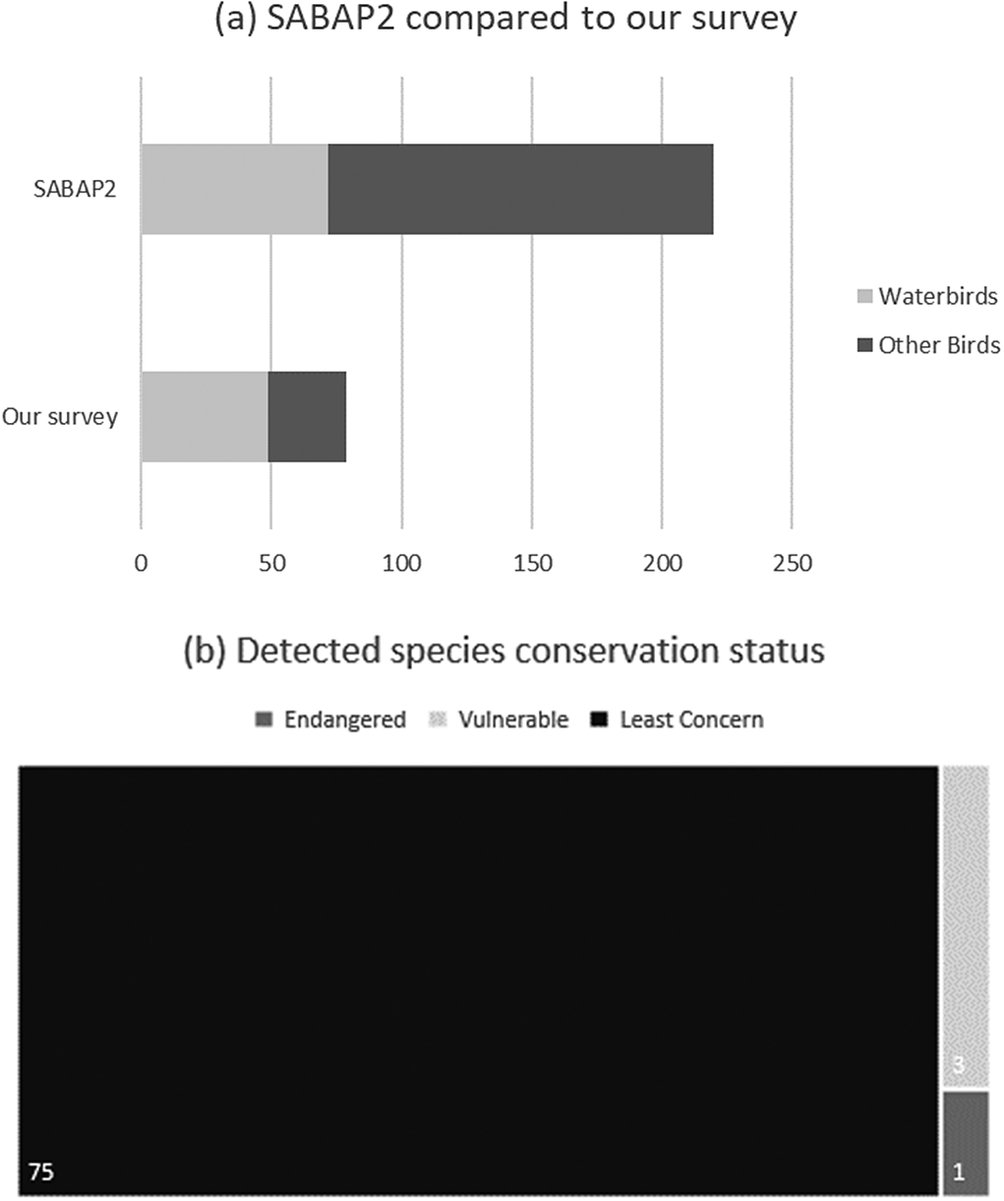
Figure 2. (a). Comparison between the acoustic survey’s results and the Southern African Bird Atlas Project 2 (SABAP2), which used traditional survey techniques to document birdlife in the Golden Gate Highlands National Park (GGHNP). (b) The global International Union for Conservation of Nature (IUCN) conservation status of birds detected at the Kleinspruit Wetland in the GGHNP in our survey.
In addition, we detected 25 species outside their expected range. SABAP2 has noted most of these species within 100 km of the GGHNP, although not within the GGHNP, suggesting that they were not entirely unexpected in this locality (Figure 2). We noted three species amongst these 25 detections that have never been recorded within a 100-km radius of the GGHNP; three of these species are of conservation concern (Table 1).
Table 1. Bird species identified at the Kleinspruit Wetland that are not part of the historical Golden Gate Highlands National Park bird list. The regional IUCN status of detected species is “Least Concern”, except where indicated as * “Vulnerable” or ** “Endangered”. Bold font highlights the species detected outside their known ranges.
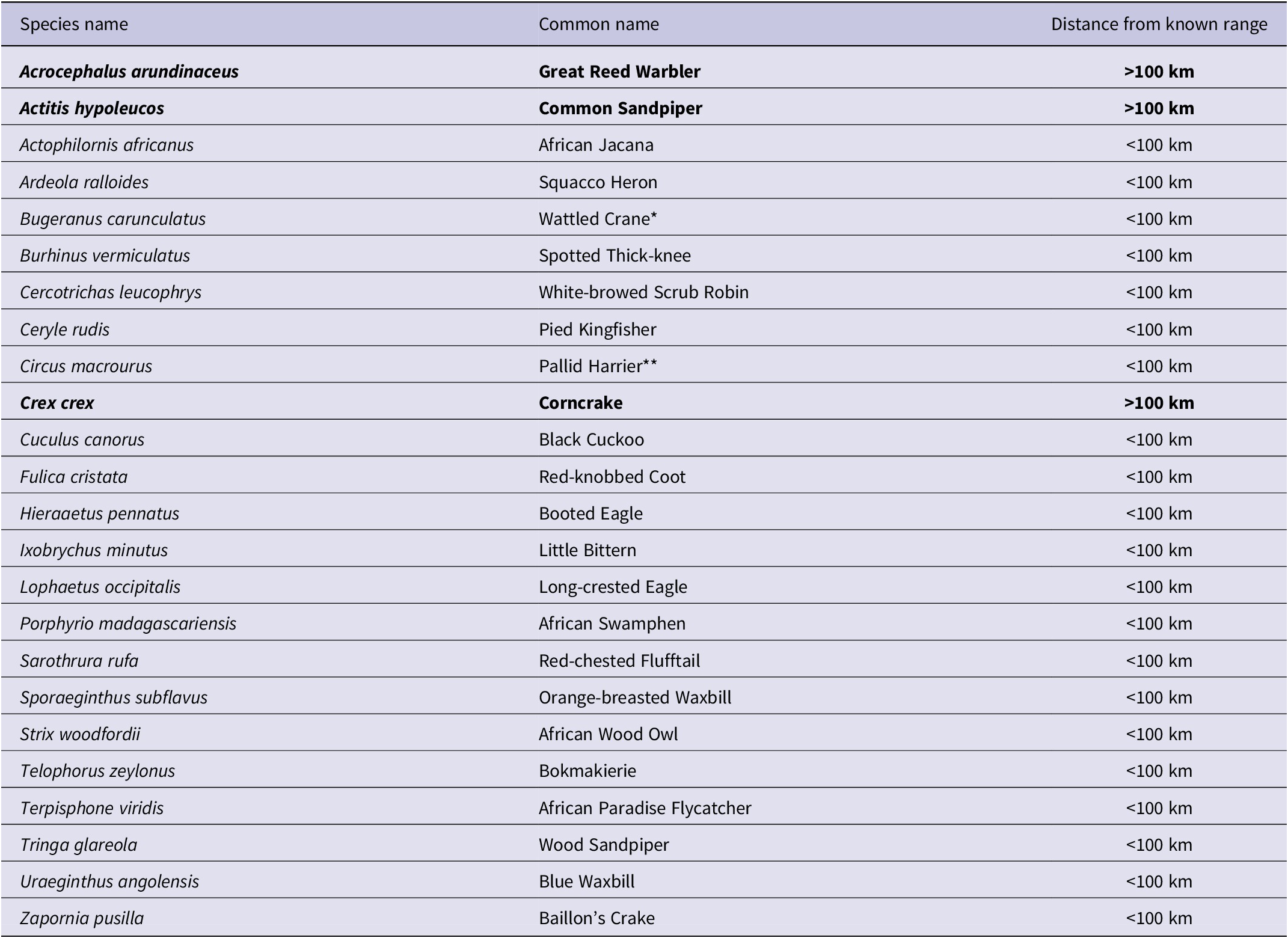
Discussion
The aim of this study was to respond to the NBA call to document the biodiversity of South African inland wetlands (Skowno et al. Reference Skowno, Poole, Raimondo, Sink, Deventer and Niekerk2019) by surveying the avian diversity of the Kleinspruit Wetland, a previously unsurveyed 200 ha, healthy, peat-based wetland in the eastern Great Escarpment region. Using PAM, we were able to add valuable novel avifaunal data to the current species records for the GGHNP, complementing the data in SABAP2 that is based on direct human observations in different pentads of the park, with more data collected from easily accessible areas such as camp sites and road sides. Through this study, we have shed a light on species distribution in a poorly known, large wetland system that is difficult to access in an area of the park not frequented by tourists, and enhanced the overall conservation value of this protected area.
Among the new species recorded were secretive species such as the African Swamphen Porphyrio madagascariensis (which is usually a locally common resident if there is thick cover, even in degraded wetlands in urban areas), and Great Reed Warbler Acrocephalus arundinaceus (recorded in the GGHNP in SABAP2, but only up to 2.5% of SABAP2 record for the two park pentads); both are common wetland species. Baillon’s Crake Zapornia pusilla is generally uncommon (Lee and Hammer Reference Lee and Hammer2022; Brooks et al. Reference Brooks, Rose, Altwegg, Lee, Nel and Ottosson2022), and its presence is a potential indicator of the value of this wetland for such secretive residents; it was recorded in nearby Bethlehem and Memel in SABAP2, so our record is a valuable additional record for the eastern Free State.
Surprise new records included species more associated with forest (African Wood Owl Strix woodfordii). However, the proximity of an Afrotemperate forest patch only 3.6 km due west of the study site may help to explain the presence of an African Wood Owl. This is a common species in the scattered temperate forests of the eastern Great Escarpment (Gunawan et al. Reference Gunawan, Hidayat, Cenggoro and Pardamean2021; Mikkola et al. Reference Mikkola, Mikkola and Mikkola2022), although not well represented in SABAP2 records for the Free State–KwaZulu-Natal border. The Yellow-Bellied Greenbul is almost certainly a vagrant, but there have been small irruptions of the species recently into the Free State, with one record for the Welkom area in SABAP2, so its presence is not improbable.
For 14 species, the novel records in GGHNP likely represent prior under-sampling, rather than true range expansions, as they have been recorded in surrounding localities such as Phuthaditjhaba, Bethlehem, Harrismith, and Clarens. Previously, species such as Common Sandpiper ( Actitis hypoleucos) , Corncrake ( Crex crex ), Great Reed Warbler, and Pallid Harrier (Circus macrourus) were thought of as just incidental records during the summer migration period, but according to our data these species are likely regular visitors or seasonal residents in the austral summer months (Lee and Hammer Reference Lee and Hammer2022). It is worth noting that two species with strongholds in the eastern Free State, i.e. Eurasian Bittern and White-winged Flufftail (Colyn et al. Reference Colyn, Campbell and Smit-Robinson2020), were not detected in the Kleinspruit Wetland during this study, despite being expected in this habitat.
Although this study was primarily focused on wetland species, the linear nature of the wetland coupled with the broad radius of detectability by the Song Meters resulted in a large component of the data set being non-wetland birds, i.e. those occurring on the adjacent hill-slope and dryland grasslands, or facultative species (those using both the wetland and the adjacent drylands). While this may seem distracting from the wetland focus, it does in fact provide cross-over perspective on avifauna in a grassland–wetland mosaic, which is typical of the eastern Great Escarpment, where wetlands of various dimensions and shapes are embedded in a grassland landscape (Mucina snd Rutherford Reference Mucina and Rutherford2006). Field observations at the wetland by TM and VRC suggest that there is a closely connected wetland–dryland ecology at the site, with dryland birds using the wetland, and vice versa. The most obvious cross-over is aerial feeders such as Swifts and Swallows, but also Chats (which were noted to forage along the wetland edges), Widowbirds (that breed in the wetland but forage deep into the adjacent grasslands), parasitic species (e.g. the Diederik Cuckoo Chrysococcyx caprius parasitising weavers that breed in the wetland), and birds of prey targeting wetland species.
It is worth noting that, surprisingly, the audio data set did not include some abundant, vocal species that have been noted visually, such as Southern Red Bishop Euplectes orix. Reasons for this are unclear, but it does raise the question as to which other species have not been recorded through PAM and suggests that a true diversity assessment still needs direct observations in the field to corroborate and augment PAM data (Towsey et al. Reference Towsey, Wimmer, Williamson and Roe2014; Fujioka et al. Reference Fujioka, Soldevilla, Read and Halpin2014; Gibb et al. Reference Gibb, Browning, Glover-Kapfer and Jones2019).
Our results underscore the importance of protecting this high-elevation wetland as a safe habitat for at-risk species utilising grassland–wetland mosaics. We confirmed the presence of species of conservation concern, the Grey Crowned Crane, which forages in the surrounding grasslands in the GGHNP and farmlands beyond (Wamiti et al. Reference Wamiti, Gichuki, Sigana, Njoroge, Morrison and Ndung’u2020; Amulike et al. Reference Amulike, Fuller, Houlihan and Griffin2020), and likely relies on the Kleinspruit Wetland for breeding. We recorded Wattled Crane for the first time in the GGHNP, at the wetland; it is amongst the most “Vulnerable” eastern Escarpment species. The unpredictable and unmanaged burns in this eastern part of the park in the winter–spring months have a major impact on the wetland; these times are critical for Wattled Crane breeding success, and fire management around the wetland should become a park priority as soon as possible (Wamiti et al. Reference Wamiti, Gichuki, Sigana, Njoroge, Morrison and Ndung’u2020). Increasing El Niño intensity also leaves the wetland vulnerable to fire for a much longer period (May–November/December, compared with the usual May–September/October fire season), potentially filtering out the White-winged Flufftail, which is believed to breed in early summer (Maphisa et al. Reference Maphisa, Smit-Robinson, Underhill and Altwegg2016; Prowse et al. Reference Prowse, Collard, Blackwood, O’Connor, Delean and Barnes2017; Smith et al. Reference Smith, Smith and Downs2017; Matteson et al. Reference Matteson, Jackson, Batzer, Wilde and Jeffers2020; Brandis et al. Reference Brandis, Mazumder, Gadd, Ji, Kingsford and Ramp2021).
Conclusions
This study has confirmed PAM as useful for augmenting direct records for remote habitats such as wetlands, and for detecting species that would be difficult to identify through direct observations, whether those are visual or auditory. The use of PAM is suitable for monitoring remote areas that have been understudied; however, PAM on its own is not sufficient. The technique requires human verification, especially with data analysis. This is not unexpected, as most machine-learning approaches require a certain degree of human input. We advise future studies to incorporate both machine learning and human verification when using PAM, especially when monitoring rare and elusive species.
Acknowledgements
Funding was provided by the Afromontane Research Unit and National Research Foundation (Integrated Biodiversity Information Programme grant number 128337). Wildlife Acoustics generously sponsored us with Song Meters and Kaleidoscope software. We thank Hanneline Smit-Robinson (Head of Conservation, BirdLife South Africa) for supporting the ARU–BirdLife South Africa partnership. We thank Robyn Colyn and Dave Weaver for assisting with identifications of unknown birdsong, and valuable logistical advice. We thank South African National Parks and the Golden Gate Highlands National Park for permits and permission to undertake the project. We are deeply grateful to Anthony Mapaura for extensive fieldwork, data collection, and processing support. Author’s contributions: T. M.: methodology, data collection, data analysis, drafting the paper, editing, and reviewing; A. le R.: conceptualisation of study, funding, data validation, and editing; N. le M.: data collection, methodology, editing, and reviewing; V. R. C.: conceptualisation of study, funding, data validation, editing, and reviewing; S.-L. S.: project management, funding, data collection, methodology, editing, and reviewing; K. J. L.: data processing, reviewing, and editing. Data used in this paper is readily available on request and all data from the Southern African Bird Atlas Project 2 is available online (SABAP 2).