Introduction
The invasive fall armyworm, Spodoptera frugiperda (J. E. Smith), and the common cutworm, Spodoptera litura (Fabricius) (Lepidoptera: Noctuidae), are both extremely polyphagous and cosmopolitan pests that undergo widespread dispersal and can feed on many economically important crops, such as maize, sorghum, wheat, cotton, groundnut, soybean and vegetables (Rao et al., Reference Rao, Wightman and Rao1993; Montezano et al., Reference Montezano, Specht, Sosa-Gomez, Roque-Specht, Sousa-Silva, Paula-Moraes, Peterson and Hunt2018). Their early instar larvae feed gregariously on plant leaves, and later instars are highly voracious and can attack most parts of the plant, often causing severe damage to crops (Sparks, Reference Sparks1979; Gupta et al., Reference Gupta, Tara, Sharma and Bala2015). Infestations of S. frugiperda can cause yield losses of 15–73% in maize (Hruska and Gould, Reference Hruska and Gould1997), and maize crops worth USD 18 billion per year have been at risk of fall armyworm damage throughout Africa, the Near East and the Asia-Pacific region since its invasion (FAO, 2020). The economic loss caused by S. litura can reach between 25.8 and 100% in crops (Dhir et al., Reference Dhir, Mohapatra and Senapati1992). Because of their high reproductive capacity and associated heavy crop losses, S. frugiperda and S. litura are considered to be the most destructive insect pests in many countries of the Asia-Pacific region, including China, Japan, India and Pakistan, where these two species threaten agricultural production, food security and the livelihoods of farmers (Ahmad et al., Reference Ahmad, Ghaffar and Rafiq2013; Early et al., Reference Early, Gonzalez-Moreno, Murphy and Day2018; FAO, 2020).
Currently, management of S. frugiperda and S. litura depends mostly on application of various insecticides. However, many field populations of S. frugiperda and S. litura have developed high resistance to conventional and newer insecticides, which leads to frequent failures with chemical pest control (Ahmad et al., Reference Ahmad, Sayyed, Saleem and Ahmad2008; Tong et al., Reference Tong, Su, Zhou and Bai2013; Gutierrez-Moreno et al., Reference Gutierrez-Moreno, Mota-Sanchez, Blanco, Whalon, Teran-Santofimio, Rodriguez-Maciel and DiFonzo2019; Wan et al., Reference Wan, Huang, Li, Zhou, Ren, Li, Xing, Zhang, Qiao, Liu, Liu, Xi, Liu, Wang, Qian, McKirdy and Wan2021). An alternative and ecologically friendly control strategy against S. frugiperda and S. litura is biological control using natural enemies. As a key component of integrated pest management, biological control has been successfully applied against a wide range of agricultural pests throughout the world during the past 130 years (Bale et al., Reference Bale, Van Lenteren and Bigler2008). Many studies have also shown that biological control is the most environmentally safe and economically profitable pest management method (Bale et al., Reference Bale, Van Lenteren and Bigler2008; Van Lenteren, Reference Van Lenteren2011; Yang et al., Reference Yang, Zang, Wang, Guo, Xu, Zhang and Wan2014).
Green lacewings are proven broad-spectrum biological control agents against a variety of soft-bodied pests, including aphids, whiteflies, thrips, mites and scales, as well as eggs and small larvae of Lepidoptera and Coleoptera (Canard et al., Reference Canard, Séméria and New1984; McEwen et al., Reference McEwen, New and Whittington2010). The efficiency of several chrysopid species in the family of Chrysopidae, such as Chrysopa pallens (Rambur), Chrysoperla sinica (Tjeder) and Chrysoperla carnea (Stephens), in controlling S. frugiperda and S. litura has been well recognized (Tauber et al., Reference Tauber, Tauber, Daane and Hagen2000; McEwen et al., Reference McEwen, New and Whittington2010; Huang et al., Reference Huang, Liu, Qi, Xu and Chen2020; Li et al., Reference Li, Li, Xiang, Wang, Mao, Chen and Zhang2020). However, there is a limited number of chrysopid species that can be used commercially for release in biological control programs, and there is an urgent need to exploit other species and to evaluate their predation efficiency. The polyphagous predator Chrysopa formosa (Brauer) is widely distributed in the region of Asia and Europe, and it is a very efficient predator of many kinds of pests, as both its larvae and adult feed on prey (Li et al., Reference Li, Wang, Gao, Zhang, Chen, Wang, Liu and Zhang2018). This predator has been successfully mass-reared within insectaries and released into cotton fields and greenhouses for controlling several pests, including aphids, whiteflies, spider mites and cotton bollworm, in China (Yang et al., Reference Yang, Zang, Wang, Guo, Xu, Zhang and Wan2014; Zhou et al., Reference Zhou, Yu, Tan, Chen and Feng2014; Lai and Liu, Reference Lai and Liu2020). C. formosa may be a good candidate for suppressing the invasive pests Hyphantria cunea (Drury) (Nan et al., Reference Nan, Song, Zuo, Yang, Wang and Sun2019), Aleurodicus dispersus (Russell) (Li et al., Reference Li, Zheng, Ye, Han, Yan and Chen2010) and Dysmicoccus neobrevipes (Beardsley) (Yan, Reference Yan2012). In our previous studies, we showed that the second- and third-instar larvae of C. formosa have a high predation on eggs and young larvae of S. frugiperda and S. litura (Li et al., Reference Li, Wang, Zhang, Ma, Li, Mao, Chen and Zhang2021; Wang et al., Reference Wang, Li, He, Zhang, Wang, Zhang and Li2022). In contrast, the ability of the chrysopid adult to prey on these two Spodoptera pests has not been studied. Thus, the aim of this study was to evaluate the predation efficiency of C. formosa adults toward S. frugiperda and S. litura.
The functional response of a predator refers to the number of prey consumed at different prey densities, and it defines the capacity of a predator to adjust its predation rate to the changing density of its prey (Solomon, Reference Solomon1949). The functional response is commonly used to assess the efficiency of predators in regulating the population of their prey, because it is an important component in the predator–prey dynamics (Hassanpour et al., Reference Hassanpour, Mohaghegh, Iranipour, Nouri-Ganbalani and Enkegaard2011; Van Lenteren et al., Reference Van Lenteren, Hemerik, Lins and Bueno2016; Feng et al., Reference Feng, Zhou, An, Yu and Liu2018). Three types of functional responses in relation to prey density have been described by Holling (Reference Holling1959). Type I is represented by a linear increase in the predation rate, type II corresponds to an increase at a decelerating rate to a plateau and type III is represented as a sigmoid increase. The analysis of functional response characteristics is important for understanding predation efficiency of a predator and for evaluating the potential of a predator as a candidate for biological control programs (Cuthbert et al., Reference Cuthbert, Dick, Callaghan and Dickey2018; Pan et al., Reference Pan, Zhang, Zhang and Chen2019; Qin et al., Reference Qin, Wu, Qiu, Ali and Cuthbertson2019; Viteri Jumbo et al., Reference Viteri Jumbo, Teodoro, Rego, Haddi, Galvao and de Oliveira2019). As S. frugiperda expands its range across China, and even the globe, the potential for using native natural enemies is uncertain. Understanding the predation capacity of generalist predators native to the newly invaded regions will help us to guide management strategies for this invasive pest.
In this study, we evaluated the potential of C. formosa as a candidate biocontrol agent for managing S. frugiperda and S. litura by conducting functional response experiments, in which we used adult predator feeding on eggs and the first three instar larval stages of the prey. The predation capacity, functional response type and the parameters of C. formosa adult predation against each life stage of the prey were determined. We also compared the consumption ability and prey preference of C. formosa toward S. frugiperda and S. litura in one-prey and two-prey systems.
Materials and methods
Insect culture
A colony of the green lacewing, C. formosa, was maintained in the laboratory as described (Li et al., Reference Li, Wang, Gao, Zhang, Chen, Wang, Liu and Zhang2018). Larvae and adults of C. formosa were all reared with abundant aphids, Megoura japonica (Matsumura), in a cage (60 cm in length, 60 cm in width and 60 cm in height) under conditions of 26 ± 1°C, 16 h light/8 h dark (16L:8D) and 70 ± 5% relative humidity (RH). Adults that emerged on the same day were collected and cultured with aphids in a separate cage. C. formosa adults were then collected for functional responses, consumption of prey species and prey preference analysis 2–4 days after emergence.
The fall armyworm S. frugiperda was originally collected from maize fields in Chongming county (102.76°E, 25.12°N), Yunnan province, China, during May 2019. Eggs for the common cutworm S. litura were provided by the Jilin Academy of Agricultural Sciences. The first- to third-instar larvae of S. frugiperda were fed on an improved artificial diet (Greene et al., Reference Greene, Leppla and Dickerson1976), and S. litura larvae were also reared with specific artificial diets (Chen et al., Reference Chen, Li, Wang, Mao and Zhang2021). These two species colonies were cultured in a climatic chamber at 26 ± 1°C and with 16L:8D and 70 ± 5% RH. Adults were fed with a 10% sucrose solution. In preliminary predation experiments, a single green lacewing C. formosa adult could consume S. frugiperda eggs, first and second-instar larvae and also could consume S. litura eggs and first-, second- and third-instar larvae. Therefore, eggs laid within 1 day and 1- to 2-day-old first and second-instar larvae of S. frugiperda and S. litura and 1-day-old third-instar larvae of S. litura were collected directly from the chambers for experimentation.
Experimental conditions
All experiments were performed under conditions of 26 ± 1°C, 16L:8D and 70 ± 5% RH. Prior to each experiment, adults of C. formosa were starved for 24 h by keeping them individually in Petri dishes (6 cm in diameter). Each experimental arena (a Petri dish; 15 cm in diameter, 2.3 cm in height) contained a small piece of artificial diets to prevent cannibalism among the prey. After allocating the prey and predator, each Petri dish was covered and sealed with parafilm to prevent the prey from escaping.
C. formosa consumption of different prey species
To assess the ability of C. formosa to consume different prey species, 500 newly laid individual eggs, 200 first-instar larvae and 40 second-instar larvae of S. frugiperda and S. litura were offered separately to individual C. formosa adults. After 24 h, the number of individuals consumed was recorded by counting intact or living prey. For each prey life stage, 10–20 replicates were conducted.
Prey preference
To determine the relative preference of C. formosa for S. frugiperda and S. litura, a 24-h starved C. formosa adult and 50 first-instar larvae each of S. frugiperda and S. litura (for a total of 100 prey items) were transferred into an experimental arena. After 24 h, the number of prey consumed for each species was recorded. Because it is hard to distinguish eggs and the second-instar larvae of the two Spodoptera species morphologically, here we only studied the preference of C. formosa for first-instar larval prey. Sixteen adult females and males were used as replicates.
Determination of the functional responses
Prey densities to be offered were determined through preliminary tests to ensure that minimum and maximum levels of predation would be obtained for each predator on each prey stage. Each 24-h starved female or male adult was exposed to five different densities of S. frugiperda or S. litura at different life stages (eggs and first-, second- and third-instar larvae). Prey densities offered to C. formosa adults are shown in table 1. Prey consumption was checked after a foraging period of 24 h, and the number of surviving prey individuals was recorded. In each treatment, 5–10 females and males (a total of 10–20 adults) were used as replicates for each prey density. Control treatments without predators were carried out and consisted of ten arenas for each prey density to determine the natural mortality by counting the dead prey.
Table 1. Prey densities offered for functional responses of C. formosa adults to S. frugiperda and S. litura

a Prey densities are shown as the number of eggs or larvae per 15-cm dish.
Statistical analyses
There was no mortality for S. frugiperda or S. litura larvae in control groups, and thus prey mortality data did not have to be corrected in the experiments. A one-way analysis of variance (ANOVA) was performed to compare the amount and proportion of prey consumed by C. formosa at different prey densities. When significant differences were detected, the means were analyzed by Tukey's multiple comparisons (P < 0.05). The consumption rates of C. formosa with respect to different life stages of S. frugiperda and S. litura at the highest prey densities were also compared by one-way ANOVA, followed by Tukey's multiple comparisons (P < 0.05). Comparisons of prey consumption amount and prey preference of C. formosa for the two Spodoptera species were performed using the t-test. The prey preference was assessed by using Ivlev's selectivity index (Lechowicz, Reference Lechowicz1982). The selectivity index Ci = (Qi − Fi)/(Qi + Fi), in which Fi is the proportion of prey type i in the experimental system, Qi the proportion of prey type i consumed by the predator and Ci represents the preference of the predator for the prey type i. If 0 < Ci < 1, there is a positive preference; if −1 < Ci < 0, there is a negative preference and Ci = 0 indicates no preference.
The type of functional response was determined by logistic regression of the proportion of prey consumed as a function of initial prey density, using a polynomial logistic regression fitted to the data:

where N e is the number of prey consumed; N 0 is the initial prey density and P 0, P 1, P 2 and P 3 are the maximum likelihood estimates of the intercept, linear, quadratic and cubic coefficients, respectively (Juliano, Reference Juliano, Cheiner and Gurven2001). The sign of the linear and quadratic coefficients is used to determine the type of functional response. If the linear coefficient (P 1) is not significantly different from 0, functional response is type I. If the linear coefficient is significantly negative (i.e., P 1 < 0), the predator displays a type II functional response. If the linear coefficient is positive (i.e., P 1 > 0) and the quadratic coefficient is negative (i.e., P 2 < 0), the predator displays a type III functional response (Juliano, Reference Juliano, Cheiner and Gurven2001). The logistic regression analysis indicated that our data fit a type II functional response for C. formosa adults (table 2). Therefore, we used the random predator equation (Rogers, Reference Rogers1972), which allows for prey depletion, for estimation as below:

in which N e is the number of prey consumed, N 0 is the initial prey density, a is the attack rate, T is the searching time (1 day) and T h is the handling time in days. These variables can also be used to calculate the maximum prey consumption, T/T h. The extra sum-of-squares F-test was used to test for differences in the functional response attack rate and handling time between the two-prey species and among different prey stages. The functional response ratio (FRR = a/T h) (Cuthbert et al., Reference Cuthbert, Dickey, Coughlan, Joyce and Dick2019), which is used to predict the predation efficiency of the predator, was also calculated. Parameters were estimated using the non-linear least squares regression procedure. All data were analyzed using Prism 9.0 (GraphPad) and SigmaPlot (version 14, Systat Software, Inc.).
Table 2. Parameters estimated from logistic regression analysis of the proportion of S. frugiperda and S. litura consumed by C. formosa adults as a function of initial prey density

Results
Prey consumption
Adults of C. formosa exhibited high predation on the eggs and on the first two instar larval stages of S. frugiperda and S. litura when provided with individual prey species. When provided with the third-instar larvae of either prey, the green lacewing adult could only attack and kill S. litura successfully. In all cases, the number of prey consumed by C. formosa over a 24-h period increased significantly with the increase in prey densities and then attained a plateau when prey densities approached the upper asymptote (fig. 1). A larger variation in predation amounts was noted among predator individuals as prey densities increased. At the highest prey densities, the average daily consumption of C. formosa declined significantly with the development of the prey (table 3). For each prey species, the largest number of prey consumed was observed for eggs, followed by the first and second-instar larvae, respectively (table 3).
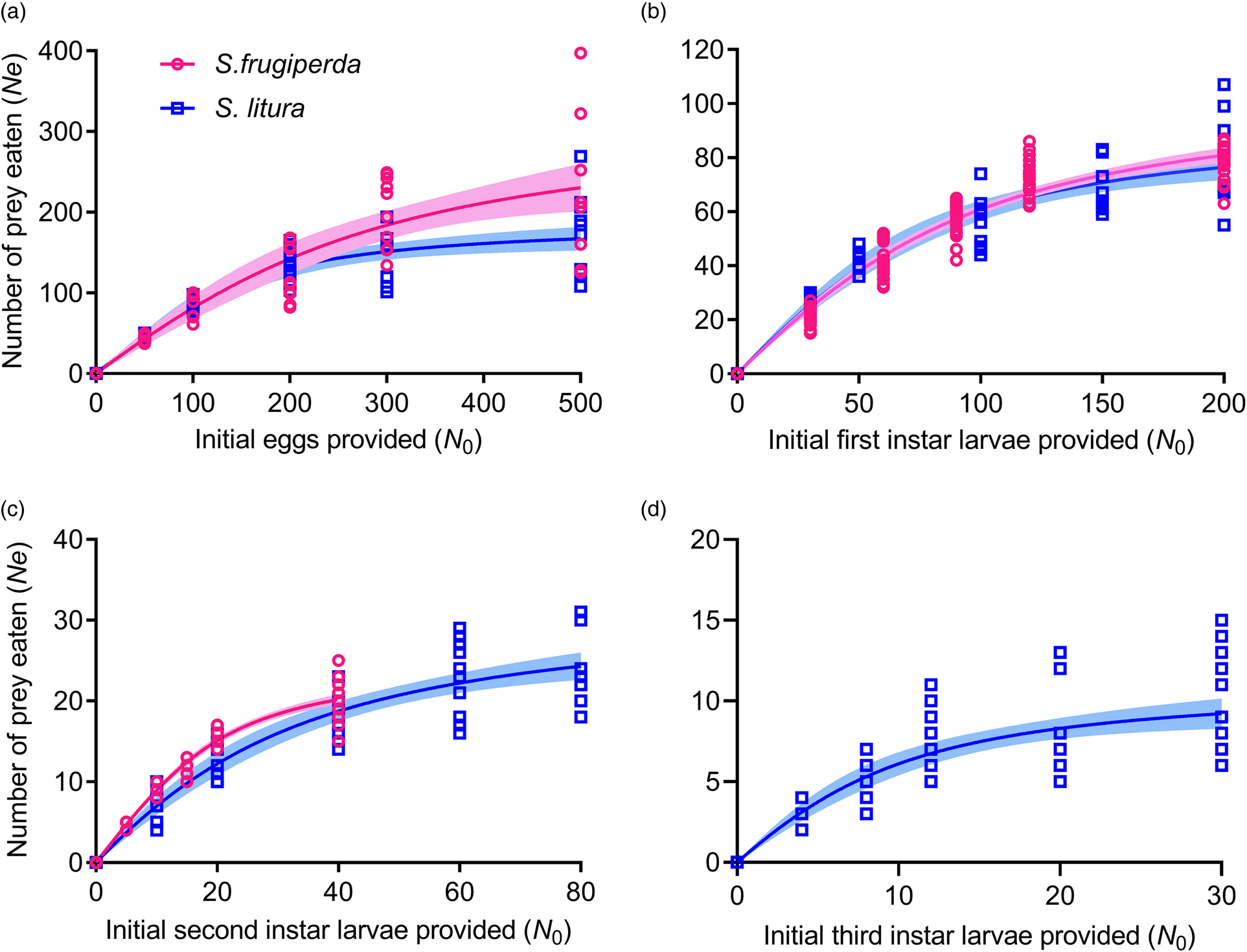
Figure 1. Functional response of C. formosa adults to different life stages of S. frugiperda and S. litura. (a–d) Prey consisted of eggs (a) and first (b), second (c) and third (d) instar larvae of S. frugiperda and S. litura at different densities as indicated. Data points represent the observed number of S. frugiperda and S. litura consumed at each initial prey density over a period of 24 h. Curves show predicted values based on Rogers random predator equation. Shaded areas indicate 95% confidence intervals.
Table 3. Prey consumption (±SD) per day for C. formosa adults with respect to different life stages of S. frugiperda and S. litura at the highest prey density

Each value represents the mean ± SD (n = 10–20). Means within the same column followed by different lowercase letters were significantly different among different stages of prey consumed by C. formosa adults (one-way ANOVA followed by Tukey's multiple comparisons, P < 0.01).
Prey species had no statistically significant effect with respect to consumption by C. formosa when the same number of eggs or first-instar larvae of S. frugiperda and S. litura was offered as prey in one-prey system (fig. 2). For C. formosa feeding on the second-instar larvae, there was a slight but significantly greater predation on S. frugiperda than on S. litura (t = 2.666, df = 28, P = 0.0126).

Figure 2. Number of S. frugiperda and S. litura consumed by C. formosa adults in a one-prey system. S. frugiperda and S. litura were offered separately as prey at the same density and life stage. An asterisk indicates a significant difference based on a t-test.
Prey preference
C. formosa adults consumed significantly larger numbers of first-instar larvae of S. litura than of S. frugiperda, regardless of the predator's sex (female: t = 2.418, df = 30, P = 0.0219; male: t = 2.463, df = 28, P = 0.0202) (fig. 3a). Both female and male adults showed a positive preference for the first-instar larvae of S. litura and displayed a negative preference for S. frugiperda at the same life stage (fig. 3b). There was no significant difference in the consumption rates between female and male adults of C. formosa.
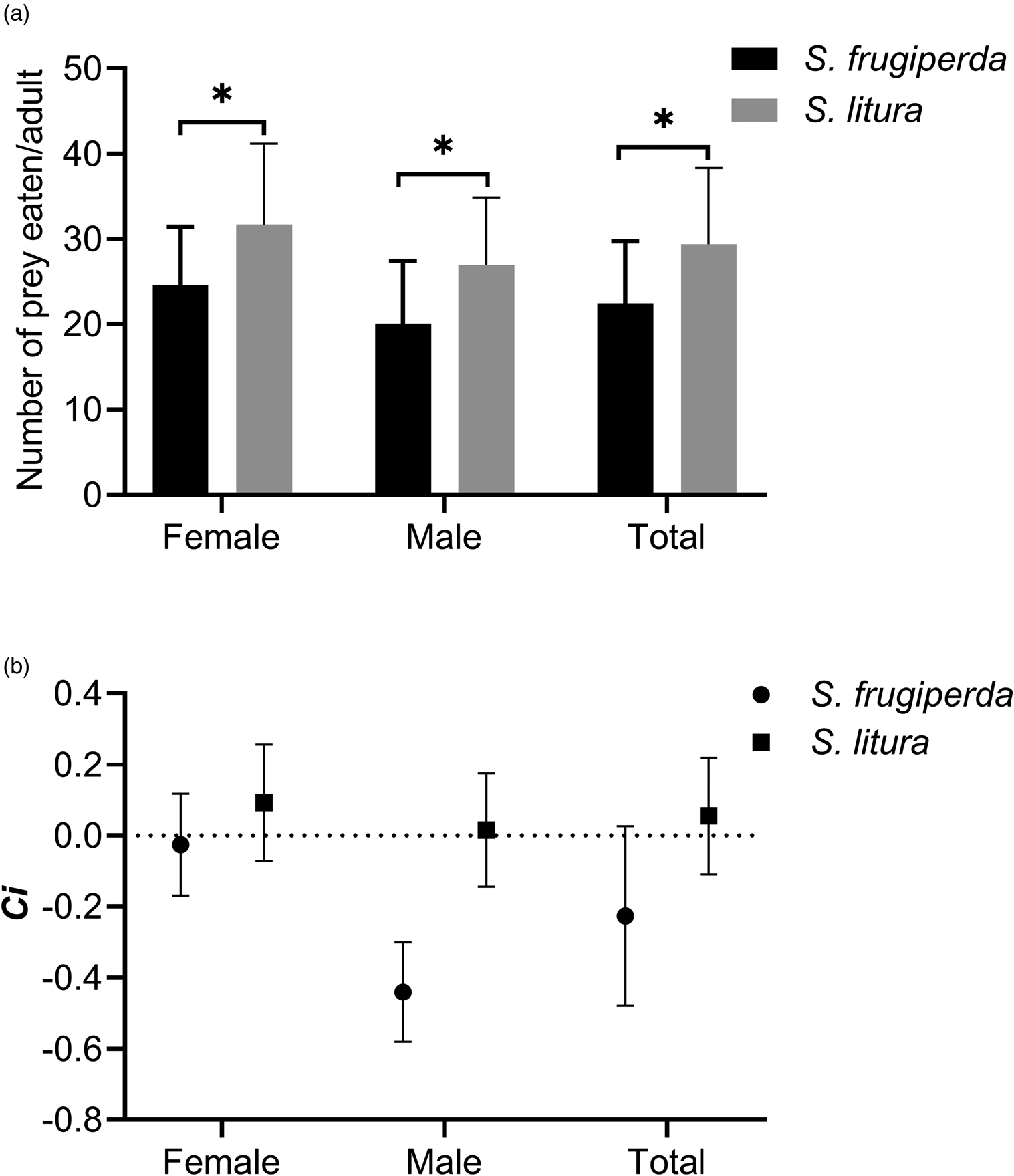
Figure 3. Mean consumption amount (a) and prey preference (Ci) (b) of C. formosa female and male adults feeding on S. frugiperda and S. litura in a two-prey system. An asterisk indicates a significant difference based on a t-test.
Functional response
Type II functional responses were displayed by C. formosa adults toward each stage of S. frugiperda and S. litura, when they were offered separately, as evidenced by a significantly negative maximum likelihood estimate of the linear coefficient (i.e., P 1 < 0) (table 2) and decreased rates of consumption with increasing prey densities (figs 1, 4 and 5). The functional response data for C. formosa feeding on S. frugiperda or S. litura over a 24-h period fit the random predator equation well, also confirming a type II response for all prey life stages (table 4). The searching efficiency of C. formosa, as measured by the proportion of prey consumed, was higher at lower densities of all prey stages but declined significantly as the prey density increased (figs 4 and 5).

Figure 4. Proportion of S. frugiperda at different life stages consumed by C. formosa adults. (a–c) Consumption was assessed for S. frugiperda eggs (a) and first-instar (b) and second-instar (c) larvae when provided at the indicated densities. Values are the mean ± SD (n = 10–20). Different letters indicate significant differences (Tukey's multiple comparisons, P < 0.05).

Figure 5. Proportion of S. litura at different life stages consumed by C. formosa adults. (a–d) Consumption was assessed for S. litura eggs (a) and first-instar (b), second-instar (c) and third-instar (d) larvae when provided at the indicated densities. Values are the mean ± SD (n = 10–20). Different letters indicate significant differences (Tukey's multiple comparisons, P < 0.05).
Table 4. Parameter estimates (±SE) from the type II functional response of C. formosa adults preying on different life stages of S. frugiperda and S. litura

Values in the same column for an individual prey species followed by different lowercase letters are significantly different as determined by the extra sum-of-squares F-test. †, ‡, §The same symbols indicate significant differences for estimated parameters a and T h between the same stage of the two-prey species (extra sum-of-squares F-test).
Attack rates and handling time of C. formosa varied depending on the life stage of the prey. The highest attack rate was observed for predators feeding on the second-instar larvae of S. frugiperda and eggs of S. litura. However, there was no significant difference among the attack rates estimated for different life stages of each prey type (table 4). In all cases, the handling time of C. formosa increased significantly as the prey developed from one growth stage to another. Prey species also had a distinct effect on functional response parameters, as reflected in the higher attack rate (F (1, 176) = 24.03, P < 0.0001) and longer handling time (F (1, 176) = 9.186, P = 0.0028) of C. formosa feeding on the second-instar larvae of S. frugiperda in contrast to S. litura second-instar larvae, whereas predators feeding on S. frugiperda eggs showed a shorter handling time in contrast to S. litura eggs (F (1, 113) = 9.12, P = 0.0031) (table 4).
In many cases, the use of either attack rate (a) or handling time (T h) alone would lead to contradictory predictions of predatory efficiency, and thus a new metric, the FRR (a/T h) was proposed to resolve such contradictions (Cuthbert et al., Reference Cuthbert, Dickey, Coughlan, Joyce and Dick2019). High attack rate values and short-handling times should be associated with high predation efficiency, and vice versa. C. formosa adults had the highest predation efficiency on eggs of each prey species (FRR = 705.3 for S. frugiperda eggs, FRR = 658.3 for S. litura eggs), followed by the first-instar larvae (table 4). The predation efficiency dropped notably with the development of the prey. When provided with eggs or second-instar larvae of the two-prey species separately, the C. formosa adults exhibited slightly higher predation efficiency on S. frugiperda than on S. litura, but when feeding on the first-instar larvae, C. formosa had similar predation efficiency for the two-prey species.
The theoretical maximum consumption (T/T h) of C. formosa estimated according to handling time was close to the measured average daily consumption at the highest prey density, suggesting that parameters of functional response were reliable for predicting the predation ability of C. formosa (tables 3 and 4). This parameter also confirmed the results we obtained from a/T h that the predation efficiency of C. formosa decreased with the development of the prey.
Discussion
Our results indicated that the green lacewing, C. formosa, is a voracious predator of Spodoptera pests, with a high predation on eggs and earlier instar larvae of both S. frugiperda and S. litura. To our knowledge, this is the first study to provide insights into the predation capacity and functional response of C. formosa adults on S. frugiperda and S. litura. The predation capacity of the chrysopid toward eggs and the first two larval stages of S. frugiperda was relatively similar to its capacity toward those of S. litura. It was difficult for C. formosa adults to attack and kill the third-instar larvae of S. frugiperda, most likely because of their strong defensive behavior (Li et al., Reference Li, Li, Xiang, Wang, Mao, Chen and Zhang2020). We note, however, that adults of a larger chrysopid species, C. pallens, can consume one to three third-instar larvae of S. frugiperda per day (Cao et al., Reference Cao, Zhang, Yang, Xu, Gu and Chen2020). Larval stages of C. formosa also consume large numbers of eggs and young larvae of both S. frugiperda and S. litura, with the third-instar larval stage of C. formosa being the most voracious among these stages, as determined by our previous work (table 5) (Li et al., Reference Li, Wang, Zhang, Ma, Li, Mao, Chen and Zhang2021; Wang et al., Reference Wang, Li, He, Zhang, Wang, Zhang and Li2022). The maximum consumption of C. formosa adult against the first- and second-instar larvae of S. frugiperda is higher than that of adult C. pallens (Cao et al., Reference Cao, Zhang, Yang, Xu, Gu and Chen2020), whereas the larval stages of C. formosa have lower predation than C. pallens larvae (Li et al., Reference Li, Li, Xiang, Wang, Mao, Chen and Zhang2020, Reference Li, Wang, Zhang, Ma, Li, Mao, Chen and Zhang2021). Based on another study, C. pallens adults consume a larger number of S. frugiperda eggs than do C. formosa adults (table 5) (Xu et al., Reference Xu, Wang, Tian, Wang, Zhang, Ma, Li and Di2019). Nunes et al. (Reference Nunes, Nascimento, Souza, Oliveira, Oliveira and Batista2017) reported that the third-instar larvae of Ceraeochrysa cubana (Hagen) could consume 47.4 eggs and 63.5 newborn caterpillars of S. frugiperda daily, which is similar to consumption by C. formosa (46.2 ± 8.9 eggs and 66.8 ± 13.3 first-instar larvae) (Li et al., Reference Li, Wang, Zhang, Ma, Li, Mao, Chen and Zhang2021). When feeding on S. litura at similar stages, C. formosa adults also exhibited higher predation than other predators, such as Harmonia axyridis (Pallas) (Islam et al., Reference Islam, Shah, Shah, Musa Khan, Rasheed, Ur Rehman, Ali and Zhou2020), Cyrtopeltis tenuis (Reuter) and Harpactor fuscipes (Fabricius) (Wang et al., Reference Wang, Li, He, Zhang, Wang, Zhang and Li2022). These results indicate the potential use of C. formosa as an effective predator, with the adult stage and last instar larval stage having the most potential for biological control.
Table 5. Summary of recent studies on the functional response of chrysopid species to noctuid pests

a To facilitate comparisons, the handling time was converted to days.
b Values were calculated based on the data presented in the references.
A steady decline in the predation efficiency of predators with the growth of the prey has been demonstrated in many chrysopids (Hassanpour et al., Reference Hassanpour, Mohaghegh, Iranipour, Nouri-Ganbalani and Enkegaard2011; Tavares et al., Reference Tavares, Cruz, Silva, Serrao and Zanuncio2011; Ganjisaffar and Perring, Reference Ganjisaffar and Perring2015; Qin et al., Reference Qin, Wu, Qiu, Ali and Cuthbertson2019). Similarly, the highest consumption and shortest-handling time was observed for adults of C. formosa feeding on eggs, followed by the first-instar larvae of both prey species. The higher predation of this chrysopid on eggs may be associated with their lower biomass and lack of defenses and with the easier handling of immobile eggs. In terms of nutrient requirements of the chrysopid, lepidopteran eggs and newborn caterpillars are generally considered as optimal prey for the development of lacewings (Huang and Enkegaard, Reference Huang and Enkegaard2010). Consistently, feeding with S. frugiperda eggs increases the survival and reduces the larval and adult developmental time of the lacewing C. cubana (Nunes et al., Reference Nunes, Nascimento, Souza, Oliveira, Oliveira and Batista2017). Chrysoperla externa could successfully reared on S. frugiperda eggs and newly hatched larvae, but fails to develop from larvae to adults when fed with 1- to 2-day-old first-instar larvae of S. frugiperda (Tavares et al., Reference Tavares, Cruz, Silva, Serrao and Zanuncio2011). The lower predation on Spodoptera larvae was probably a result of defensive behavior and more rapid movement with the increase in prey size, which made larvae more difficult to attack, subdue and consume, thus leading to rejection of the prey (Zanuncio et al., Reference Zanuncio, da Silva, de Lima, Pereira, Ramalho and Serrao2008). Our results indicate that the lacewing C. formosa will be an effective control agent early in an infestation when the two Spodoptera populations comprise more eggs and earlier instar larvae.
Feeding preference of polyphagous predators is one of the most important criteria used in the evaluation of their efficiency. As generalist predators that prey on many soft-bodied insects and mites, the consumption efficiency of the chrysopids is often influenced by prey preference and the presence of alternative prey (Pappas et al., Reference Pappas, Broufas and Koveos2007; Huang and Enkegaard, Reference Huang and Enkegaard2010; Tavares et al., Reference Tavares, Cruz, Silva, Serrao and Zanuncio2011; Messelink et al., Reference Messelink, Vijverberg, Leman and Janssen2016). The current experiments provide evidence that C. formosa has a preference for S. litura relative to S. frugiperda when both prey types are present, whereas their consumption rates were similar for this chrysopid species in a one-prey system. Preference of predators for different prey species often correlates with body size, nutrient quality and behavior traits of the prey (Pappas et al., Reference Pappas, Broufas and Koveos2007; Huang and Enkegaard, Reference Huang and Enkegaard2010; Messelink et al., Reference Messelink, Vijverberg, Leman and Janssen2016; Sattayawong et al., Reference Sattayawong, Uraichuen and Suasa-ard2016). A strong preference of C. carnea for aphids as compared with Pieris brassicae (Linnaeus) eggs might be a reflection of variation in nutritional quality (Huang and Enkegaard, Reference Huang and Enkegaard2010). Conversely, Chrysoperla rufilabris second- and third-instar larvae have a preference for the larger size of active caterpillars of Heliothis virescens (Fabricius) as compared with nymphs and adults of the cotton aphid Aphis gossypii Glover, but they prefer to feed on aphids over H. virescens eggs when offered the choice (Nordlund and Morrison, Reference Nordlund and Morrison1990). Among three cassava mealybug species, the higher preference of the green lacewing Plesiochrysa ramburi (Schneider) larvae for Phenacoccus manihoti (Matile-Ferrero) and Phenacoccus madeirensis (Green) relative to Pseudococcus jackbeardsleyi (Gimpel & Miller) is probably due to the softer and smaller body size of the first two species (Sattayawong et al., Reference Sattayawong, Uraichuen and Suasa-ard2016). As the armyworms S. frugiperda and S. litura have very similar size and host ranges (Pogue, Reference Pogue2002; EPPO, 2015), we suspect that the higher degree of aggressiveness, stronger defenses and greater escape response of S. frugiperda larvae may lead C. formosa to reject that prey more frequently when alternatives are available (Li et al., Reference Li, Li, Xiang, Wang, Mao, Chen and Zhang2020). As evidenced by our observations, adults of C. formosa consumed 9.4 ± 3.1 third-instar larvae of S. litura daily but rarely attacked and fed on S. frugiperda third-instar larvae. Thus, caution must be taken when using this chrysopid to control S. frugiperda in the presence of S. litura in nature, because the functional response and predation efficiency may be affected by non-target prey species as supported by several studies (Nachappa et al., Reference Nachappa, Braman, Guillebeau and All2006; Messelink et al., Reference Messelink, Vijverberg, Leman and Janssen2016). The prey preference and predation efficiency of C. formosa in complex environments with multiple prey species should be further evaluated in the field.
C. formosa adults exhibited a type II functional response toward all prey stages for both prey species. A type II functional response is characterized by an inverse density-dependent relationship between the consumption rate and prey density, with the maximum consumption being limited by the handling time (Holling, Reference Holling1965). This kind of functional response is commonly found among chrysopid species, including C. pallens (Cao et al., Reference Cao, Zhang, Yang, Xu, Gu and Chen2020; Li et al., Reference Li, Li, Xiang, Wang, Mao, Chen and Zhang2020), Chrysopa oculata (Parajulee et al., Reference Parajulee, Shrestha, Leser, Wester and Blanco2006), C. sinica (Huang et al., Reference Huang, Liu, Qi, Xu and Chen2020; Lai and Liu, Reference Lai and Liu2020), C. carnea (Hassanpour et al., Reference Hassanpour, Mohaghegh, Iranipour, Nouri-Ganbalani and Enkegaard2011; Sultan and Khan, Reference Sultan and Khan2014), C. rufilabris (Stewart et al., Reference Stewart, Braman and Pendley2002) and Ceraeochrysa caligata (Viteri Jumbo et al., Reference Viteri Jumbo, Teodoro, Rego, Haddi, Galvao and de Oliveira2019). However, type III functional responses can be observed for several chrysopids, such as the third-instar larvae of C. carnea feeding on Helicoverpa armigera (Hübner) eggs (Hassanpour et al., Reference Hassanpour, Mohaghegh, Iranipour, Nouri-Ganbalani and Enkegaard2011) and sugar cane whitefly Aleurolobus barodensis (Maskell) (Sultan and Khan, Reference Sultan and Khan2014), the third-instar larvae of Chrysoperla congrua on H. armigera eggs (Kabissa et al., Reference Kabissa, Yarro, Kayumbo and Juliano1996) and the second-instar larvae of C. caligata preying upon red palm mite Raoiella indica (Hirst) (Viteri Jumbo et al., Reference Viteri Jumbo, Teodoro, Rego, Haddi, Galvao and de Oliveira2019). Although predators with type II functional responses are considered less efficient than those with type III responses in long-term suppression of prey populations (Holling, Reference Holling1965), the chrysopids with a type II response can be more effective at lower prey densities and thus used for augmentative biological control. In addition to prey species and size, the predation efficiency of a predator also could be influenced by the numerical response of a group of predators (Mushtaq and Khan, Reference Mushtaq and Khan2010), the hunger level of a predator (Hassanpour et al., Reference Hassanpour, Maghami, Rafiee-Dastjerdi, Golizadeh, Yazdanian and Enkegaard2015; Pan et al., Reference Pan, Zhang, Zhang and Chen2019), intraguild predation (Noppe et al., Reference Noppe, Michaud and De Clercq2012), prey distribution (Feng et al., Reference Feng, Zhou, An, Yu and Liu2018), host plants (Barbosa et al., Reference Barbosa, Poletti and Poletti2019) and abiotic environmental factors (Parajulee et al., Reference Parajulee, Shrestha, Leser, Wester and Blanco2006; Ziaei Madbouni et al., Reference Ziaei Madbouni, Samih, Namvar and Biondi2017). Under complex environmental conditions in the field, the predator could exhibit a type III functional response caused by the growth of its body size (Viteri Jumbo et al., Reference Viteri Jumbo, Teodoro, Rego, Haddi, Galvao and de Oliveira2019), prey switching (Schenk and Bacher, Reference Schenk and Bacher2002) or the size of the search area (Feng et al., Reference Feng, Zhou, An, Yu and Liu2018). To improve their biological control efficiency, chrysopids with a type II functional response would need to be released early and at a high predator–prey ratio in an augmentative biological control program.
Attack rate and handling time are valuable indicators of predation ability, as they determine the capture success and the cumulative time invested in capturing, killing and digesting the prey (Viteri Jumbo et al., Reference Viteri Jumbo, Teodoro, Rego, Haddi, Galvao and de Oliveira2019). The two parameters may be affected by many factors, such as prey species, stage, movement and defense behaviors (Hassell, Reference Hassell1978; Ganjisaffar and Perring, Reference Ganjisaffar and Perring2015), as well as predator stage, food preference, voracity, walking speed and digesting rate (Hassanpour et al., Reference Hassanpour, Mohaghegh, Iranipour, Nouri-Ganbalani and Enkegaard2011). Based on our observations, prey stage had a distinct influence on the handling time of C. formosa for both prey species, whereas no statistically significant difference was noted for the attack rate. The attack rate and handling time of C. formosa showed a life stage-dependent difference between the two-prey species. Based on a/T h, C. formosa adults exhibited a greater predation efficiency on eggs and second-instar larvae of S. frugiperda than that of S. litura, which suggests that this chrysopid may be more efficient in controlling the invasive S. frugiperda.
The functional response of chrysopids on noctuid pests, including S. frugiperda (Xu et al., Reference Xu, Wang, Tian, Wang, Zhang, Ma, Li and Di2019; Cao et al., Reference Cao, Zhang, Yang, Xu, Gu and Chen2020; Huang et al., Reference Huang, Liu, Qi, Xu and Chen2020; Li et al., Reference Li, Wang, Zhang, Ma, Li, Mao, Chen and Zhang2021), S. litura (Wang et al., Reference Wang, Li, He, Zhang, Wang, Zhang and Li2022), H. armigera (Huang et al., Reference Huang, Yan and Li1990; Hassanpour et al., Reference Hassanpour, Mohaghegh, Iranipour, Nouri-Ganbalani and Enkegaard2011), H. virescens (Hernández-Juárez et al., Reference Hernández-Juárez, Aguirre-Uribe, González-Ruíz, Chacón-Hernández, Landeros-Flores, Cerna-Chávez, Flores-Dávila and Harris2015) and Helicoverpa zea (Boddie) (Parajulee et al., Reference Parajulee, Shrestha, Leser, Wester and Blanco2006) has been reported in several studies. As shown in table 5, we summarize the functional response type, the estimated attack rate, handling time and predation efficiency (a/T h) for these lacewing species and compare these values with those obtained for C. formosa. Most chrysopids showed a type II response against different stages of noctuid pests, with the exception of the third-instar larvae of C. carnea and C. congrua (table 5). It is obvious that the functional response parameters are generally varied for different species and stages of both prey and predator, which indicates that predators exhibiting analogous functional response curves cannot be deemed to respond similarly. As is common, but not universal (Stewart et al., Reference Stewart, Braman and Pendley2002; Viteri Jumbo et al., Reference Viteri Jumbo, Teodoro, Rego, Haddi, Galvao and de Oliveira2019), the consumption ability and attack rate of predators usually increase, but the handling time decreases as they develop (Lee and Kang, Reference Lee and Kang2004; Huang and Enkegaard, Reference Huang and Enkegaard2010; Li et al., Reference Li, Wang, Zhang, Ma, Li, Mao, Chen and Zhang2021). The adult and final instar stages of lacewing larvae generally are associated with a higher level of predation efficiency relative to earlier stages. Although there were some differences in the experimental conditions, such as the experimental arena size, duration and temperature and statistical method used in these studies (Kabissa et al., Reference Kabissa, Yarro, Kayumbo and Juliano1996; Parajulee et al., Reference Parajulee, Shrestha, Leser, Wester and Blanco2006; Hassanpour et al., Reference Hassanpour, Mohaghegh, Iranipour, Nouri-Ganbalani and Enkegaard2011; Xu et al., Reference Xu, Wang, Tian, Wang, Zhang, Ma, Li and Di2019), C. formosa adults and larval stages seem to have a relatively higher or similar predation efficiency when compared with the same stage of other chrysopid species. Currently, the most commonly used commercially available lacewings are Chrysoperla species, including C. carnea, C. sinica, C. rufilabris and Chrysoperla comanche (Banks), which exhibit their predation capacity only as larvae (Pappas et al., Reference Pappas, Broufas and Koveos2011). The great ability of C. formosa to consume prey as larvae and as adults may be an important advantage for their use in biological control.
Despite the limitations of a laboratory-determined functional response, the results presented here demonstrate that C. formosa is a promising agent for biological control of both S. frugiperda and S. litura. The most suitable time to introduce the chrysopid in augmentative biological control is when the prey population at lower densities and consists of more eggs and first- to second-instar larvae relative to later life stages. The findings can help us to understand the ecological mechanisms of predator–prey interactions in the field. However, to develop a biological control program for Spodoptera pests using C. formosa, further experiments into the foraging ability, developmental performance, prey preference, numerical response and release methods of C. formosa should be conducted under both laboratory and field conditions.
Acknowledgments
We thank Dr Hualing Wang from Hebei Agricultural University, Hebei province, China for providing valuable comments and suggestions on the manuscript. This research was financed by the National Key Research and Development Program of China (2017YFE0104900, 2019YFD1002103); International Science & Technology Innovation Program of Chinese Academy of Agricultural Sciences (CAAS-ZDRW202108); the Cooperative Project between Institute of Plant Protection, Chinese Academy of Agricultural Sciences, China and the Rural Development Administration of the Republic of Korea (PJ016496) and Major Projects of China National Tobacco Corporation [110201901038 (LS-01)].
Conflict of interest
The authors declare no conflict of interest.