INTRODUCTION
Classical swine fever (CSF) is a highly contagious viral disease of pigs that causes severe economic and social consequences for infected countries and regions [Reference Saatkamp, Berentsen and Horst1]. The CSF virus (CSFV) is an RNA virus that has been classified as a Pestivirus of the Flaviviridae family. CSFV can be transmitted within domestic and wild-pig populations by direct and indirect contacts, mainly through the movement of infected animals, the use of infected semen, or via fomites, e.g. contaminated feed or trucks [Reference Elbers, Stegeman and de Jong2, Reference Ribbens3]. Additionally, wild boars have been reported as a reservoir for the virus and a probable source of infection for domestic pigs [Reference Laddomada4–Reference Laddomada7].
Despite the financial and human resources allocated to control, eradicate, and prevent the introduction of CSFV into Europe for over 120 years, CSF remains a threat for the European pig sector. From 1996 to 2007, 8307 CSF outbreaks affecting 49% of European countries have been reported [8, 9]. From 2005 to 2007, 3812 CSF outbreaks have been reported in ten European countries (Bosnia & Herzegovina, Bulgaria, Croatia, France, Germany, Hungary, Romania, Russia, Serbia, Slovakia) [9]. The most devastating CSF incidents recorded in recent European history include the epidemics that affected Germany and Belgium in 1993–1994, in which 217 infected premises were reported [Reference Fritzemeier10, Reference Mintiens11], and The Netherlands in 1997–1998, resulting in 429 infected premises and more than 8·7 million pigs being destroyed [Reference Elber12].
Spain has experienced two CSF epidemics in the last decade, one in 1997–1998 and the other in 2001–2002. The 1997–1998 epidemic was caused by the importation of piglets from The Netherlands and resulted in 99 outbreaks in six affected provinces (Lérida, Segovia, Madrid, Toledo, Zaragoza, Sevilla), with 138 259 pigs being destroyed. The 2001–2002 epidemic affected five provinces (Lérida, Castellón, Cuenca, Valencia, Barcelona), where 49 outbreaks were reported and 70 698 pigs destroyed [Reference Edwards13, Reference Greiser-Wilke14]. These epidemics were estimated to cause losses of more than €89·5 million in 1997–1998 and more than €7·4 million in 2001–2002, respectively (Spanish Ministry of Agriculture, Fisheries and Food, unpublished observations).
The production of domestic pigs has experienced a 2·25-fold increase in Spain during the last 18 years (1990–2007) [15]. Spain is currently considered the second largest pig producer among European Union (EU) member countries after Germany [16]. Spanish pig production is still heavily dependent on the importation of pigs from other EU member countries. For example, 1 204 500 pigs were imported into Spain during 2006. Moreover, a large number of wild boars (e.g. 525 400 in 2006) are annually introduced into Spain for hunting purposes [17]. The combination of a recent history of frequent CSF outbreaks in the EU, relatively short distance for animal movements, free animal trade policy among EU countries, and large number of susceptible animals introduced annually into Spain, makes the country particularly vulnerable to the introduction of the disease during the undetected period of an epidemic. This high risk was evidenced in 1997, when CSFV spread from The Netherlands into Spain via the importation of piglets before the epidemic was detected in the country of origin [Reference Greiser-Wilke14].
Quantitative assessment of the risk of introducing CSFV into a country can help the decision-making process in the allocation of human and financial resources to the prevention and control of disease introduction at locations and times at highest risk for the disease. To the best of our knowledge, no study has been recently published quantifying the monthly risk associated with introduction of wild boar, in addition to domestic pigs, into a country or region. Moreover, in the peer-reviewed literature we failed to identify any studies assessing the temporal and spatial variation in the risk of introduction of CSF into Spain.
The present study provides quantitative estimates of the risk of CSFV introduction into Spain associated with the importation of domestic and wild boars and discriminated by province and month of introduction. These results will be useful in the design and implementation of prevention and surveillance strategies throughout the country.
METHODS
Level of data aggregation and unit of analysis
Patterns of animal movements and herd demographics are not spatially homogeneous and tend to vary temporally throughout Spain. Pig density is also heterogeneous, varying from a minimum of <0·01 herds/km2 in the province of Cantabria to a maximum of 0·47 herds/km2 in the province of Barcelona. Therefore, the risk of potential introduction and animal disease spread is expected to change with the period of the year and with the geographical region assessed. In order to address the expected spatial and temporal heterogeneity of the estimates, risk of CSFV introduction into Spain was quantified per month and at a province level. Province is the lowest administrative level of Spain at which decisions concerning animal health policy are made.
Differentiation in the risk associated with introduction of domestic and non-domestic pigs was considered important for Spain. Disease prevalence and presentation of clinical signs of CSF differ considerably between domestic and wild boars and, therefore, the probability of detection of disease is also expected to differ. Moreover, the likelihood of the Spanish domestic-pig population being exposed to CSFV-infected pigs would be different depending on whether the virus was introduced by domestic pigs or by wild boars. Because of the characteristics of the regions and premises where wild boars are introduced, CSFV-infected wild boars might be a potential source of infection for other wild boars and for Iberian pigs. Iberian pigs are a specific Spanish type of free-range pigs from which high-quality meat products are obtained. Iberian pig operations are extensive, typically delimited by fences of variable quality and state of repair. Thus, Iberian pigs have a higher risk of contact with wild boars, compared to the risk of contact with domestic pigs, which are typically introduced into confined systems. Infection of the Spanish wild-boar and Iberian-pig populations would cause additional difficulties for the control and eradication of the disease throughout the country, and would have important consequences for the ecological and economic balance of Spain. Therefore, risk associated with the introduction of CSFV through wild boars and domestic pigs was computed separately.
Model formulation
The probability of a CSF epidemic in Spain due to the importation of live susceptible animals (PI) was estimated per month (m=12), country of origin of imported pigs (c=13), province of destination of the import (g=50), and type of imported pig (t=2, domestic, d; wild boar, w) assuming a binomial process of the form

where n cgtm was the number of pigs of type t imported from country c into province g at month m and p cgtm is the probability that an infected pig of type t was introduced from country c into province g and effectively contacted a susceptible pig at month m.
In general, this model is similar to an approach previously used to model the risk of introduction of foot-and-mouth disease (FMD) into Spain [Reference Martínez-López18].
The conditional probability p cgtm was estimated as the product of five independent events (P i, i=1–5)

where P 1 is the probability of a country c being infected at month m; P 2 is the probability of selecting a CSFV-infected pig of type t from country c at month m to be exported into Spanish province g before detection of CSF in country c; P 3 is the probability that an infected pig of type t survived the CSFV infection; P 4 is the probability that an infected pig of type t reached premises in Spain and, P 5 is the probability that an infected pig of type t transmitted the disease to a susceptible pig on Spanish premises.
Description of input distributions
Input parameters were parameterized using gamma, normal, Pert, and beta probability distributions. An extended description of the nature and characteristics of those probability distributions can be found elsewhere [19].
The number of type t pigs imported from country c into province g at month m, n cgtm, was modelled using a normal distribution with parameters μcgtm and σcgtm, where μcgtm is the number of type t pigs imported from country c into Spanish province g at month m of 2006, which is the most recent year for which information was available and, σcgtm is the standard deviation of the number of type t pigs imported from country c into Spanish province g at month m from 2000 to 2006 [17]. This approach is consistent with the assumption that the number of pigs expected to be imported from a given EU country to a given Spanish province is stationary, i.e. that it takes the form of a stochastic process, with mean and variance equal to the values observed during the last 7 years.
The probability of a country c being infected at month m, P 1, was modelled using a gamma distribution with parameters αctm and β [Reference Vose20], where αctm is the probability of having at least one CSF epidemic involving type t pigs in country c during the period of time β which is the period of time considered in the assessment (β=1 month), and that has been estimated here as:

where E ctm is the number of CSF epidemics reported from country c during month m to the Office des Epizooties (OIE) [8, 9], and t ctm is the period of time (months) for which information regarding the occurrence of CSF epidemics was available at the OIE website, i.e. from 1996 to 2007, for each particular country c. Here, a CSF epidemic was defined as the occurrence of a CSF outbreak in a free country; therefore, if the index case of an epidemic in country c was reported in month m and several secondary outbreaks were reported in the following months, only month m was considered for the computation of the month when a CSF epidemic is likely to start in country c.
The probability of selecting an infected type t pig from country c at month m to be exported into Spanish province g before detection of CSF in country c, P 2, was modelled using a beta distribution with parameters α1 ctm and α2 ctm. The values of α1 ctm and of α2 ctm were computed as α1 ctm=NI ctm+1 and α2 ctm=NT ct−NI ctm+1, where NI ctm is the number of type t pigs in country c expected to be infected at month m before the detection of the epidemic, and NT ct represents the population of type t pigs in country c.
The value of NI ctm was estimated as the product of the number of outbreaks affecting type t pigs that are expected to occur before the detection of the epidemic in country c (undetected outbreaks) at month m, the average herd size of type t pigs in country c, and the expected intra-herd CSF prevalence. The expected number of undetected outbreaks affecting type t pigs in country c at month m (O ctm) was assumed to be Pert-distributed. For domestic pigs, the minimum value for the Pert distribution was assumed to be 1, which represents that the scenario of at least one outbreak, corresponding to the index case, will be undetected in country c; a probable value of six undetected outbreaks, based on the experience of Spain in 2001 [Reference Allepuz21]; and a maximum value of 39 undetected outbreaks, based on the experience of The Netherlands in 1997 [Reference Elber12]. For wild boars, values for the minimum, most likely, and maximum number of outbreaks expected to have occurred before the detection of the epidemic were assumed to be 1, 4 and 13, respectively, based on Danish estimations [22]. For domestic pigs, the average herd size of type t in country c (H ct) was computed as the ratio between NT ct and the total number of type t pig herds in country c (NH ct), whereas the value of H ct for wild boars was modelled using a Pert distribution with minimum, most likely and maximum values of 5, 20 and 35, respectively [Reference Pohlmeyer and Sodeikat23]. NT ct and NH ct were assumed to be normally distributed, with parameters μct equal to the total number of type t pigs and type t herds in country c in 2005 and σct equal to the standard deviation of the number of type t pigs and type t herds reported during the last 5 years, respectively [16, 24, Reference Artois25]. Intra-herd prevalence (P Hct) was assumed to be Pert-distributed, with a minimum value of 0·05, which is the expected intra-herd prevalence at 2 days post-infection under a scenario of low transmission rate, a most likely value of 0·4, which is the expected intra-herd prevalence that is likely to result in detection of a CSF outbreak by the herd-owner, and a maximum value of 1, which typically occurs within 2 weeks after infection [Reference Engel26]. Implicitly, the scenario of low transmission rate could also represent, for example, those cases in which partitioning or fragmentation of herds is likely to result in low risk of CSFV spread throughout the susceptible population of the herd by the time the disease is detected.
The probability that a type t pig survives CSF infection, P 3, was calculated as 1 minus the probability that a type t pig infected by CSFV dies due to the infection, Pm. The value of Pm was parameterized using a Pert distribution with minimum, most likely, and maximum values obtained from data from the CSF 2001–2002 Spanish epidemic. The minimum, most likely and maximum values were 0·068, 0·22 and 0·37, respectively (J. M. Sánchez-Vizcaíno, unpublished observations).
Because no pigs are imported for slaughtering, the probability of a type t pig reaching premises, P 4, was assumed to be equal to the probability of surviving during transportation. The probability of survival during transportation was calculated as 1 minus the probability of dying during transport, which was modelled using a Pert distribution with minimum, most likely, and maximum values of 0·0005, 0·0027 and 0·092, respectively [Reference Murray and Johnson27].
The probability of establishing an effective contact, P 5, was estimated as 1 minus the product of the probability that pigs were quarantined prior to their introduction into the premises, P q and, the probability of detection of infected animals during the quarantine, P d. The values for P q and P d were modelled using beta distributions with parameters α1 q and α2 q for P q and, α1 d and α2 d for P d, respectively. Values for α1 q, α2 q, α1 d and α2 d were 130·71, 15·41, 1·33 and 34·16, respectively, which were provided by the Head of the Epidemiology section of the Spanish Ministry of Agriculture [Reference Martínez-López18].
The model was run 10 000 times using a Monte-Carlo approach and commercially available software (@Risk version 4.5.5, Professional Edition, Palisade Corporation, Newfield, NY, USA) on Microsoft Excel (Microsoft Office Professional Edition). Results were mapped using ArcGIS 9.1 (ESRI).
The potential influence that the introduction of CSF into Spain during the 1997–1998 and 2001–2002 epidemics may have had on model outcomes was evaluated by re-running the model after removing the data corresponding to the months of April 1997 and June 2001, when the index cases of those epidemics were detected. Changes of >10% in the estimated risk of introduction were considered evidence of influence of those specific periods of time in the model results.
Sensitivity analysis
The sensitivity of the estimated values of PI to changes in the values of the input variables was quantified using a two-step procedure [19]. At the first step, the standardized regression coefficient, βi, of the relationship between the input parameter i and the probability of CSFV introduction into Spain was calculated to identify input variables most likely to influence (β⩾0·1) the model outcomes. In the second step, variables most likely to influence the outcomes of the model (β⩾0·1) were evaluated using a one-way sensitivity analysis and a balanced design. The second step was used to identify whether the estimates of β obtained from the first step may have been influenced by dependence among the input variables used to formulate the model.
For the first step of the sensitivity analysis, the value of βi was calculated as:

where b i was the regression coefficient of the association between the probability of CSFV introduction into Spain estimated using multiple linear regression analysis, and SD xi and SD yi were, respectively, the standard deviations of the input variable and the outcome estimated in 10 000 runs of the model. Estimates of β<0·1 were considered evidence of lack of sensitivity of the model to the variable.
For the second step of the sensitivity analysis a one-way sensitivity analysis was used to assess the association between variables with β⩾0·1 and the probability of CSFV introduction into Spain. The value of each variable with β⩾0·1 was varied in eight stages from minimum and maximum values equal to, respectively, a 20% reduction and a 20% increase in the base value of the variable. All other variables were kept constant on their base values. Spider graphs were used to visualize whether changes in the input variables were associated with changes in the expected values of the probability of CSFV introduction into Spain. Each line of the spider graph monitors the relationship between changes to the input variables and the model outcome. The larger the slope of the line, the greater the influence that variation of the input variable has on the outcome.
Time–space clustering of the risk of CSF introduction
Time–space clusters of Spanish provinces at high risk for CSFV introduction were identified using the normal model implementation of the time–space scan statistic [Reference Kulldorff28]. Under the normal model of the time–space scan statistic, each observation, i.e. province and month, is associated with a continuous attribute, i.e. the probability of CSFV introduction. The computation of the time–space scan statistic was based on the application of cylinders of candidate clusters throughout the study region. Base and height of the cylinders of clusters representing the area and the time period, respectively, were varied up to a maximum size equal to 50% of the population at risk and 50% of the study period, respectively. The probability of CSFV introduction within the time–space cluster was compared with the background probability estimated for Spain. The expected distribution of the probability of introduction of CSFV into Spain, under the null hypothesis of homogeneous distribution throughout the country, was computed using Monte Carlo simulation and significance (P⩽0·05) of the candidate clusters was tested using a likelihood function.
RESULTS
The annual mean probability of CSFV introduction into Spain via import of live pigs per year was 0·11 (95% PI=2·98×10−3 to 5·80×10−1), which corresponds to about one outbreak every 9 years.
The minimum probability of CSFV introduction into Spain was estimated for the province of Cuenca (mean 4·43×10−7). The highest probabilities of CSFV introduction were estimated for the provinces of Lérida (5·66×10−2), Gerona (2·33×10−2), Huesca (1·77×10−2), Barcelona (5·90×10−3), and Zaragoza (3·64×10−3). These five provinces concentrated 95·88% of the overall probability of CSFV introduction (Fig. 1).

Fig. 1. (a) Probability of introduction of classical swine fever virus (CSFV) into Spain by province via importation of live animals estimated using a risk assessment model. The probability associated with the introduction of (b) domestic pigs and (c) wild boars is indicated.
The probability of CSFV introduction through import of domestic pigs (mean=8·23×10−2, 95% PI=1·19×10−3 to 4·70×10−1) was 2·83 times higher than the probability of introduction through wild boars (mean=2·91×10−2, 95% PI=3·44×10−5 to 2·48×10−1).
The months of February, March, April and November concentrated 54·8% of the total probability of CSFV introduction into Spain (Fig. 2).

Fig. 2. Probability of introduction of classical swine fever virus (CSFV) into Spain per month via importation of domestic pigs (□) and wild boars (▪) estimated using a risk assessment model.
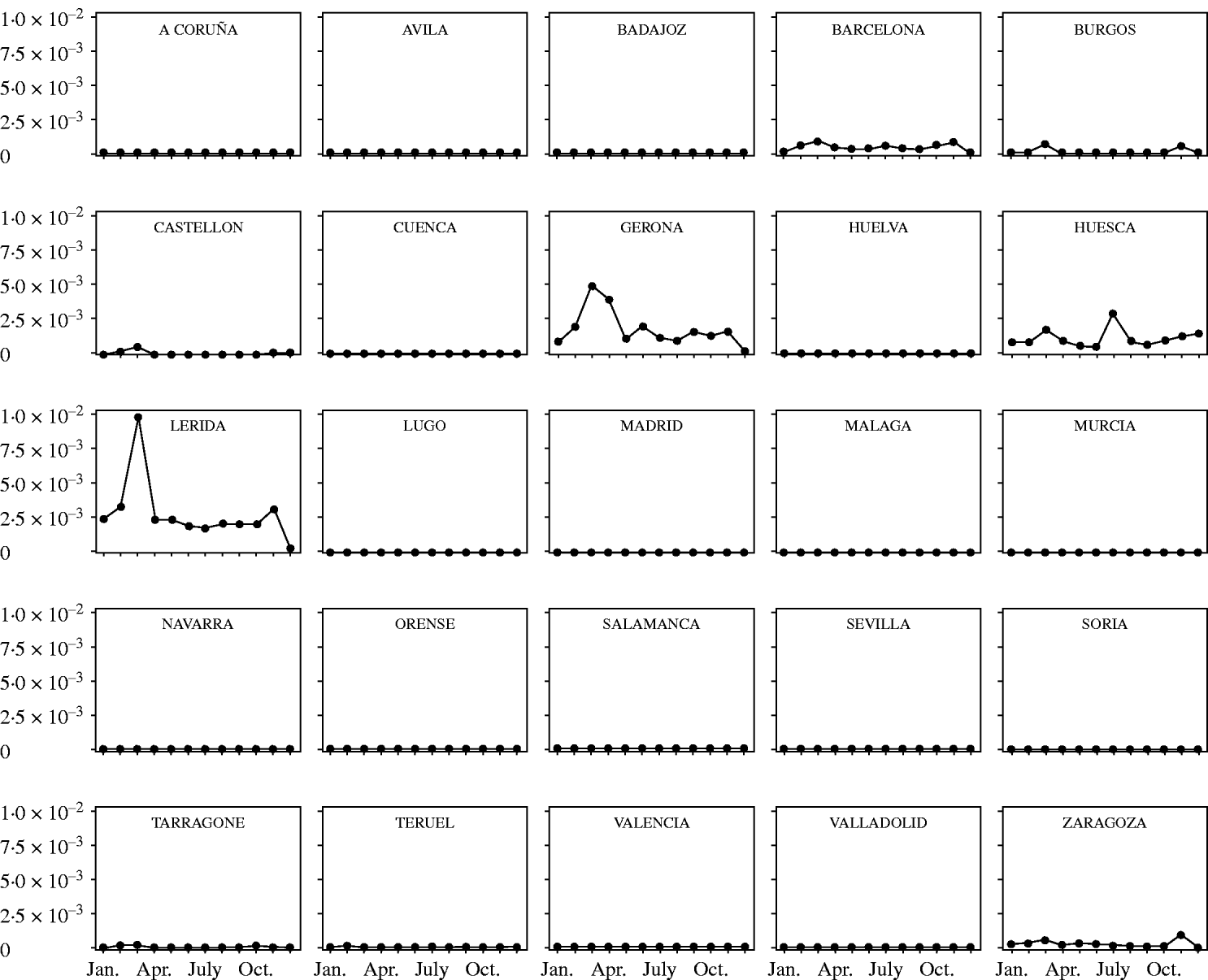
Fig. 3. Probability of introduction of classical swine fever virus (CSFV) into Spain per province and month associated with the importation of domestic pigs and estimated using a risk assessment model. Only provinces with probability >0 are represented.
The temporal and spatial variation in the risk of introduction of CSFV into Spain through import of domestic pigs and wild boars is shown in Figures 3 and 4, respectively. The time–space scan statistic estimated a significantly (P<0·01) higher risk of introducing CSFV into the province of Lérida and during the months of February and March, compared with the background risk of the country.

Fig. 4. Probability of introduction of classical swine fever virus (CSFV) into Spain per province and month associated with the importation of wild boars and estimated using a risk assessment model. Only provinces with probability >0 are represented.
The Netherlands concentrated 86·0% of the overall probability of CSFV introduction into Spain. Germany, Slovakia and Belgium accounted for 5·45%, 3·54% and 3·40% of the risk, respectively. All other countries accounted for <1% of the risk.
An alternative model was formulated without including data from April 1997 and June 2001, which are the months when CSFV was introduced into Spain. The outcomes of the model that did not include data from April 1997 and June 2001 were similar (<10% variation) to those obtained when those two months were included in the model. Therefore, we assumed that the model outcomes were not too greatly influenced by the recent history of CSF epidemics in Spain.
The multiple linear regression analysis showed that the probability of CSFV introduction into Spain was mostly affected (β⩾0·1) by changes in the probability of selecting an infected domestic pig from Austria in March (β=0·262) and in November (β=0·208) and by the probability of wild-boar infection in The Netherlands in February (β=0·209) and in March (β=0·227). However, Figure 5 showed that change in the probability of wild-boar infection in The Netherlands in February and March was the only factor influencing the probability of CSFV introduction into Spain.

Fig. 5. Graph depicting the influence that variables hypothesized as being associated (multivariate regression analysis, β⩾0·1) with the risk of introduction of classical swine fever virus (CSFV) into Spain in the model outcome (when the association was assessed using a balanced design). Variables are the probability of importing an infected domestic pig from Austria in March and November () and the probability of infection in wild boars in The Netherlands in March (•) and in February (▵).
DISCUSSION
Introduction of CSFV into Spain was estimated as being likely to occur (PI=0·11), mainly through the Northeastern provinces of the country and via the import of domestic pigs. February and March were the months with the highest contribution to the risk of CSFV entry. Results were consistent with the months and location when and where CSFV was introduced into Spain in 1997 and in 2001 [Reference Allepuz21].
The Netherlands was found to be the country imposing the highest risk for introduction of CSFV into Spain via the import of live animals. This finding is probably related to the combination of a large number of pigs annually imported into Spain and a relatively high probability of having a CSF outbreak in The Netherlands, emerging from the recent history of outbreaks in that country. The large number of pigs imported into Spain from The Netherlands is a consequence of the relatively low price that piglets realize in The Netherlands, compared with the price of piglets in Spain. Thus, pigs imported from The Netherlands are mainly young animals, which are typically introduced into Spanish premises in the absence of a previous quarantine. Germany was the country imposing the second highest risk for introduction of CSFV into Spain. CSF outbreaks were more frequently reported in Germany than in The Netherlands during the last decade, but the number of pigs imported from Germany into Spain is relatively low.
Risk of CSFV introduction into Spain was mostly affected by the probability of infection of wild boars in The Netherlands during the months of February (β=0·209) and March (β=0·227). Late winter is the season with the largest volume of wild boar movements in Spain, which can be explained, at least in part, by the beginning of the hunting season. Many of the pigs imported in winter are from The Netherlands. Based on these observations and on the results of the risk assessment, it is probable that the ability of the surveillance system to prevent or detect early the introduction of CSFV would be substantially improved by targeting imports introduced into Spain during the last part of the winter season.
To the best of our knowledge, this is the first quantitative assessment of the risk of introducing CSFV into a CSF-free country via legal importation of pigs that have been discriminated by month, by type of pig introduced (domestic, wild boars), by country of origin, and by province of destination. Horst et al. [Reference Horst29] developed Monte Carlo simulation models to quantify the risk of introducing CSF and FMD into The Netherlands. De Vos et al. [Reference de Vos30] presented a conceptual framework for the qualitative estimation of the risk of introducing CSF into Europe and Bronsvoort et al. [Reference Bronsvoort, Alban and Greiner31] developed a quantitative assessment of the likelihood of introducing CSFV into Denmark. There are, however, important differences between those earlier studies and the present study. None of the earlier studies estimated the seasonal variation in the risk of CSFV introduction. Assessment of the risk at the monthly level was considered important for Spain because trade of pigs is seasonal in nature, due to the management factors and characteristics of commercial pig production systems. Therefore, the risk of a CSF epidemic in Spain would also be expected to fluctuate seasonally, which is supported by the results of the risk assessment presented here. The Dutch study concluded that the most likely (>50% of the risk) route of CSFV introduction into The Netherlands was associated with the importation of live domestic pigs [Reference Horst29]. This finding is consistent with the decision of focusing our risk assessment on the introduction of live animals into Spain. The Danish study [Reference Bronsvoort, Alban and Greiner31], however, found that returning livestock trucks and legal meat imports imposed the highest risk of introducing CSFV into Denmark. The Danish scenario considerably differs from the Spanish situation because a voluntary 30-day quarantine of pigs is systematically implemented for imported animals, and because the number of domestic live animals imported annually into Denmark is much lower (1280 pigs) compared with Spain (1·2 million animals). Interestingly, the Danish study estimated that an abandonment of the voluntary 30-day quarantine of pigs would result in a median of one CSF outbreak every 9 years, which is similar to the results presented here for Spain. Moreover, and similar to our study, The Netherlands and Germany were the two countries imposing the highest risk of introduction of CSFV into Denmark.
None of those earlier assessments of the risk of CSFV being introduced into European countries considered the risk associated with the introduction of wild boars. Differences in the methodological approach were probably due to a combination of negligible (or absent) number of wild boars imported into The Netherlands and Denmark and to differences in the characteristics of the wild-boar production system in those two countries, compared to those observed in Spain. Although the risk of CSFV introduction via wild boars into Spain was estimated to be almost three times lower than via domestic pigs, introduction via wild boars may result in more severe consequences for the Spanish pig industry than introduction via domestic pigs. Because clinical signs of the disease in wild boars are typically milder than in domestic pigs and because wild boars are less frequently observed by farmers than domestic pigs, one would expect that the time to diagnosis of a CSF case would be longer in wild boars than in domestic pigs. Thus, by the time the disease is diagnosed in wild boars, it is possible that infection would be widely spread throughout the susceptible population of the country. Moreover, because of the demographic characteristics of the operations where wild boars are introduced, infected animals would probably impose a high risk of transmission to Iberian pigs, which are a major source of income for the Spanish agricultural industry. Finally, logistic and technical considerations would result in more difficult control of a CSF epidemic in wild boars compared with an epidemic in domestic pigs. In light of the severe consequences that introduction of CSFV via wild boars may have for Spain, the threefold lower risk estimated for wild boars compared with domestic pigs might not be as low as first thought when analysing the results presented here. Therefore, the contingency plan in the event of a CSF epidemic in Spain should consider the particular aspects related to the wild boar production system in the country in order to anticipate and prevent the potential impact of the disease.
Certain assumptions and limitations of the study may have influenced the model results. The model relies on the accuracy of the sources of information used to parameterize the model. The probability of detection of infected animals during quarantine was based on expert opinion that probably refers to general estimates applicable to the most common infectious diseases of pigs, such as FMD or CSF, rather than CSF specifically. This limitation is reflected on the wide range of the parameter distributions. However, it is of note that the parameter has not influenced the model outcomes, as indicated by the results of the sensitivity analysis. Quality of information obtained by international agencies, such as OIE or FAO, depends on the willingness and capability of countries to collect and share the information. For example, failure of countries to report CSF outbreaks may have resulted in an underestimation of the risk of introduction of CSFV into Spain. Risk for disease spread within the EU is probably associated with the silent phase of an epidemic, i.e. before the infected country detects the disease and bans animal movements into other countries. The silent phase of a CSF epidemic was assumed to last for about 1 month; therefore, the risk of introducing CSFV into Spain via animal movements was only computed for such period of time. The probability of infection at the origin was computed at the country level, leading to the assumption of homogenous spatial distribution of the probability within a given country. Consideration of spatial variation of risk within the countries of origin would have required models for individual countries within the EU in order to quantify the intra-country risk of spread during the silent phase of the epidemic, as well as information on the geographical origin of pig shipment from each exporting country. This alternative formulation would have resulted in an extremely complex model for which quality data were not available to us. Another alternative formulation of the model would have been consideration of the risk associated with shipments of pigs imported into Spain, rather than a formulation based on the number of animals shipped. We failed to identify reasons why this alternative parameterization would have led to results different than those presented here and for that reason, the model has been simplified following the principle of parsimony. In general, we believe that the assumptions and limitations did not result in biased estimates of the geographical variation of risk of CSFV introduction into Spain.
CONCLUSION
If prevailing conditions persist, Spain is at a relatively high risk for the introduction of CSFV via importation of live animals and the virus is most likely to be introduced into the country in late winter, into Catalonian operations, and via the importation of domestic pigs. Surveillance and prevention activities should be selectively targeted to those specific areas, species, and periods of time. A finding of note, however, was that the risk associated with the introduction of the virus via the importation of wild boars is not nil in Spain. Because of the particular implications that a CSF epidemic could have for wild boars in the country, it is recommended that Spain increases its surveillance and biosecurity measures, such as quarantine, intended for the early diagnosis and prevention of the introduction and spread of the virus in this species. The methodological approach and conclusions presented here will be useful for helping Spain to develop policies and to allocate resources in areas and periods of time at the highest risk of CSFV introduction and will ultimately help to prevent or mitigate the consequences of a CSFV incursion into the country.
ACKNOWLEDGEMENTS
The project was funded in part by the Spanish Ministry of Education and Science (MEC), the Spanish Organization of Agricultural Insurances (ENESA), the Spanish Ministry of Agriculture, Fisheries and Food (MAPA), and the U.S. National Center for Medical Intelligence.
DECLARATION OF INTEREST
None.