With advances in neonatal intensive care, the survival of very preterm (gestational age (GA) < 32 weeks) and/or very low birth weight (VLBW; birth weight < 1500 g) children has improved considerably. However, these children are at risk for poor motor, cognitive and behavioural outcomes later in childhood( Reference de Kieviet, Piek and Aarnoudse-Moens 1 , Reference Bhutta, Cleves and Casey 2 ) due to a variety of risk factors associated with preterm birth. Risk factors include neonatal infections and inflammatory responses, both contributing to early brain injury( Reference Adams-Chapman and Stoll 3 ).
Over the past decade, the potential protective effects of supplementation of the amino acid glutamine in very preterm and/or VLBW children have been extensively studied, including the modulation of inflammatory response and stimulation of immunity( Reference Neu and Li 4 ). Experimental studies have shown that glutamine plays an important role in maintaining the functional integrity of the gut( Reference Khan, Iiboshi and Cui 5 , Reference Rhoads, Argenzio and Chen 6 ), which in turn leads to decreased bacterial translocation and systemic spread of bacteria( Reference Fox, Kripke and De Paula 7 – Reference Souba, Klimberg and Hautamaki 9 ), and consequently may lead to decreased infectious morbidity. Indeed, a few studies found that glutamine-enriched enteral nutrition between day 3 and day 30 of life decreased the number of serious neonatal infections in very preterm and/or VLBW children( Reference Neu, Roig and Meetze 10 , Reference van den Berg, van Elburg and Westerbeek 11 ), although other studies failed to replicate the beneficial effects of glutamine( Reference Tubman, Thompson and McGuire 12 ). A lower number of serious neonatal infections may potentially be beneficial for long-term motor, cognitive and behavioural outcomes in very preterm and/or VLBW children, as evidence from several studies emphasises the negative effects of neonatal infections on long-term neurodevelopment( Reference Kermorvant-Duchemin, Laborie and Rabilloud 13 – Reference Volpe 16 ). One study found no beneficial or adverse effects of enteral glutamine supplementation on mental or motor outcomes at 2 years of age as measured by the Bayley Scales of Infant Development, second edition( Reference van Zwol, van den Berg and Huisman 17 ), while another study found that long-term enteral glutamine supplementation may lead to significant improvements in growth measures( Reference Korkmaz, Yurdakok and Yigit 18 ). However, until now it remains unclear whether a lower incidence of neonatal infections and improved growth in very preterm and/or VLBW children following glutamine supplementation may be beneficial for long-term motor, cognitive and behavioural development.
The aim of the present study was to determine the long-term effects of enteral glutamine, supplemented in a randomised placebo-controlled trial between day 3 and day 30 of life, on the cognitive, motor and behavioural outcomes of very preterm and/or VLBW children at school age.
Methods
The initial sample for the present study consisted of 102 very preterm ( < 32 weeks) and/or VLBW ( < 1500 g) infants participating in a randomised placebo-controlled trial on glutamine supplementation. In the present study, infants received enteral glutamine supplementation (0·3 g/kg per d) or an isonitrogenous placebo supplementation (alanine) between day 3 and day 30 of life. All very preterm and/or VLBW infants admitted to the level III neonatal intensive care unit (NICU) of the VU University Medical Center Amsterdam between September 2001 and July 2003 were eligible for inclusion. Descriptions of baseline characteristics and techniques used to determine incidence of serious neonatal infections, intraventricular haemorrhage, periventricular leucomalacia and bronchopulmonary dysplasia have been previously reported( Reference van den Berg, van Elburg and Westerbeek 11 ). Of the 102 infants included in the study, eighty-nine infants were alive at 1 year of age, and seventy-four were still participating at 6 years of age.
At 7–8 years of age, parents of all seventy-four children were contacted and invited to participate in the present study, of which sixty-eight (92 %) showed up, and sixty-four (94 %) successfully completed all tasks at the mean age of 7·5 (sd 0·4) years. The remaining four children had serious motor (n 2), hearing (n 1) or vision (n 1) difficulties, crucially interfering with task execution. The final sample consisted of thirty children that had received glutamine (glutamine group) and thirty-four children that had received placebo (control group). From the original dataset, the presence of serious neonatal infections and other clinical complications were extracted. Serious neonatal infections included sepsis, meningitis, pyelonephritis, pneumonia and arthritis, accompanied by positive microbial cultures of blood, cerebrospinal fluid, urine, tracheal aspirates and synovial fluid, respectively, as previously described in more detail( Reference van den Berg, van Elburg and Westerbeek 11 ). Socio-economic status (SES) was determined by classifying the highest level of education in a household with a number ranging from 0 to 4. A higher number indicated a higher level of education and a corresponding higher SES. Characteristics of both groups are shown in Table 1.
Table 1 Sample characteristics (Mean values and standard deviations; numbers of participants and percentages; medians and ranges)
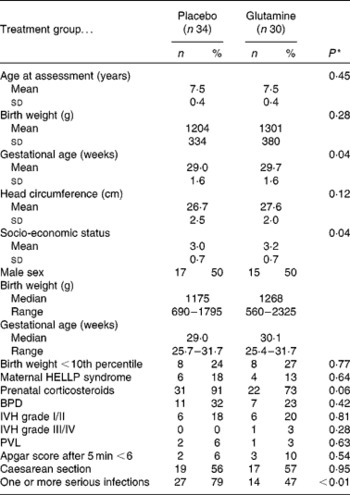
HELLP, haemolysis elevated liver enzymes and low platelets; BPD, bronchopulmonary dysplasia; IVH, intraventricular haemorrhage; PVL, periventricular leucomalacia.
* χ2 and t tests.
Procedure
The present study was conducted according to the guidelines laid down in the Declaration of Helsinki and all procedures involving human subjects were approved by the medical ethical committee of the VU University Medical Center. Written informed consent was obtained from all subjects. Cognitive and motor assessment took place at the VU University Amsterdam by qualified and trained testers using a completely standardised instruction protocol. Both parents and each child's teacher were asked to fill in questionnaires addressing behavioural problems at home and at school, respectively. Parents filled in the questionnaires in the presence of an interviewer.
Cognitive and motor measures
The main aspects of cognitive and motor functioning were assessed, including intellectual development, working memory, motor development, aspects of attentional functioning and processing speed. Intellectual development was measured by a short form of the Wechsler Intelligence Scale for Children-III (WISC-III( Reference Wechsler 19 )), including the subtests Vocabulary and Block Design. Both subtests correlate strongly (r >0·90) with full-scale intelligence quotient( Reference Groth-Marnat 20 ). Scores on this test were normalised with a mean of 100 (sd 15). Two aspects of working memory abilities were assessed: verbal working memory and visual working memory. Verbal working memory abilities were measured using the Digit Span subtest of the WISC-III. In this subtest, children had to verbally reproduce dictated series of digits increasing in length, both in a forward and a backward condition. Maximal spans of reproduced digits for both the forward as well as the backward condition were included as dependent variables in the analysis. Visual working memory abilities were measured using an adapted version of a task developed by Nutley et al. ( Reference Nutley, Söderqvist and Bryde 21 ). In this task, children had to reproduce sequences of circles appearing in a 4 × 4 grid on a touch screen. Difficulty level was increased during the course of the task by increasing the span and by manipulating the position of the stimuli. Two trials were administrated for each difficulty level, and the task was terminated when the child failed to accomplish both trials at a certain difficulty. Maximal difficulty levels with forward reproduction and backward reproduction were included as dependent variables in the analysis. Motor development was assessed using the Movement Assessment Battery for Children (MABC)( Reference Henderson and Sugden 22 ), and outcomes on the scales Manual Dexterity, Balance Skills and Ball Skills were included in the analysis. Scores on the scales of the MABC were normalised using T-scores with a mean of 50 (sd 10). Orienting, executive and alerting attention was assessed using an adapted version of the Attention Network Test( Reference Fan, McCandliss and Fossella 23 ) suitable for use with young children. In this task, children had to respond as accurately and quickly as possible to the appearance of a target on the left side or the right side of the screen by pressing a button corresponding to the location at which the target appeared. There were four types of trials. Neutral trials contained a neutral cue in the middle of the screen which preceded the target. Orienting trials contained a directional cue in the middle of the screen pointing to the position of the target which subsequently followed. Executive trials contained directional cues incongruent with the position of the target. Alerting trials contained no cue at all and the target was presented instantaneously. The four trial types were randomly presented in four blocks of forty-eight trials. Measures of orienting, executive and alerting attention were obtained by subtracting the mean response time on the orienting, executive and alerting trials from the mean response time on neutral trials, respectively. In this way, measures of orienting, alerting and executive attention are controlled for differences in information-processing capacities between children. The gain in response time in an orienting trial as compared with a neutral trial was used as a measure of the ability to aim attention (orienting attention). More gain in response time corresponds to better orienting attention. The loss in response time in an executive trial as compared with a neutral trial was used as a measure of the ability to actively ignore irrelevant information (executive attention). Less loss in response time corresponds to better executive attention. The loss in response time in an alerting trial as compared with a neutral trial was used as a measure of alertness (alerting attention). Less loss in response time corresponds to better alerting attention. Finally, the combined effects of cognitive and motor speed were assessed by mean response time in neutral trials and included as a measure of overall processing speed in the analysis.
Behavioural measures
Parents rated their children's behavioural difficulties using the Child Behaviour Checklist (CBCL)( Reference Verhulst, van der Ende and Koot 24 ) and the parent-rated Disruptive Behaviour Disorders questionnaire (PDBD)( Reference Oosterlaan, Scheres and Antrop 25 ). Teachers rated their pupil's behavioural difficulties using the Teacher's Report Form (TRF)( Reference Verhulst, van der Ende and Koot 26 ) and teacher version of the Disruptive Behaviour Disorders questionnaire (TDBD)( Reference Oosterlaan, Scheres and Antrop 25 ). The CBCL and TRF encompass three broad-band scales assessing total, externalising and internalising behaviour problems, and the PDBD and TDBD comprise two scales addressing inattention and hyperactivity problems. Scores on all scales were depicted as normalised T-scores with a mean of 50 (sd 10); higher scores indicated greater severity of problems. All questionnaires are widely used and have excellent psychometric properties.
Statistical analyses
All analyses were performed using SPSS 17.0 (SPSS, Inc.), and raw continuous data were successfully standardised and normalised by applying a Van der Waerden transformation. Pearson and point biserial correlations were used to explore the effects of serious neonatal infections, SES and GA on cognitive, motor and behavioural outcomes at school age. To study the effects of glutamine supplementation, univariate ANOVA with intervention as a between-subject factor and SES and GA as covariates was conducted on the dependent measures of the cognitive and motor tasks and parent- and teacher-rated questionnaires. To adjust for possible beneficial indirect effects of glutamine on the outcome measures by decreasing the number of serious neonatal infections, the analysis was repeated with serious neonatal infections as a second between-subjects factor. Standardised group differences were quantified in terms of effect sizes (Cohen's d)( Reference Cohen 27 ), which is an effect size defined by the difference between two group means divided by the pooled sd, enabling the opportunity to indicate the size of an effect independent of group size. Cohen's d guidelines were followed to indicate the strength of the group differences, with values of 0·20, 0·50 and 0·80 referring to small, medium and large effects, respectively( Reference Cohen 27 ). To minimise the possibility of a type 2 error, which may erroneously lead to the conclusion that no detrimental or beneficial effects of glutamine-enriched feeding exist on long-term outcomes, α was not corrected for multiple comparisons and set at 0·05.
Results
Sample characteristics
Clinical characteristics of the glutamine and placebo groups are shown in Table 1. The two groups did not differ on the majority of clinical characteristics, indicating that there were no differences in illness severity between the groups. However, SES and GA were higher for the glutamine group as compared with the placebo group. In addition, as was found in a previous study( Reference van den Berg, van Elburg and Westerbeek 11 ), the rate of serious neonatal infections was lower in the glutamine group than in the placebo group (P = 0·006).
Correlational analyses
Higher SES was associated with higher full-scale intelligence quotient scores (r 0·26; P = 0·002), and a shorter GA was associated with higher ratings on the TDBD Hyperactivity scale (r − 0·34; P = 0·006). Furthermore, the presence of serious neonatal infections was positively associated with multiple scales of parent- and teacher-rated questionnaires, including TRF Total Problems (r 0·25; P = 0·04), TRF Externalising (r 0·25; P = 0·04), PDBD Inattention (r 0·25; P = 0·04), TDBD Inattention (r 0·27; P = 0·03) and TDBD Hyperactivity (r 0·27; P = 0·03), indicating that the presence of infections is associated with higher ratings of behavioural problems and symptoms of attention deficit hyperactivity disorder in particular.
Cognitive, motor and behavioural outcomes
Cognitive, motor and behavioural outcomes are shown in Table 2. After adjusting for SES, GA, and including the presence of serious neonatal infections as a second between-subjects factor, the glutamine group showed poorer ball skills (P = 0·002; Cohen's d = 0·67), indicating that ball skills were more impaired in the glutamine group as compared with the placebo group. Furthermore, the glutamine group showed poorer executive attention as compared with the placebo group, although this effect was of marginal significance (P = 0·06; d = 0·49). When only adjusting for SES and GA, poorer ball skills for the glutamine group remained significant (P = 0·005), indicating that a reduced presence of serious neonatal infections following glutamine supplementation could not compensate for the differences in ball skills between the glutamine and placebo groups. No significant differences were found between the glutamine and placebo groups for any of the remaining cognitive and motor measures.
Table 2 Cognitive, motor and behavioural functioning for the placebo and glutamine groups (Mean values and standard deviations)
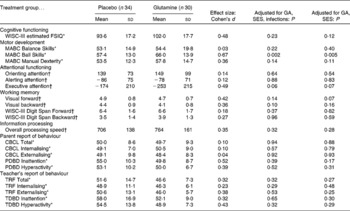
GA, gestational age; SES, socio-economic status; WISC-III, Wechsler Intelligence Scales for Children, 3rd edition; FSIQ, full-scale intelligence quotient; MABC, Movement Assessment Battery for Children; CBCL, Child Behaviour Checklist; PDBD, parent-rated Disruptive Behaviour Disorders questionnaire; TRF, Teacher's Report Form; TDBD, teacher version of the Disruptive Behaviour Disorders questionnaire.
* Normalised T-scores. Higher scores indicate poorer performance.
† Raw scores.
Behavioural outcomes
Behavioural outcomes as measured by parent- and teacher-rated questionnaires did not differ significantly between the glutamine and placebo groups. Including serious neonatal infections as a second between-subjects factor did not change the results of the primary analysis.
Discussion
The majority of long-term cognitive, motor and behavioural outcome measures were not different in the glutamine and placebo groups. These findings demonstrated that glutamine supplementation between day 3 and day 30 of life did not have beneficial or detrimental effects on a wide range of cognitive and motor functions or on parent- and teacher-reported behavioural outcomes at school age. However, ball skills as measured by the MABC were found to be significantly poorer in the glutamine group as compared with the placebo group, suggesting the possibility that glutamine treatment in the first month after birth may have adverse effects on visuomotor development.
Many factors may influence developmental outcomes between birth and 8 years of age, thereby diluting the effects of enteral glutamine supplementation between day 3 and day 30 of life. The current cognitive, motor and behavioural measures were selected to maximise the ability to chart any long-term effects on outcomes at school age. Furthermore, long-term effects on cognitive, motor and behavioural development of enteral glutamine supplementation could be expected, as glutamine treatment reduced the incidence of serious neonatal infections known to affect brain development( Reference Adams-Chapman and Stoll 3 , Reference Stoll, Hansen and Adams-Chapman 15 ). Indeed, significant associations were present between the presence of serious neonatal infections and the selected behavioural outcomes, including inattention and hyperactivity, indicating that an increase in the presence of serious neonatal infections may be associated with poorer outcomes. Nonetheless, no major effects of enteral glutamine supplementation on long-term cognitive, motor and behavioural outcomes were present.
The unfavourable effect of glutamine supplementation on visuomotor development as measured with the Ball Skills scale of the MABC was an unexpected finding, as our previous work has shown no unfavourable short-term effects of enteral glutamine supplementation( Reference van Zwol, van den Berg and Huisman 17 ), and no direct detrimental effects of glutamine on the brain have been reported( Reference Garlick 28 ). However, derivates of glutamine, including glutamate and ammonia, have been associated with neurotoxicity( Reference Garlick 28 , Reference Suárez, Bodega and Fernández 29 ), and could underlie detrimental outcomes following glutamine treatment. In patients with hepatic encephalopathy, ammonia was shown to affect the metabolism and function of astrocytes, leading to astrocytic swelling which in turn leads to cerebral oedema. Glutamine is synthesised in excess from ammonia and glutamate by glutamine synthetase, an astrocyte enzyme. Recent data suggest that many aspects of ammonia toxicity in hepatic encephalopathy are mediated by glutamine( Reference Albrecht, Zielinska and Norenberg 30 ). Nevertheless, plasma concentrations of glutamine, glutamate and other amino acids were not different between the glutamine and placebo groups in the neonatal period in the present study sample( Reference van den Berg, van Elburg and Teerlink 31 ), and the highest median glutamate (68 μmol/l glutamine group; 70 μmol/l placebo group) or glutamine concentrations (555 μmol/l glutamine group; 515 μmol/l placebo group) were within normal reference ranges( Reference Oladipo, Weindel and Saunders 32 ). Furthermore, if adverse neurological effects as a consequence of neurotoxicity occurred, more widespread consequences on cognitive, motor and behavioural outcomes would have been expected, given that visuomotor skills concern interplay between perception, motor skills and timing, involving multiple brain systems. Hence, direct effects on the brain seem unlikely, and we speculate that the presence of differences in visuomotor skills between the glutamine and placebo groups is related to baseline differences in visuomotor abilities at the moment of randomisation.
Several studies in VLBW infants have investigated the effects of parenteral or enteral glutamine supplementation on morbidity, mortality and (growth) outcome in the neonatal period( Reference Neu, Roig and Meetze 10 , Reference Korkmaz, Yurdakok and Yigit 18 , Reference Bober-Olesińska and Kornacka 33 – Reference Vaughn, Thomas and Clark 36 ). No evidence of toxicity of glutamine supplementation was found in these clinical trials, but the results of efficacy on a limited number of outcomes have been mixed( Reference Tubman, Thompson and McGuire 12 ), possibly due to differences in supplementation methods, dose and definition of infections. As a consequence, the use of glutamine supplementation has not become routine. However, the effects of glutamine supplementation on the incidence of serious neonatal infections warrants further investigation, given that our findings indicate no clear evidence of long-term detrimental effects on motor, cognitive and behavioural development, underlining the relative safe use of glutamine supplementation in very preterm and/or VLBW children in future research or clinical practice.
The present study has some limitations that need to be taken into account when interpreting the findings. First, the power of analyses was somewhat limited due to the fact that not all children were still participating nearly 8 years after original enrolment in the present study. However, drop-out rates were low for this type of long-term follow-up, and drop-out was equally present in the glutamine and placebo groups. Furthermore, power was sufficient to detect any medium- to large-sized beneficial or detrimental effects of glutamine treatment, as calculated using G-power software( Reference Faul, Erdfelder and Lang 37 ). Second, to reduce the chance of a type 2 error that erroneously may lead to the conclusion that no detrimental or beneficial effects of glutamine-enriched feeding on long-term outcomes exist, no α correction for multiple comparisons was conducted. The flip side of the coin is that our finding of unfavourable effects on visuomotor abilities of glutamine intervention might result from an unadjusted α level in our statistical tests.
In summary, with one single exception (visuomotor abilities), no medium- or large-sized beneficial or detrimental effects of short-term enteral glutamine supplementation in the neonatal period were found on long-term cognitive, motor and behavioural outcomes of very preterm and/or VLBW children at school age.
Acknowledgements
The present study was supported by an unrestricted research grant from Danone® (Friederichsdorf, Germany). Danone® also supported manufacture of the glutamine supplement used in the present study but had no involvement in the analysis of the data or the interpretation of the results of this investigation. J. F. d. K. carried out the practical work, the analyses and wrote the manuscript. In addition, A. v. Z., G. B. and H. N. L. co-wrote the manuscript and gave advice. J. O. and R. M. v. E. co-wrote the manuscript, gave advice and supervised the project. J. F. d. K., J. O., A. v. Z., H. N. L. and R. M. v. E. have no conflict of interest. G. B. is an employee of Danone Research, Centre for Specialised Nutrition.