Whole milk powder (WMP) contains 26 to 40% (w/w) fat, and is generally manufactured from raw milk and processed by pasteurisation, concentration, evaporation and spray-drying. WMP is an important ingredient for a wide range of food products as it can be reconstituted to produce milk drinks, infant milk formula, yoghurts, milk chocolate and ice cream, amongst others (USDEC, 2005).
WMP sensory characteristics can be influenced by animal diet, heat treatment and other processing and storage conditions (water activity, moisture, packaging, light and temperature: Baldwin et al., Reference Baldwin, Cooper and Palmer1991; Birchal et al., Reference Birchal, Passos, Wildhagen and Mujumdar2005; Faulkner et al., Reference Faulkner, O'Callaghan, McAuliffe, Hennessy, Stanton, O'Sullivan, Kerry and Kilcawley2018; Clarke et al., Reference Clarke, O'Sullivan, Kerry and Kilcawley2020a). Pasture-based farming systems are widely practiced in Ireland for the majority of lactation, allowing for the creation of a low-cost, animal welfare friendly, natural feed source to produce high-quality milk products, which are considered more organic and healthier by consumers (Whelan et al., Reference Whelan, Carey, Boland, Lynch, Kelly, Rajauria and Pierce2017). However, feeding concentrates, such as total mixed ration (TMR) and housing cows indoors is widely implemented in most developed countries mainly for economic reasons (Haskell et al., Reference Haskell, Rennie, Bowell, Bell and Lawrence2006). Numerous studies have explored the composition of milk from different breeds and feeding systems which have demonstrated that pasture-derived milks have higher levels of polyunsaturated fatty acids (PUFA) and conjugated linoleic acid (CLA) and lower levels of saturated fatty acids compared with those derived from TMR diets (O'Callaghan et al., Reference O'Callaghan, Mannion, Apopei, McCarthy, Hogan, Kilcawley and Egan2019; Kalač and Samková, Reference Kalač and Samková2010). A diet of fresh pasture significantly increases levels of β-carotene, enhancing a yellow colour, particularly obvious in butter products derived from pasture, but also apparent in milk and milk powders. Some studies have shown that volatiles generated indirectly through rumen metabolism from forage can also have a sensory impact on milk. For example, p-cresol probably contributes to the ‘barn-yard’ aroma/flavour of cow milk derived from pasture (Faulkner et al., Reference Faulkner, O'Callaghan, McAuliffe, Hennessy, Stanton, O'Sullivan, Kerry and Kilcawley2018; Kilcawley et al., Reference Kilcawley, Faulkner, Clarke, O'Sullivan and Kerry2018) and it is likely that some volatiles are directly transferred from diet (Villeneuve et al., Reference Villeneuve, Lebeuf, Gervais, Tremblay, Vuillemard, Fortin and Chouinard2013).
Thermal processing conditions can also alter the volatile profile of milk products. For example, increases of aldehydes and methyl ketones derived from lipid oxidation (decarboxylation or light induced oxidation) of β-keto acids after heat treatment (Vazquez-landaverde et al., Reference Vazquez-Landaverde, Velazquez, Torres and Qian2006; Hougaard et al., Reference Hougaard, Vestergaard, Varming, Bredie and Ipsen2011), sulphur compounds formed by oxidation of methanethiol (Contarini et al., Reference Contarini, Povolo, Leardi and Toppino1997), esters from heat-catalysed esterification reactions (Faulkner et al., Reference Faulkner, O'Callaghan, McAuliffe, Hennessy, Stanton, O'Sullivan, Kerry and Kilcawley2018), increases in γ-lactones derived from hydroxy fatty acids after heat processing (Yoshinaga et al., Reference Yoshinaga, Tago, Yoshinaga-Kiriake, Nagal, Yoshida and Gotoh2019), increases in ketones formed by β-oxidation of saturated fatty acids (Li et al., Reference Li, Zhang and Wang2012), Maillard reaction products such as benzaldehyde, furans, maltol, acetaldehyde, 3-methylbutanal, 2-methylbutanal, 2-methylpropanal and possibly acetophenone (Calvo and de la Hoz, Reference Calvo and de la Hoz1992) and the degradation of β-carotene resulting in the formation of toluene and xylenes (Zepka et al., Reference Zepka, Garruti, Sampaio, Mercadante and Silva2014). Several ketones, aldehydes and sulphur compounds have been reported to increase during ultra-high temperature treatment and sterilisation of milk (Soukoulis et al., Reference Soukoulis, Panagiotidis, Koureli and Tzia2007; Al-Attabi et al., Reference Al-Attabi, D'Arcy and Deeth2009; Zabbia et al., Reference Zabbia, Buys and Kock2012). Many of these VOCs can be perceived as off-flavours and can be problematic in products such as WMP, and potentially even carry through into the final product applications resulting in consumer complaints (Hough et al., Reference Hough, Sánchez, de Pablo, Sánchez, Villaplana, Giménez and Gámbaro2002). Storage time was also shown to have an effect on losses of VOCs in milk powders and this could be due to metabolic and enzymatic reactions post pasteurisation, or chemical reactions (Contarini et al., Reference Contarini, Povolo, Leardi and Toppino1997; Clarke et al., Reference Clarke, Griffin, Rai, O'Callaghan, O'Sullivan, Kerry and Kilcawley2020b).
Therefore, VOC and sensory profiling of milk and milk products, especially powders such as WMP, is necessary for quality and shelf life purposes. Sensory profiling when undertaken in association with VOC analysis can provide useful additional information in relation to the association between VOCs and sensory properties. VOC profiling is predominately undertaken using gas chromatography mass spectrometry (GC-MS) and recent studies have shown the importance of extraction method choice in relation to VOC profiling of WMP (Cheng et al., Reference Cheng, Mannion, O'Sullivan, Miao, Kerry and Kilcawley2021) and the impact of diet on the oxidative and sensory shelf life of WMP (Clarke et al., Reference Clarke, Griffin, Rai, Hennessy, O'Callaghan, O'Sullivan, Kerry and Kilcawley2021). Three distinct VOC extraction techniques were utilised in this study in order to obtain a more complete VOC profile; headspace solid phase micro-extraction (HS-SPME), thermal desorption (TD) and a high capacity sorptive extraction (Hi-Sorb) procedure by direct immersion (DI). The aim of this study was to investigate the effect of three distinct diets (GRS, CLV and TMR) on the sensory and VOC profile of WMP.
Materials and methods
Whole milk powder manufacture
Raw milk from 54 Friesian cows was split into 3 groups (n = 18) at the Teagasc Moorepark dairy farm, Teagasc, Animal & Grassland Research Centre, Fermoy, Co. Cork, Ireland. Each group of 18 cows were given separate diets; outdoor pasture grazing on perennial ryegrass (Lolium perenne) denoted as GRS, outdoor pasture grazing on perennial ryegrass supplemented with white clover (Trifolium repens) denoted as CLV, and indoors on total mixed rations (TMR) consisting of grass silage, maize silage and concentrates, which was a replication of the study outlined in detail by O'Callaghan et al. (Reference O'Callaghan, Faulkner, McAuliffe, O'Sullivan, Hennessy, Dillon, Kilcawley, Stanton and Ross2016). Raw whole milk (approximately 1000 kg) was collected from cows on each dietary treatment. The preparation of the WMP was as outlined in Cheng et al. (Reference Cheng, Mannion, O'Sullivan, Miao, Kerry and Kilcawley2021). The milk was not standardised, but the average fat contents of the milk from each diet in triplicate were quite similar (GRS 27.9%, CLV 28.2%, TMR 28.2%). WMP samples were stored at room temperature in sealed 900 g aluminium vacuum cans until analysis (all analysis was undertaken within the designated shelf life of the samples).
Sample preparation
Prior to sensory and VOC analysis WMP from each dietary treatment (GRS, CLV and TMR) at <2 months was dissolved at 10% solids (w/v) using ultra-pure deionised water and stored at 4°C overnight to ensure solubility without overhead lights to prevent light-induced off-flavour formation as outlined in Cheng et al. (Reference Cheng, O'Sullivan, Kerry, Drake, Miao, Kaibo and Kilcawley2020) in the preparation of SMP for sensory analysis. VOC analysis and sensory analysis were performed the following day.
To monitor the performance of each extraction procedure, an internal standard (IS) of 2-phenyl-D5-ethanol and 4-methyl-2-pentanol (Merck., Arklow, Wicklow, Ireland) at 20 mg/l in ultra-pure water, was added (50 μl) to each WMP sample prior to extraction.
Volatile organic compound extraction
The extraction procedures for HS-SPME, TD and DI-HiSorb were identical to those described by Cheng et al. (Reference Cheng, Mannion, O'Sullivan, Miao, Kerry and Kilcawley2021).
GC–MS analysis
The GC–MS system was an Agilent 7090A GC and Agilent 5977B MSD (Agilent Technologies Ltd., Cork, Ireland) using a non-polar column DB5 (60 m × 0.25 mm × 0.25 μm) (Agilent Technologies Ltd., Ireland). The column oven was held at 40°C for 5 min, then increased to 230°C at 5°C/min and held at 230°C for 35 min, yielding a total run time of 60 min. The carrier gas was helium held at a constant flow of 1.2 ml/min. The ion source temperature was 220°C and the interface temperature was set at 260°C. The mass spectrometer was in electronic ionisation (70 V) mode with the mass range scanned between 35 and 250 amu. Analysis was undertaken using Mass Hunter Qualitative Analysis Software (Agilent Technologies Ltd) with target and qualifier ions and linear retention indices for each compound compared an in-house library based on mass spectra obtained from NIST 2014 mass spectral library MS searching (v.2.3, Gaithersburg, MD, USA), and an in-house library created using authentic compounds with target and qualifier ions and linear retention indices for each compound using Kovats index. Spectral deconvolution was also performed to confirm identification of compounds using Automated Mass spectral Deconvolution and Identification System (AMDIS). Batch processing of samples was carried out using MetaMS (Wehrens et al., Reference Wehrens, Weingart and Mattivi2014). MetaMS is an open-source pipeline for GC–MS-based untargeted metabolomics. The peak areas of the analytes were normalised to the peak areas of the IS (4-methyl-2-penanol) at first and then expressed as a percentage of the total.
To monitor the performance of the GC-MS operating conditions, an external standard (ES) solution was added at the start and end of each GC-MS sample run, the peak areas were monitored to ensure they were within a specified tolerance (10% coefficient of variation) to ensure that both the extraction and MS detection were performing within specification during the analysis. The ES was comprised of 1-butanol, dimethyl disulphide, butyl acetate, cyclohexanone, and benzaldehyde (Merck, Ireland) at 10 mg/l with 2-phenyl-D5-ethanol (Merck, Arklow, Co., Wicklow, Ireland) added at 5 mg/l in ultra-pure water. For the HS-SPME technique, 10 μl of ES was added to the sample in a 20 ml amber HS- SPME vial (Apex Scientific Ltd., Maynooth, Ireland). For TD and DI-HiSorb, the ES (10 μl) was added to the TD tube containing the sample extract for both TD and DI-HiSorb extracts.
Sensory analyses
All sensory analyses was undertaken at the sensory facility within the School of Food and Nutritional Science, University College Cork, Co. Cork, Ireland, according to International Standards (ISO 11136, 2014).
Milk consumers residing in Cork (Ireland, n = 99) (70 : 30 male/female, age 18–50 years), participated in the consumer test (hedonic attribute testing). The consumers consisted of students and staff from Sensory Group, School of Food and Nutritional Science, University College Cork, Co. Cork, Ireland. Consumers were regular self-reported consumers of milk, had experiences in drinking powdered milk products, and were non-rejecters of milk. Participants used the sensory hedonic descriptors (Table 1) provided to them for three different WMP samples (CLV, GRS and TMR) presented in triplicate at 10% solids (w/v). For consumer testing, samples were dispensed into 30 ml inert plastic tumblers provided with three digit codes presented simultaneously but with randomised order to prevent first order and carry-over effects (Macfie et al., Reference Macfie, Bratchell, Greenhoff and Vallis1989). Samples were taken from the refrigerator (4°C) and served after 15 min at 12°C temperature. Participants were first asked to evaluate the overall appearance and colour of the sample. The appearance of each sample was scaled using a 1–9 hedonic scale, where 1 = dislike extremely and 9 = like extremely. They were then asked to taste the sample and evaluate their overall impression using a 9-point hedonic scale. The assessors were asked to assess using a 9-point hedonic scale the liking of flavour, freshness, liking of appearance, liking of aroma, overall acceptability, cooked flavour, thickness, creaminess, aftertaste, intensity of aftertaste and quality.
Table 1. Sensory terms for the affective (consumer acceptance testing) and optimised descriptive profiling (ODP) of whole milk powder

Optimised descriptive profiling (ODP: da Silva et al., Reference da Silva, Minim, Simiqueli, Moraes, Gomide and Minim2012, Reference da Silva, Minim, Carneiro, Nascimento, Della Lucia and Minim2013) was undertaken with trained panellists at University College Cork, Ireland (n = 33). These assessors were presented with all samples simultaneously, but with randomised order to prevent first order and carry-over effects (Macfie et al., Reference Macfie, Bratchell, Greenhoff and Vallis1989). Assessors used the consensus list of sensory descriptors as described by Cheng et al. (Reference Cheng, O'Sullivan, Kerry, Drake, Miao, Kaibo and Kilcawley2020) which were measured on a 10 cm line scale with the term ‘none’ used as the anchor point for the 0 cm end of the scale and ‘extreme’ for the 10 cm end of the scale (Table 1). Sensory terms, which were the main sensory dimensions, were pre-selected from the sample set using an expert sensory panel (n = 10). Assessors evaluated the intensity of each attribute for each sample on the scales. Attributes were presented along with the table describing the sensory terms (Table 1). All samples were prepared in the same manner as the consumer analysis study and presented in duplicate.
Statistical analysis
Statistical analyses for data relating to volatile analysis were carried out using one way-ANOVA. The level of significance for correlation was set at P < 0.05. To classify WMP samples in a supervised multivariate model, partial least-squares discriminant analysis (PLS-DA) was performed. Unsupervised hierarchical clustering analysis (HCA) was used to show the patterns in the VOC profile and is presented as a heatmap (Online Supplementary Fig. S1). Consumer acceptance data obtained from sensory analysis was evaluated by one-way ANOVA using differences of perception related to diet as the primary factors. Analyses were carried out at only one time point and where normally distributed were analysed using one-way ANOVA with post hoc. The SPSS V23.0 (IBM Statistics Inc., Armonk, NY) was used for one-way ANOVA. Principal component analysis (PCA) plots of the volatile and diet data were used to demonstrate correlations between the VOC and the different diet attributes. These were constructed using the ‘factoextra’ and ‘FactoMinoR’ packages in R (v 3.4.1). The Unscrambler X software, version 10.3 (Camo Software, Oslo, Norway) was used for ANOVA-PSLR (APLSR) analysis of WMP data from different diets and variance of OPD sensory data. Analysis of variance (ANOVA) with post hoc Tukey significant test was applied to OPD data, working at an alpha level of 0.05. The correlations between sensory attributes and VOC were also analysed by PLSR. PLSR were performed with VOC data as the X-matrix and sensory attributes as the Y-matrix. Regression coefficients were analysed by Jack-knifing to derive significance indicators for the relationships determined in the quantitative APLSR (data not shown). Metabolic Analyst 4.0 (McGill University, Montreal, QC, Canada) software was used to perform the HCA graph (Chong et al., Reference Chong, Soufan, Li, Caraus, Li, Bourque, Wishart and Xia2018).
Results
Volatile analysis
The volatile compounds identified in the WMP from the three different diets (GRS, CLV and TMR), using three extraction methods are listed in Table 2. Results are expressed as percentage abundance of each VOC per extraction method based on the recovery in relation to the recovery of the IS for that extraction method. A total of 64 VOCs were identified in these WMP derived from all three diets by HS-SPME, TD and DI-HiSorb. Distinct differences in the VOCs profiles were evident between the different extraction techniques, which highlights the effectiveness of each extraction technique for particular chemical classes. The same trends in relation to individual VOCs were evident across the different extraction techniques where a VOC was identified by one or more extraction technique. Eight lactones, 5 aldehydes, 3 ketones, 3 terpenes, 2 alcohols, 2 esters, 1 acid, 1 hydrocarbon and 1 sulphur compound varied significantly (P < 0.05 or P < 0.01) in the WMP based on cow diet (Table 2).
Table 2. The volatile compounds in the whole milk powders derived from different diets, grass (GRS), grass/clover (CLV) and total mixed ration (TMR) by GCMS from three volatile extraction procedures
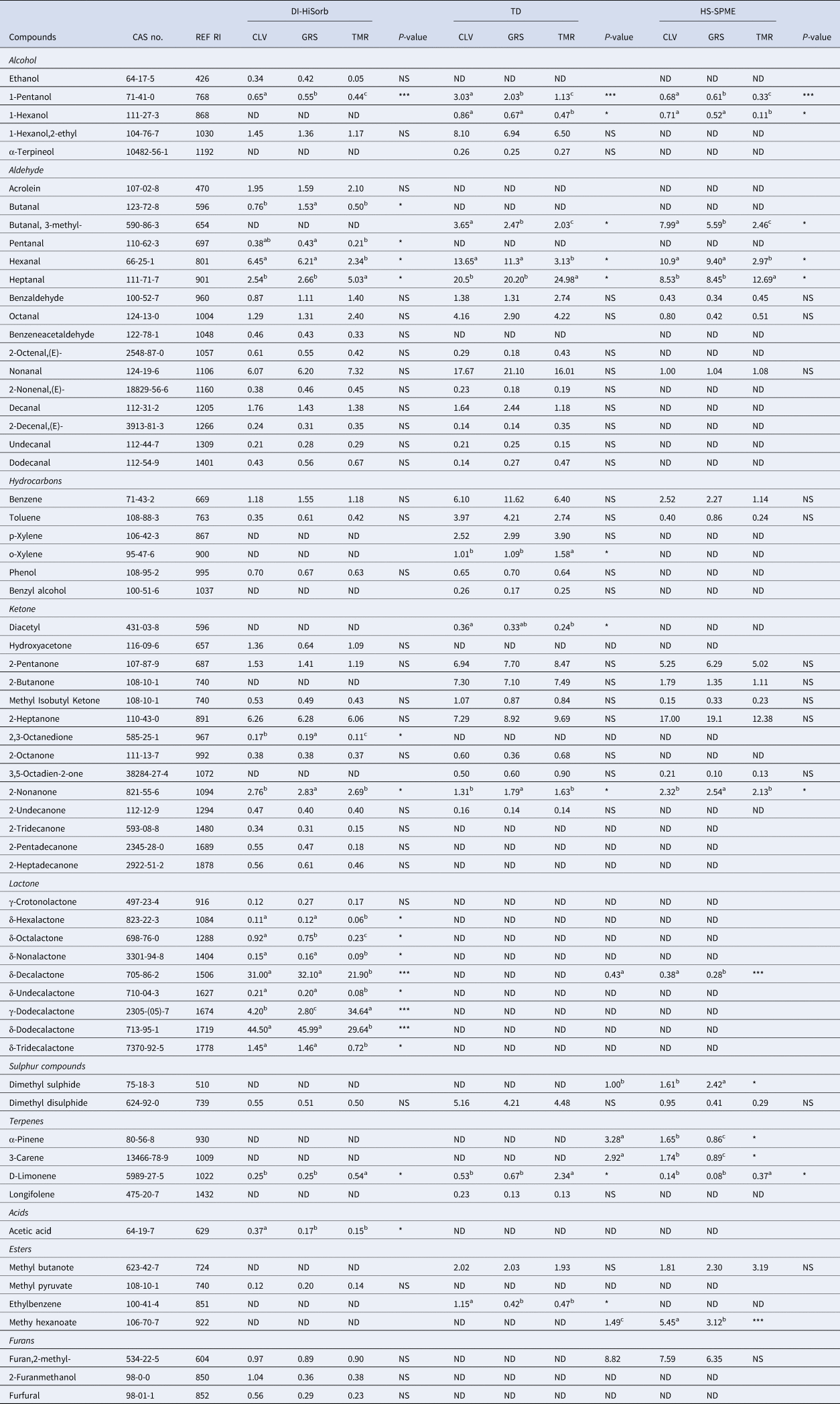
RI: Retention index. REF RI: Reference retention index. CAS no: Chemical Abstracts Service Number. One-way ANOVA statistical analysis :* and *** denote significant differences at P < 0.05 and P < 0.01, respectively. Values in the same row not sharing the same superscript (a,b,c) specify significant difference in peak area % value carried out by Tukey post hoc test.
Results are expressed as percentage abundances for each extraction technique based on the recovery of the internal standard (2-phenyl-D5-ethanol).
Seven VOCs were significantly more abundant in WMP derived from CLV; 1-pentanol (P < 0.05) and 3-methyl-butanal, δ-octalactone, α-pinene, 3-carene, acetic acid and ethyl-benene (P < 0.01). Four VOCs were significantly more abundant in the WMP derived from GRS; methyl-hexanoate (P < 0.05) and butanal, 2,3-octanedione and 2-nonanone (P < 0.01). Five VOCs were significantly more abundant in the WMP derived from TMR; γ-dodecalactone GRS (P < 0.05) and dimethyl sulphide, D-limonene, heptanal and ο-xylene (P < 0.01). Another eight VOCs were significantly more abundant in WMP derived from both CLV and GRS in comparison to WMP derived from TMR; δ-decalactone and δ-dodecalactone (P < 0.05) and δ-tridecalactone, hexanol, hexanal, δ-hexalactone, δ-nonalactone and δ-undecalactone (P < 0.01). Another VOC, pentanal, was significantly (P < 0.01) more abundant in WMP derived from GRS in comparison to WMP from TMR, but was not statistically different to WMP derived from CLV (nor was there any statistical difference between WMP from CLV or TMR). Similarly diacetyl was significantly higher (P < 0.01) in WMP derived from CLV than from WMP derived from TMR, but was not statistically different to WMP from GRS (WMP from GRS and from TMR were also not statistically different). These associations are more clearly represented in the PCA plots (Fig. 1a and b) and by the HCA heatmap (online Supplementary Fig. S1), where it is evident that both the WMP derived from GRS and CLV diets are more comparable, but distinctly separate from WMP derived from TMR diets (Fig. 1a and b).
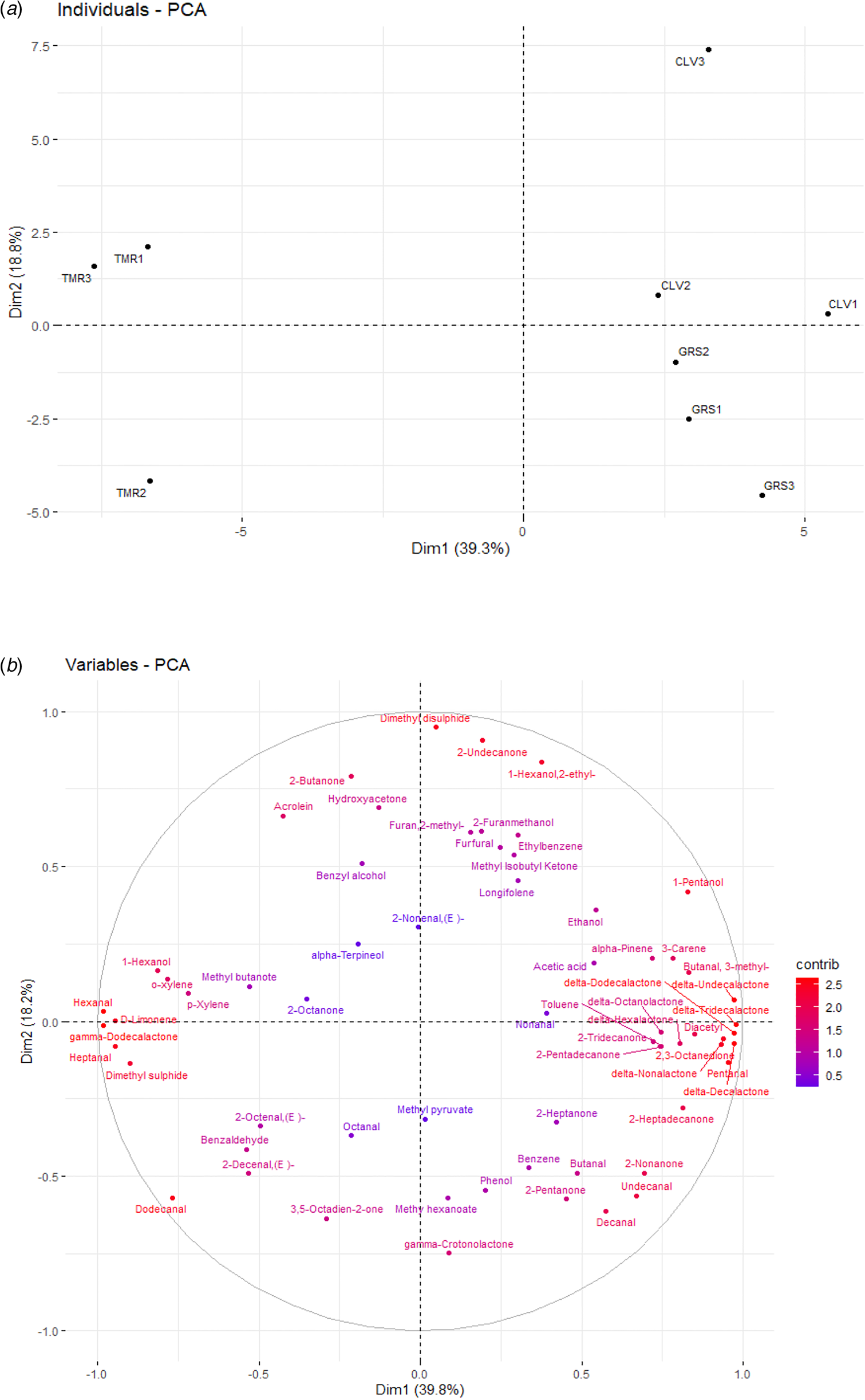
Fig. 1. Principal component analysis: (a) Three kinds of feeding system of grass (GRS), grass/clover (CLV) and total mixed rations (TMR). Scores and loadings are based on the average of three repetitions for each feeding diets. (b) Variables: the relative percent amount of 64 volatile compounds. Colour gradient: low = white, mid = blue and high = red, midpoint set 1.0.
Sensory evaluation
The sensory attributes identified in the WMP derived from CLV, GRS and TMR diets by consumer acceptance are shown in Fig. 2. Five significant sensory differences (P < 0.05) were observed between the WMP derived from these diets; creaminess, aftertaste, cooked-flavour, quality and liking of flavour. The WMP derived from CLV diets scored statistically (P < 0.05) highest for liking of flavour, creaminess and quality. The WMP derived from TMR diets scored significantly highest (P < 0.05) for cooked-flavour and aftertaste. The WMP derived from GRS diets did not score significantly different for any of the sensory attributes in comparison to WMP samples derived from either CLV or TMR diets.

Fig. 2. Hedonic sensory analysis of whole milk powder drink derived from different feeding systems of grass (GRS), grass/clover (CLV) and total mixed rations (TMR). The whole milk powder samples were assessed by consumers (n = 99) familiar with milk using blind replicates in a full balanced block design, where consumers evaluated all samples in duplicate. The error bars represent standard mean error within replicates. Columns with different letters (a–c) for each attribute are statistically different (P < 0.05) carried out by ANOVA and Tukey post hoc test.
The ODP evaluation of WMP from different diets is shown in the PCA plot (online Supplementary Fig. S2). The significance (P-value) of regression coefficients and average results (ANOVA) for the ODP attributes for WMP from different diets (CLV, GRS and TMR) are illustrated in Table 3. The assessors rated WMP derived from CLV diets as significantly greater for liking of colour (P < 0.05) and creamy aroma (P < 0.05) in comparison to the WMP-derived TMR diets. The WMP derived from GRS diets scored statistically (P < 0.01) higher for cooked aroma and cooked flavour and WMP derived from TMR diets highest for caramelised flavour (P < 0.05), sweet aroma and sweet taste (P < 0.01).
Table 3. ODP (Optimised Descriptive Profiling) evaluation by assessors of whole milk powder produced by different diets, grass (GRS), grass/clover (CLV) and total mixed rations (TMR)

ANOVA values are the average results. One-way ANOVA statistical analysis :* and *** denote significant differences at P < 0.05 and P < 0.01, respectively. Values in the same row not sharing the same superscript (a, b,c) specify significant difference in sensory attribute value carried out by Tukey post hoc test.
Correlation relationships and ANOVA analysis with sensory attributes observed in whole milk powder. Positive and negative values indicate positive and negative correlations between the diets and sensory attributes, respectively.
OPD sensory and volatile data correlations
APLSR was conducted to study the relationships of the individual VOCs with the sensory descriptors as used in the ODP study. The X-matrix was composed of 64 VOCs, whereas the Y-matrix consisted of the sensory attributes from OPD (Fig. 3). When the two PLSs were taken into account, 63% of the VOCs explained 36% of the variation among the sensory data and diets. All variances were placed between the inner (r 2 = 0.5) and outer ellipses (r 2 = 1.0), thereby indicating that the APLSR model sufficiently described the associations between descriptors and VOCs. The centre ellipsoid in Fig. 3 indicates 50% of the explained variation. Many VOC were located inside the ellipsoid, which means they did not greatly contribute to the model. Figure 3 demonstrates that the WMP samples are separated along PLS1, with the WMP derived from TMR on the right side and the WMP derived from GRS and CLV on the left side. This highlights again that WMP derived from TMR diets is more distinct than the WMP from both the CLV and GRS diets.

Fig. 3. ANOVA-PLSR correlation loadings plot of sensory attributes (aroma and flavour) by OPD analysis and volatile compounds (X-matrix) in the WMP from the three distinct diets, grass (GRS), grass/clover (CLV) and total mixed rations (TMR). Ellipses represent r2 = 0.5 and 1.0, respectively.
Discussion
The source of many of these VOCs in WMP is varied and probably a combination of some or all of the following; direct transfer from the diet, rumen metabolism, metabolism in the raw milk, created during heat processing or by auto chemical reactions. However, some trends were evident. Seventeen of the twenty six VOCs that were significantly different based on diet in these WMP samples are derived from fatty acids either by lipid oxidation, thermal degradation or β-oxidation and lactonisation (butanal, pentanal, hexanal, hexanal, 1-pentanol, 1-hexanol, 2-nonanone, 2,3-octanedione, δ-hexalactone, δ-octanolactone, δ-nonalactone, δ-decalactone, δ-undecalactone, δ-dodecalactone, δ-tridecalactone, γ-dodecalactone). It is well established that different diets have a significant impact on the fatty acid profile of cow's milk (Kalač and Samková, Reference Kalač and Samková2010; O'Callaghan et al., Reference O'Callaghan, Faulkner, McAuliffe, O'Sullivan, Hennessy, Dillon, Kilcawley, Stanton and Ross2016), hence it is logical that VOCs derived from fatty acids are also likely to be significantly impacted. Such differences may be further exacerbated by subsequent thermal treatment during processing to WMP enhancing lipid oxidation and Maillard and lactonisation reactions (Calvo and de la Hoz, Reference Calvo and de la Hoz1992; Havemose et al., Reference Havemose, Weisbjerg, Bredie, Poulsen and Nielsen2006; Kilcawley et al., Reference Kilcawley, Faulkner, Clarke, O'Sullivan and Kerry2018; Clarke et al., Reference Clarke, Griffin, Rai, Hennessy, O'Callaghan, O'Sullivan, Kerry and Kilcawley2021).
Straight-chain aldehydes are major contributors to off-flavours in dairy products (Barrefors et al., Reference Barrefors, Granelli, Appelqvist and Bjoerck1995). Previous studies have also found that the abundance of methyl ketones also from lipid oxidation were correlated to the severity of heat treatment in milk and associated with off-flavour development that can be carried over to final product applications (Nursten, Reference Nursten1997). Only one methyl ketone, 2-nonanone was significantly different based on diet, and most abundant in WMP derived from GRS. The ketone 2,3-octanedione, which is also a product of lipid oxidation, has previously been shown to be higher in milk derived from pasture (Coppa et al., Reference Coppa, Martin, Pradel, Leotta, Priolo and Vasta2011) as found in this study. Two primary alcohols, 1-pentanol and 1-hexanol are also products of lipid oxidation. 1-Pentanol is derived from the primary aldehyde pentanal and was significantly correlated with the WMP from CLV diets, in agreement with previous studies on milk (Faulkner et al., Reference Faulkner, O'Callaghan, McAuliffe, Hennessy, Stanton, O'Sullivan, Kerry and Kilcawley2018; Clarke et al., Reference Clarke, O'Sullivan, Kerry and Kilcawley2020a). Villeneuve et al. (Reference Villeneuve, Lebeuf, Gervais, Tremblay, Vuillemard, Fortin and Chouinard2013) found levels of 1-pentanol were significantly higher in milk derived from pasture and silage than from hay, however, other studies have not found an impact of diet on levels of 1-pentanol (Coppa et al., Reference Coppa, Martin, Pradel, Leotta, Priolo and Vasta2011). 1-Hexanol, derived from hexanal (Zhang et al., Reference Zhang, Ai, Wang, Tong, Zheng and Sun2016) was significantly higher in the WMP derived from TMR diets, in agreement with that found by Faulkner et al. (Reference Faulkner, O'Callaghan, McAuliffe, Hennessy, Stanton, O'Sullivan, Kerry and Kilcawley2018) and Clarke et al. (Reference Clarke, Griffin, Rai, O'Callaghan, O'Sullivan, Kerry and Kilcawley2020b) in milk, but other studies did not find an impact of diet on levels of 1-hexanol in milk (Villeneuve et al., Reference Villeneuve, Lebeuf, Gervais, Tremblay, Vuillemard, Fortin and Chouinard2013). Esters are probably present as a result of microbial activity in the rumen, post-pasteurisation microbial contamination or heat-catalysed esterification during thermal-treatment (Manzocchi et al., Reference Manzocchi, Martin, Bord, Verdier-Metz, Bouchon, Marchi, Constant, Giller, Kreuzer, Berard, Musci and Coppa2021; Vazquez-Landaverde et al., Reference Vazquez-Landaverde, Velazquez, Torres and Qian2006). Only two fatty acid esters were found in these WMP samples and only methyl hexanoate varied with diet. Methyl hexanoate was found to be significantly more abundant in WMP derived from GRS diets.
The significant difference in the abundance and presence of lactones in the WMP derived from these diets is especially interesting due to their potential positive sensory impact (the abundance of 8 of the 9 lactones identified varied with diet). Lactones are cyclic compounds, formed through metabolism and/ or thermal degradation of δ- and γ-hydroxyacids and are known to contribute to sweet, creamy and fatty flavours in milk powders (Yoshinaga et al., Reference Yoshinaga, Tago, Yoshinaga-Kiriake, Nagal, Yoshida and Gotoh2019; Ianni et al., Reference Ianni, Bennato, Martino, Grotta and Martino2020). The recovery of lactones was mostly achieved using the DI-HiSorb extraction method, which is a sorptive extraction technique particularly useful for extracting lactones in contrast to other extraction methods (High et al., Reference High, Bremer, Kebede and Eyres2019; Cheng et al., Reference Cheng, Mannion, O'Sullivan, Miao, Kerry and Kilcawley2021). Some studies have stated that milk from cows fed hay (timothy) was characterised by higher contents of γ-lactones than silage-fed and pasture-fed derived milk (Villeneuve et al., Reference Villeneuve, Lebeuf, Gervais, Tremblay, Vuillemard, Fortin and Chouinard2013; Bovolenta et al., Reference Bovolenta, Romanzin, Corazzin, Spanghero, Aprea, Gasperi and Piasentier2014). This may be why γ-dodecalactone is significantly more abundant in WMP from TMR in this study. The proliferation of both δ-decalactone and δ-dodecalactone in these samples, but especially in WMP derived from CLV and GRS diets, might also relate to changes in the fatty acid profile and hydroxy acid content due to the different diets (Villeneuve et al., Reference Villeneuve, Lebeuf, Gervais, Tremblay, Vuillemard, Fortin and Chouinard2013; Ianni et al., Reference Ianni, Bennato, Martino, Grotta and Martino2020). As thermal treatments increase the levels of free hydroxy acids it may be assumed that dietary differences in lactone contents are exacerbated during the spray drying process for the preparation of the WMP (Calvo and de la Hoz, Reference Calvo and de la Hoz1992).
The remaining VOC that were significantly different based on diet and not derived from lipids were 3-methyl-butanal, dimethyl sulphide, α-pinene, 3-carene, D-limonene, ethylbenzene, o-xylene, acetic acid, and diacetyl. The only Strecker degradation product was 3-methyl-butanal which was significantly more abundant in WMP derived from CLV diets and is a product of leucine metabolism, or also possibly directly transferred from the diet (Faulkner et al., Reference Faulkner, O'Callaghan, McAuliffe, Hennessy, Stanton, O'Sullivan, Kerry and Kilcawley2018). Strecker derived VOCs can also be involved in the Maillard reaction, and some have previously been shown to be indicators of severely heat-treated milk and UHT milk (Calvo and de la Hoz, Reference Calvo and de la Hoz1992; Belitz et al., Reference Belitz, Grosch and Schieberle2004). Previous studies have identified 3-methyl-butanal in milk, but it was not impacted by diet (Bendall, Reference Bendall2001; Faulkner et al., Reference Faulkner, O'Callaghan, McAuliffe, Hennessy, Stanton, O'Sullivan, Kerry and Kilcawley2018). Dimethyl sulphide was significantly higher in WMP derived from TMR than from GRS or CLV diets. Dimethyl sulphide is mainly derived from methionine, but the impact of diet is unclear (Clarke et al., Reference Clarke, Griffin, Rai, O'Callaghan, O'Sullivan, Kerry and Kilcawley2020b), possibly because many sulphur compounds are so reactive and thermally labile (Vazquez-Landaverde et al., Reference Vazquez-Landaverde, Velazquez, Torres and Qian2006; Hougaard et al., Reference Hougaard, Vestergaard, Varming, Bredie and Ipsen2011). Zabbia et al. (Reference Zabbia, Buys and Kock2012) also highlighted that Maillard reactions during heat treatment of milk also generate sulphur- and nitrogen-containing compounds. It is likely that any potential differences due to diet may also be exacerbated by thermal-treatments during the production of the WMP.
Only a minority of terpenes or VOC derived from carotenoids were present in these WMP samples. Terpenes, are directly transferred from feed (Kalač, Reference Kalač2011), but also generated through metabolism of sesquiterpenes in rumen or in milk (Fedele et al., Reference Fedele, Claps, Rubino, Sepe and Cifuni2004). The monoterpenes, α-pinene and 3-carene were significantly more abundant in WMP derived from CLV diets, and D-limonene was significantly more abundant in WMP derived from TMR. Previous studies have highlighted changes in terpene content due to diet and season (Prache et al., Reference Prache, Cornu, Berdague and Priolo2005; Engel et al., Reference Engel, Ferlay, Cornu, Chilliard, Agabriel, Bielicki and Martin2007). ο-Xylene is also potentially derived from β-carotene degradation or possibly from direct transfer from diet (Kilcawley et al., Reference Kilcawley, Faulkner, Clarke, O'Sullivan and Kerry2018). In this study, ο-xylene was significantly higher in WMP derived from TMR diets. Ethylbenzene, another product of carotenoid degradation (Zhang et al., Reference Zhang, Ai, Wang, Tong, Zheng and Sun2016) was significantly higher (P < 0.01) in WMP derived from a CLV diet, but a previous study did not show any impact of diet on ethylbenzene levels in milk (Coppa et al., Reference Coppa, Martin, Pradel, Leotta, Priolo and Vasta2011).
WMP derived from CLV had significantly more acetic acid compared to WMP from either GRS or TMR diets. Acetic acid is primarily a product of carbohydrate metabolism or can be produced from the metabolism of amino acids, but is also known to be directly transferred from feed (Kilcawley, Reference Kilcawley, Fox, Guinee, Cogan and McSweeney2017; Clarke et al., Reference Clarke, Griffin, Rai, O'Callaghan, O'Sullivan, Kerry and Kilcawley2020b). Most studies have found acetic acid in milk, but the dietary impact varies (Croissant et al., Reference Croissant, Washburn, Dean and Drake2007; Villeneuve et al., Reference Villeneuve, Lebeuf, Gervais, Tremblay, Vuillemard, Fortin and Chouinard2013). Diacetyl was significantly more abundant in WMP derived from GRS diets. Diacetyl is widely found in milk and is a result of pyruvate metabolism or direct transfer (Moio et al., Reference Moio, Rillo, Ledda and Addeo1996).
In terms of consumer acceptance testing, creaminess, aftertaste, cooked-flavour, quality and flavour were significantly different in these WMP based on diet. WMP from CLV was significantly higher for quality, which is probably related to the fact that it was also highest for creaminess and flavour, but significantly lowest for cooked flavour, and aftertaste. Diet is known to influence the composition and distribution of fat and protein in milk, which can impact creamy and smooth perception (Frøst and Janhøj, Reference Frøst and Janhøj2007). O'Callaghan et al. (Reference O'Callaghan, Faulkner, McAuliffe, O'Sullivan, Hennessy, Dillon, Kilcawley, Stanton and Ross2016) found that milk derived from a CLV diet had a higher level of fat and protein than milk derived from a TMR diet. In this study, WMP derived from CLV diets scored significantly higher for creaminess, which is probably related to the impact of diet on fat content and distribution. Clarke et al. (Reference Clarke, Griffin, Rai, O'Callaghan, O'Sullivan, Kerry and Kilcawley2020b) also found similar results where a trained descriptive panel scored creaminess significantly higher in pasteurised milk derived from CLV diets in comparison to milk derived from GRS and TMR diets. It is also plausible that the very abundant lactones (δ-decalactone, δ-dodecalactone and γ-dodecalactone) that differed due to diet also impacted creamy perception (Karagül-Yüceer et al., Reference Karagül-Yüceer, Drake and Cadwallader2001; Villeneuve et al., Reference Villeneuve, Lebeuf, Gervais, Tremblay, Vuillemard, Fortin and Chouinard2013). It is difficult to explain a relationship between aftertaste in WMP and the impact of diet, although it may be associated with cooked flavour as both were significantly higher (P < 0.05) in WMP derived from TMR diets. The development of cooked/milky flavour in UHT milk has been suggested to be derived from milk protein denaturation, particularly serum protein, and possibly also linked to volatile sulphur compounds (Deeth, Reference Deeth and Griffiths2010; Zabbia et al., Reference Zabbia, Buys and Kock2012). However, as mentioned, only dimethyl sulphide was found to be significantly different (P < 0.01) in this study based on diet, but at greatest abundance in WMP derived from TMR.
The ODP assessors found a significant impact of cow diet (CLV, GRS and TMR) on WMP for colour, caramelised flavour, sweet aroma, sweet taste, creamy aroma, cooked aroma and cooked flavour. WMP derived from CLV and GRS diets was significantly higher for liking of colour compared to WMP derived from TMR diets (Table 3). This result is similar to that reported by Faulkner et al. (Reference Faulkner, O'Callaghan, McAuliffe, Hennessy, Stanton, O'Sullivan, Kerry and Kilcawley2018) for pasteurised milk, and due to higher β-carotene contents. The correlation of the colour of dairy products and β-carotene content has been extensively highlighted and reviewed by Martin et al. (Reference Martin, Verdier-Metz, Buchin, Hurtaud and Coulon2005). ODP data also clearly identified that both sweet aroma, and sweet taste scored significantly higher for WMP derived from TMR diets. This result is similar to that obtained by Villeneuve et al. (Reference Villeneuve, Lebeuf, Gervais, Tremblay, Vuillemard, Fortin and Chouinard2013), who found sweet flavour higher in milk from cows fed hay as opposed to pasture and silage, as did Croissant et al. (Reference Croissant, Washburn, Dean and Drake2007), when comparing milk from Jersey and Holstein cows fed TMR vs. pasture. Villeneuve et al. (Reference Villeneuve, Lebeuf, Gervais, Tremblay, Vuillemard, Fortin and Chouinard2013) speculated that this may be due to the abundance of γ-lactones, which corresponds well with this study where γ-dodecalactone was present at significantly higher abundances in WMP from TMR and is characterised with a sweet, green odour (Karagül-Yüceer et al., Reference Karagül-Yüceer, Drake and Cadwallader2001). Carmelised flavour was also significantly higher in WMP derived from TMR diets and may also be linked to Maillard reactions (Kilcawley et al., Reference Kilcawley, Faulkner, Clarke, O'Sullivan and Kerry2018), and/ or to the greater abundance of γ-dodecalactone. Both cooked aroma and cooked flavour were significantly higher in WMP derived from a GRS diet compared to WMP from TMR and CLV diets. This is different to that found in the consumer acceptance part of this study, and in previous studies (Clarke et al., Reference Clarke, Griffin, Rai, O'Callaghan, O'Sullivan, Kerry and Kilcawley2020b; Manzocchi et al., Reference Manzocchi, Martin, Bord, Verdier-Metz, Bouchon, Marchi, Constant, Giller, Kreuzer, Berard, Musci and Coppa2021). The only VOC significantly higher in just GRS WMP were butanal and methyl hexanoate neither of which are associated with cooked flavour. The significant difference in creamy aroma which was higher in WMP derived from CLV than from TMR and GRS diets, similar to that found in the consumer part of this study. As mentioned, this may relate to differences in fat particle-size distribution, fat globule flocculation or fat coalescence due to the presence of lower-melting-point fatty acids and lactones due to diet (Richardson et al., Reference Richardson, Booth and Stanley1993; Villeneuve et al., Reference Villeneuve, Lebeuf, Gervais, Tremblay, Vuillemard, Fortin and Chouinard2013; O'Callaghan et al., Reference O'Callaghan, Faulkner, McAuliffe, O'Sullivan, Hennessy, Dillon, Kilcawley, Stanton and Ross2016; Faulkner et al., Reference Faulkner, O'Callaghan, McAuliffe, Hennessy, Stanton, O'Sullivan, Kerry and Kilcawley2018). However, previous studies have not found that diet impacted creamy perception in milk (Manzocchi et al., Reference Manzocchi, Martin, Bord, Verdier-Metz, Bouchon, Marchi, Constant, Giller, Kreuzer, Berard, Musci and Coppa2021).
The WMP derived from GRS diets was positively correlated to cooked aroma and with butanal, 2-pentanone, decanal, undecanal, 2-nonanone, γ-crotonlactone, methyl hexanoate and 2-heptadecanone. It is difficult to associate these individual VOCs with cooked aroma as none have previously been directly associated with this attribute. The WMP derived from CLV diets was positively correlated with creamy aroma, dimethyl disulphide, 2-undecanone, ethyl benzene, 2-ethyl-1-hexanol, 1-pentanol, α-pinene, 3-carene and 3-methyl-butanal. None of these VOCs would typically be associated with cream attributes. However, WMP derived from both GRS and CLV were also associated with many δ lactones that are probably influencing the creamy aroma, especially as some were very abundant. The WMP derived from TMR diets was positively associated with carmelised flavour, sweet aroma and oxidised flavour, but also with dimethyl sulphide, ο-xylene, p-xylene, hexanal, 1-hexanol, γ-dodecalactone, D-limonene, heptanal and dodecanal (obtained from the jack-knife uncertainty test, Fig. 3). Sweet taste has previously been found to be a dominant sensory descriptor for WMP derived from TMR diets (Clarke et al., Reference Clarke, Griffin, Rai, Hennessy, O'Callaghan, O'Sullivan, Kerry and Kilcawley2021) and sweet has also been associated with concentrate diets, like TMR and γ-lactones (Villeneuve et al., Reference Villeneuve, Lebeuf, Gervais, Tremblay, Vuillemard, Fortin and Chouinard2013). Chen et al. (Reference Chen, Zhao, Hao, Yu, Tian and Zhao2017) also found that greater abundances of γ-lactones corresponded with higher scores for the sweet, vanilla and caramel descriptors.
In conclusion, significant differences were evident in the sensory perception and volatile profile of the WMP based on the diet. Consumers rated the overall acceptability, flavour, creaminess and quality of the WMP derived from CLV higher than WMP derived from GRS or TMR diets. Consumers also found that WMP derived from TMR diets scored higher for cooked flavour and aftertaste. The familiarity of the consumers in this study with pasture derived dairy products maybe a factor influencing these results. ODP analysis with trained assessors found that colour was one of the most discriminatory sensory attributes for these WMP based on diet and was significantly positively correlated to WMP derived from CLV diets. Overall ODP analysis found that WMP from all three diets differed, but that WMP from both pasture diets (CLV and GRS) were more closely related, which was confirmed by VOC analysis. The different VOC extraction techniques used provided a more comprehensive volatile profile of these WMP samples, with sixty four individual VOCs identified. Similar trends were evident for those VOCs which were extracted by each technique in relation to the impact of diet. However, DI-HiSorb extracted significantly more VOCs than TD or HS-SPME, but was especially beneficial for extracting lactones. This proved to be very useful as the abundance of some δ-lactones (δ-decalactone and δ-dodecalactone) and γ-dodecalactone were very high. Both δ-decalactone and δ-dodecalactone were significantly more abundant in WMP derived from CLV and GRS diets, with the abundance of γ-dodecalactone significantly higher in WMP from TMR diets. γ-Dodecalactone was probably influencing the sweet aroma and flavour and possibly carmelised flavour associated with WMP from TMR, with δ-decalactone and δ-dodecalactone probably influencing the positive association of creamy aroma with the WMP from the CLV diet. ODP analysis did not find any negative sensory attributes associated with lipid oxidation in these samples, and this is probably due to the fact that they were analysed not long after manufacture, therefore, potential differences did not have sufficient time to develop. It was also apparent that by far most of the significant differences in relation to VOCs in these samples in relation to diet were those primarily derived from fatty acids; lactones, primary and secondary aldehydes, alcohols, ketones and an ester. In hindsight this is not surprising as diet has such a significant impact on the fatty acid profile of milk and is, therefore, likely to impact on subsequent VOCs from this source. This study has also highlighted that differences in VOC due to diet are probably exacerbated by thermal treatment and thus another important contributory factor in WMP manufacture.
Supplementary material
The supplementary material for this article can be found at https://doi.org/10.1017/S0022029922000589.