Until quite recently, radiocarbon dating has been underused to resolve questions about historical or contact-era events in North American archaeology. Because of the perceived security of dates derived from trade good assemblages (e.g., Bradley Reference Bradley2007; Fitzgerald Reference Fitzgerald1990; Kenyon and Kenyon Reference Kenyon and Kenyon1983) and a reliance on canonical telling and retelling of the ethnohistoric record (e.g., Tooker Reference Tooker1964; Trigger Reference Trigger1969, Reference Trigger1976), archaeologists have only recently begun to evaluate the efficacy of absolute dating techniques for linking the archaeological record to historically documented events (e.g., Manning et al. Reference Manning, Birch, Conger, Dee, Griggs, Hadden, Hogg, Ramsey, Sanft, Steier and Wild2018; Thompson et al. Reference Thompson, Jeffries and Moore2019). One such event occurred in the summer of AD 1615 (all calendar dates in this article are AD), when Samuel de Champlain visited a village he called Cahiagué en route to assist an assembled party of Wendat (Huron) warriors in their raid against the Onondaga (Biggar Reference Biggar, Langton and Ganong1922–1936:3:49). Champlain is a figure who looms large in the Canadian historical pantheon, and identifying the sites that he and other notable European explorers and missionaries visited during their voyages occupies a prominent place in the archaeological imagination. There has been a long-standing debate in Iroquoian archaeology about what archaeological site may represent the village of Cahiagué. The primary candidates have been the Ball and Warminster sites (e.g., Fitzgerald Reference Fitzgerald1986; McIlwraith Reference McIlwraith1946, Reference McIlwraith1947; Sykes Reference Sykes1983; Trigger Reference Trigger1976; see Figure 1). Another contact-era site, Hunter's Oro 41, is a third candidate (Fitzgerald Reference Fitzgerald1986); however, it is generally agreed to be too small (0.8 ha) and likely dates to the later Jesuit period, thus being a candidate for a small village that was reported burned down in 1642 (Trigger Reference Trigger1976:660–661; Warrick Reference Warrick2008:219, 237). For these reasons, we do not consider this site here. Until now, much of this debate has rested on historical and linguistic data, as well as relative frequencies and types of trade goods.

Figure 1. Regional map, including sites identified in text. Base map: ESRI.
Although the Warminster-as-Cahiagué debate was believed to have been largely resolved by the 1990s (Fitzgerald et al. Reference Fitzgerald, Knight and Bain1995), recent redating of the Warminster site leads us to suggest that critical, independent assessment of chronological assignments for sites previously dated based on trade good assemblages is warranted. When dated in isolation, and compared with a sequence of village sites located on West Duffins Creek, east of Toronto, Manning and colleagues (Reference Manning, Birch, Conger, Dee, Griggs, Hadden, Hogg, Ramsey, Sanft, Steier and Wild2018) found that Warminster was at least partly contemporary with the Jean-Baptiste Lainé (Mantle) site. This is despite vast disparities in the frequency and types of European goods occurring on each—Warminster containing hundreds of glass beads and other items of European manufacture and the full excavation of Jean-Baptiste Lainé revealing only two European copper beads and an iron fragment. The two sites had previously been placed 75–100 years apart in time on the basis of these trade good assemblages (Birch and Williamson Reference Birch and Williamson2013; Fitzgerald et al. Reference Fitzgerald, Knight and Bain1995). The present analysis is prompted by this demonstrated discrepancy between the relative (trade good-based) and absolute (radiocarbon) temporal records. Our objectives here are twofold. First, to test the assertion that the Warminster site is the most likely candidate for the village of Cahiagué using empirical evidence derived from radiocarbon dating that is independent of trade good chronologies. Second, to evaluate the applicability of employing radiocarbon dating and Bayesian analysis of radiocarbon dates to construct refined timeframes independent of, and as tests of, existing temporal constructs based on trade goods and other archaeological correlates for site sequences from the early contact era. By doing so, we aim to avoid circular reasoning, and to provide new, absolute, temporal datasets that permit the interpretation of contact-era archaeological assemblages as the remains of Indigenous action and agency vis-à-vis the reception, rejection, and negotiation of European influences in the sixteenth- and seventeenth-century Northeast.
In this article, we introduce a suite of recently analyzed AMS 14C dates on short-lived botanicals from the Benson, Sopher, Ball, and Warminster sites and a preserved tamarack (Larix laricina) post associated with House 4 at Warminster (Table 1). The dates derive from four modern radiocarbon laboratories each yielding compatible results. We employ Bayesian chronological modeling (e.g., Bayliss Reference Bayliss2009, Reference Bayliss2015; Bronk Ramsey Reference Bronk Ramsey2009a; Hamilton and Krus Reference Hamilton and Krus2018; Manning et al. Reference Manning, Ramsey, Kutschera, Higham, Kromer, Steier and Wild2006; Whittle et al. Reference Whittle, Healy and Bayliss2011). This technique permits integration of the 14C dates on the short-lived samples, the tree ring 14C wiggle-match dating of the post (e.g., Bronk Ramsey et al. Reference Bronk Ramsey, van der Plicht and Weninger2001; Galimberti et al. Reference Galimberti, Ramsey and Manning2004; Manning et al. Reference Manning, Birch, Conger, Dee, Griggs, Hadden, Hogg, Ramsey, Sanft, Steier and Wild2018), and archaeological and ethnohistoric evidence. We can thus investigate and quantify various possible assumptions concerning the order and duration of site occupations to produce refined age estimates for the sites. Our results indicate that Warminster was occupied during 1615–1616 (see also Manning et al. Reference Manning, Birch, Conger, Dee, Griggs, Hadden, Hogg, Ramsey, Sanft, Steier and Wild2018). In addition, both the archaeological evidence and the radiocarbon evidence (independently) suggest the following set of outcomes:
• Ball, considered the immediate predecessor village of the same community later located at the Warminster site, was earlier than Warminster.
• Sopher was perhaps earlier than the Ball site but may also have overlapped.
• Benson chronologically preceded Warminster, Ball, and Sopher and, although not necessarily in a direct site relocation sequence (but cf. Michelaki et al. Reference Michelaki, Hancock, Warrick and Knight2013), thus sets a likely terminus post quem (TPQ) for these sites. The analysis also places the occupation of Benson somewhat earlier than previous interpretations have suggested (i.e., Ramsden Reference Ramsden2016a, Reference Ramsden, Loewen and Chapdelaine2016b).
This set of findings provides independent evidence for the assertion that Warminster is most likely the village of Cahiagué visited by Champlain. It also confirms that AMS 14C dating of short-lived botanicals and tree rings combined with Bayesian modeling can achieve results that permit the precise pinpointing of historical events in the archaeological record, suggesting that further work of this nature is both achievable and recommended.
Table 1. All Radiocarbon Samples and Conventional Radiocarbon Ages (CRA) Used in This Study.

Notes: Individual 68.2% most likely calibrated ages from OxCal 4.3.2 (Bronk Ramsey Reference Bronk Ramsey2009a) and IntCal13 (Reimer et al. Reference Reimer, Bard, Bayliss, Warren Beck, Blackwell, Ramsey, Buck, Cheng, Lawrence Edwards, Friedrich, Grootes, Guilderson, Haflidason, Hajdas, Hatté, Heaton, Hoffman, Hogg, Hughen, Felix Kaiser, Kromer, Manning, Niu, Reimer, Richards, Marian Scott, Southon, Staff, Turney and van der Plicht2013) with a curve resolution set at five years given as a guide; see especially the wide and ambiguous (100+ years) dating of most of these samples when considered in isolation. For the individual nonmodeled calibrated age ranges at 68.2%, 95.4%, and 99.7% probability, see Supplemental Table 1. Samples with the same Dating Iroquoia Project ID are the same sample, e.g., a single organic specimen that has been divided to obtain multiple measurements.
UGAMS Lab ID designations are as follows: n or no alphabetic label = New pretreatment as per Cherkinsky et al. (Reference Alexander, Culp, Dvoracek and Noakes2010). u = No pretreatment. Sample combusted at 900°C in an evacuated and sealed quartz tube in the presence of CuO to produce CO2, which was then dated by the AMS. r = A remnant of the original pretreated sample was combusted without additional pretreatment at 900°C in an evacuated and sealed quartz tube in the presence of CuO to produce CO2, which was then dated by the AMS (Cherkinsky et al. Reference Alexander, Culp, Dvoracek and Noakes2010). r2 = Complete reanalysis starting with untreated sample following standard UGAMS AAA pretreatment; then sample combusted at 900°C in an evacuated and sealed quartz tube in the presence of CuO to produce CO2, which was then dated by the AMS (Cherkinsky et al. Reference Alexander, Culp, Dvoracek and Noakes2010). Note: this reanalysis date is significantly different (more than 2 SD) from the original date (a chi-squared test shows the two dates are not compatible at the 95% level with being the same radiocarbon age: χ2 df1, T = 6.4 > 3.8 [Ward and Wilson Reference Ward and Wilson1978]). r2r = Remnants of UGAMS-25451-r2 were subjected to a second AAA pretreatment and then usual combustion at 900°C in an evacuated and sealed quartz tube in the presence of CuO to produce CO2, which was then dated by the AMS (Cherkinsky et al. Reference Alexander, Culp, Dvoracek and Noakes2010). rr = A remnant of the original pretreated sample (as used for UGAMS-25451) was subjected to a second AAA pretreatment and then combusted at 900°C in an evacuated and sealed quartz tube in the presence of CuO to produce CO2, which was then dated by the AMS (Cherkinsky et al. Reference Alexander, Culp, Dvoracek and Noakes2010).
The listed UGAMS and GrM δ13C values are from independent IRMS analysis. The VERA values are those from the AMS. The Wk AMS 14C dates, with target preparation at Waikato and the samples then run at the W. M. Keck Carbon Cycle Accelerator Mass Spectrometry Laboratory, are corrected for isotopic fractionation but do not have publicly released δ13C information. This reflects the policy of the Keck Laboratory not to release AMS-produced δ13C values, which necessarily include fractionation and can thus be misleading.
The Isotopes Inc. conventional radiocarbon result from the Sopher site, I-6846, is not corrected for isotopic fractionation. However, the dated material, stated as burned bark, should have a similar value to wood and thus be little affected by the absence of correction for isotopic fractionation (versus the assumed typical wood value used). And so we employ it as published. For I-6846, see Buckley (Reference Buckley1976:184). There is no mention of isotopic correction for the University of Saskatchewan dates from the Benson site, S-1535, S-1539 (Rutherford et al. Reference Rutherford, Wittenberg and Wilmeth1981:126) either in the Rutherford and colleagues (Reference Rutherford, Wittenberg and Wilmeth1981) report or in the earlier laboratory statements cited there. Again, samples of charcoal should not be significantly affected by this absence, and so we use the date as published.
The “n/a” means these samples were collected and dated prior to the Dating Iroquoia project, either as found in previous literature (I-6846, S-1535, S-1539 from, respectively, Buckley Reference Buckley1976; Rutherford et al. Reference Rutherford, Wittenberg and Wilmeth1981) or as published in Manning and colleagues (Reference Manning, Birch, Conger, Dee, Griggs, Hadden, Hogg, Ramsey, Sanft, Steier and Wild2018).
Benson _9b sample: GrM-17562. We note that there was a previous, now failed, date for this sample that was anomalously too old. The original date on this sample, GrM-14545, 443±20 14C years BP, was clearly a too old outlier. The sample was re-pretreated from scratch, remeasured, and a much later date achieved that was compatible with the other Benson data: this is GrM-17562, which we use. In the absence of any other explanation, we have to assume that in the processing of the sample for the original GrM-14545 date, some old carbon was accidentally incorporated into the dated target. Hence GrM-14545 is regarded as “failed” and is not used.
Archaeological and Historical Background
At the time of sustained European contact in the early seventeenth century, Northern Iroquoian peoples comprised confederacies of allied Nations and clusters of associated settlements occupying regions adjacent to the Lower Great Lakes, St. Lawrence Valley, and the Finger Lakes Regions of Ontario, Québec, and New York State. This article focuses on the ancestors of the contemporary Huron-Wendat Nation. Between the thirteenth and seventeenth centuries ancestral Huron-Wendat peoples transitioned from groups occupying small agricultural village communities to a suite of allied Nations sharing a territory located immediately southeast of Georgian Bay, a large bay of Lake Huron (Birch Reference Birch2015; Trigger Reference Trigger1976; Williamson Reference Williamson2014:Figure 1).
The Benson, Sopher, Ball, and Warminster sites were villages of the Wendat Arendarhonon Nation (Trigger Reference Trigger1976:156) or “people at the rock” (Steckley Reference Steckley2007:37; anglicized as the Rock Nation). A well-known sequence of large pre- and protohistoric villages in the Upper Trent Valley has been interpreted as representing that ancestral population (e.g., Ramsden Reference Ramsden1990, Reference Ramsden2016a).
Based on archaeological and historical knowledge, Benson is understood to be one of the last sites in the upper Trent Valley before a population move to the area west of Lake Simcoe, where the Sopher, Ball, and Warminster sites are located (Trigger Reference Trigger1976:156). This 1.5 ha village was excavated in the 1970s (Fogt and Ramsden Reference Fogt, Ramsden, Hanna, Meyer and Dawson1996; Ramsden Reference Ramsden1978, Reference Ramsden1988, Reference Ramsden, Keenleyside and Pilon2009; see Figure 2a). Its occupation has been estimated as starting around 1550 (Ramsden Reference Ramsden2016a) or 1570 (Ramsden Reference Ramsden, Loewen and Chapdelaine2016b) and ending by about 1590 (Ramsden Reference Ramsden2016a; Williamson Reference Williamson2014) or 1600 (Ramsden Reference Ramsden, Loewen and Chapdelaine2016b) based on ceramic seriation and the presence of some European metals. Two primary lines of evidence suggest that the Benson site is earlier than the other Arendarhonon sites. The first is ethnohistoric. Writing about the Arendarhonon in 1636, the Jesuit Lalemant asserts that they came to the country some 50 years earlier (Thwaites Reference Thwaites1896–1901:16:227), interpreted as about 1590 (Williamson Reference Williamson2014:34). Members of the Arendahronon told Champlain that they formerly lived in the Trent valley and had abandoned the area because of fear of their Haudenosaunee enemies (Biggar Reference Biggar, Langton and Ganong1922–1936:3:59). Although the Arendarhonon had a clear interest in the Trent Valley (Trigger Reference Trigger1976:156), it was reportedly abandoned by the time Champlain passed through with the assembled Wendat war party in 1615. The second line of evidence comprises the European trade goods from Benson, which suggest that it dates to the period of very early European contact and so before the last decades of the sixteenth century. Ramsden (Reference Ramsden, Keenleyside and Pilon2009) notes that fragments of European metal—but no glass beads—were found only in House 10 at the site, which he interprets as the leading household in a community faction with strong ties to the St. Lawrence Valley and very early European trade connections. Although we seek to avoid circular reasoning and periodization based on trade good frequencies in this article, the ethnohistoric evidence together with the limited presence of trade goods suggests an occupation prior to Ball that has glass beads of both Glass Bead Periods (GBP) 1 and 2 (Fitzgerald Reference Fitzgerald1986:4).

Figure 2. (a) Benson site plan, modified after Ramsden (Reference Ramsden, Keenleyside and Pilon2009); (b) Ball site plan, including sites identified in text, modified after Michelaki and others (Reference Michelaki, Hancock, Warrick and Knight2013); (c) Overall Warminster site plan, north and south villages indicated; (d) North village plan, including locations of Houses 4, 5, and 9 from which samples were obtained; (e) Plan of House 4 indicating the location of Feature 13. Images c, d, and e are all modified after Sykes (Reference Sykes1983).
The Sopher and Ball sites are thought to have been among the pioneering Arendarhonon settlements in historic Wendake. Sopher is best known for excavations of its ossuary, including the recovery of an iron celt (Noble Reference Noble1971). Limited excavations in the 1.5 ha village revealed two longhouses and a number of middens, although no palisade (Noble Reference Noble1968; Sykes Reference Sykes1980). On the basis of its small trade good assemblage, Sopher is thought to date to the mid to late sixteenth century (Noble Reference Noble1968, Reference Noble1971); thus, it is one of the likely earliest Arendarhonon sites to relocate from the Trent Valley and to have contributed population to the later Arendarhonon sequence.
Ball is a 3.5 ha village that was excavated in its entirety by Dean Knight (Reference Knight1987) in the 1970s and 1980s (see also Martelle Reference Martelle2002; Michelaki et al. Reference Michelaki, Hancock, Warrick and Knight2013; see Figure 2b). Its size suggests that it was the principal village of the Arendarhonon. Based on the similar sizes and proximity of Ball and Warminister, together with interpretations of the ethnohistoric record, it has been assumed that Ball dates to the late sixteenth or early seventeenth centuries and that the Warminster site followed Ball as the primary village in the historic Wendake Arendarhonon sequence (Heidenreich Reference Heidenreich1971, Reference Heidenreich2014; Williamson Reference Williamson2014).
The Warminster site was first identified by Andrew Hunter (Reference Hunter1902) as a large contact-period village with two associated ossuaries, one of which was heavily looted in the late nineteenth century (Heidenreich Reference Heidenreich2014:24; Sykes Reference Sykes1983). The site was subject to intermittent excavations between the 1940s and 1970s (McIlwraith Reference McIlwraith1946; Sykes Reference Sykes1983). Warminster is essentially a double village, consisting of two palisaded sections located approximately 165 m apart (Figure 2c–e). The north village is 3.4 ha in size, and the south village is 2.6 ha. Seven complete longhouses and portions of 13 more were reported from the northern village (Sykes Reference Sykes1983:81, 85). Similarities in the material culture assemblages recovered from each section of the site suggest that they were occupied contemporaneously (Williamson Reference Williamson2014:34). Possible ethnohistoric associations, trade good frequencies, and artifact types have at different times been used to suggest that either Ball preceded Warminster, or vice versa (e.g., Fitzgerald Reference Fitzgerald1986, Reference Fitzgerald1990; Fitzgerald et al. Reference Fitzgerald, Knight and Bain1995; McIlwraith Reference McIlwraith1947). Such debate and ambiguity provide good reason to seek independent radiocarbon confirmation of the dating of these two sites.
Warminster as Cahiagué: History of the Debate
Historical and Linguistic Evidence
The primary ethnohistoric documents cited in the Cahiagué debate were written by Samuel de Champlain (Biggar Reference Biggar, Langton and Ganong1922–1936) and Gabriel Sagard (Wrong Reference Wrong1939). Champlain first visited the Atlantic coast of eastern North America in 1603 before establishing a settlement at what is now Québec City in 1608. In his explorations, he interacted with many Indigenous peoples seeking to develop alliances and trade relations. He was well aware of the enmities that existed between various nations, some of which he perceived as impediments to the diplomatic and economic interests he sought to extend on behalf of France. For these reasons, in 1615, he agreed to join a party of Wendat allies in a raid against the Onondaga, a Haudenosaunee nation in what is now New York State. Accompanied by a contingent of men, he traveled through the country of the Attignawantan (“of bear country” [Steckley Reference Steckley2007:36], the largest and northernmost of the Wendat Nations) to the Arendarhonon, from whose territory the raid would depart. In August 1615, according to his account, he “visited five of the principal villages, enclosed by wooden palisades, as far as Cahiagué, the principal village of the country, which contains two hundred fairly large lodges and where all the warriors were to assemble” (Biggar Reference Biggar, Langton and Ganong1922–1936). It is generally agreed that Champlain's estimate of the number of houses was at best a rough guess (e.g., Heidenreich Reference Heidenreich2014:22). Since Ball and Warminster are believed to be successive iterations of the same community, both may have been the “principal village of the country” (in turn). Along the journey, he notes that they traveled along a “strait” that connected to a larger lake, which is generally interpreted as being Lakes Couchiching and Simcoe (see Figure 1). About September 8, Champlain set off to the eastern Haudenosaunee territory with approximately 500 Wendat and Algonquin warriors. Champlain was wounded during the campaign and returned to Cahiagué on December 23. He remained among the Wendat, both at Cahiagué and traveling within the region, until May 1616.
Sagard was a Recollect lay brother who lived among the Wendat from the summer of 1623 to the autumn of 1624. His writings include detailed descriptions of Wendat life and customs (Wrong Reference Wrong1939), as well as a dictionary of the Wendat language (Steckley Reference Steckley2009). Although Sagard is recognized as the first “ethnographer” of the Wendat, portions of his writings are extensively reproduced from Champlain's own accounts. When Sagard arrived at Cahiagué in 1624, he reported that “the chief town formerly contained two hundred large lodges, each filled with many households; but of late, on account of the lack of wood and because the land began to be exhausted, it has been reduced in size, divided in two, and rebuilt in another more convenient locality” (Wrong Reference Wrong1939:92). It is unclear whether this passage should be interpreted as the village being (1) divided in two, with each part rebuilt adjacent to each other, as is the arrangement at Warminster; (2) divided with its population relocating to two new settlements located at some distance from one another; or (3) divided in two with some portion of the community remaining behind in the preexisting settlement.
Heidenreich (Reference Heidenreich1971, Reference Heidenreich2014) has been one of the staunchest proponents of the identification of Warminster as Cahiagué. He has defended this attribution based on Champlain's accounts of the direction and distance traveled between bodies of water and the villages, the large size of the village, and his analysis of the word Cahiagué as meaning “place divided in two” (Heidenreich Reference Heidenreich1971:303) or “it is cut in two” (Steckley Reference Steckley2007:142). However, as Trigger (Reference Trigger1976:304) has noted, “It is strange that Champlain would not have mentioned such an unusual feature as the village being divided into two separately palisaded units.” This seems especially so given his close eye to military matters in the remainder of his account. As such, interpretive difficulties in both Champlain's and Sagard's accounts call the identification of Warminster as Cahiagué into question.
Trade Goods
Fitzgerald (Reference Fitzgerald1986) notes that in 1615 Champlain was attempting to establish more secure trade connections with the Huron, the Arendarhonon specifically. The Arendarhonon were the first Wendat nation to meet and conclude an alliance with the French (Thwaites Reference Thwaites1896–1901:20:19; Trigger Reference Trigger1976:246). As per Wendat custom, the lineage whose members first discovered a trade route claimed rights to it (Trigger Reference Trigger1976). Assemblages of trade goods, including European metals and glass beads, have been identified at both the Ball and Warminster sites (Fitzgerald Reference Fitzgerald1986:Tables 1–2, Reference Fitzgerald1990:Table 10; Fitzgerald et al. Reference Fitzgerald, Turgeon, Whitehead and Bradley1993; Sykes Reference Sykes1983). The Ball site contains glass beads associated with GBP1 and GBP2, whereas the Warminster site contains beads that date overwhelmingly to GBP2 (Fitzgerald at al. Reference Fitzgerald, Knight and Bain1995:Table 2). In Ontario, there is general agreement that GBP1 dates to 1580–1600 (Garrad Reference Garrad, Pilon and Fox2014; Kenyon and Fitzgerald Reference Kenyon and Fitzgerald1986; Kenyon and Kenyon Reference Kenyon and Kenyon1983); however, the most recent and complete review of trade bead assemblages from the region advises exercising caution about the beginning and ends of such relative, assemblage-based chronologies, drawing attention to distinct regional exchange and supply networks and associated variations in assemblage content between regions (Loewen Reference Loewen, Loewen and Chapdelaine2016).
The assignment of dates for the beginning and end of GBP2 has in fact hinged on the Cahiagué debate. Kenyon and Kenyon (Reference Kenyon and Kenyon1983) dated the Period 2–3 transition to 1616–1624, believing Warminster to be Cahiagué. They based the start of the transition on the date of Champlain's stay and the end on their interpretation of Sagard's claim that the village had been abandoned by 1624 (Wrong Reference Wrong1939:92). Based on his assessment of the trade bead assemblages and the assertion that Ball is most likely the village visited by Champlain in 1615–1616, Fitzgerald dated the GBP2–GBP3 transition to much later: 1628–1632 (Reference Fitzgerald1986:3–7; Kenyon and Fitzgerald Reference Kenyon and Fitzgerald1986:15). A subsequent reassessment of the Ball site trade bead assemblage by Fitzgerald and colleagues (Reference Fitzgerald, Knight and Bain1995) suggested that Ball dates to about 1585–1605 and Warminster to 1605–1623. This reassessment was based on historical and archival evidence of European trade shipments and merchant activity on the east coast and the St. Lawrence corridor.
In both of these assessments, potential problems derive from making absolute date determinations based on the relative presence of trade goods on Indigenous sites, interpretations of Sagard's imperfect descriptions of the nature of the village's relocation and/or division in 1624, and the assumption that both villages are perfectly sequential. For example, Margaret Thompson (in McIlwraith Reference McIlwraith1946:400) notes that European goods were only found in the upper layers of midden deposits at Warminster, suggesting that the settlement was initially occupied prior to the widespread circulation of European material. A similar argument has been used to justify the Sopher village's mid to late sixteenth-century date (Noble Reference Noble1968, Reference Noble1971; Williamson Reference Williamson2014).
Although there have been advances in research on the temporal implications of the geochemical composition of glass beads (e.g., Bonneau et al. Reference Bonneau, Moreau, Hancock and Karklins2013; Hancock Reference Hancock and Janssens2012; Hancock et al. Reference Hancock, McKechnie, Aufreiter, Karklins, Kapches, Sempowski, Moreau and Kenyon2000; Walder Reference Walder2013), these studies often relate to assemblages postdating 1630 (after the period in question here), and they rely on archaeologically based chronologies and broad intervals in which certain bead types were being produced in European manufacturing facilities. Rather than serving to date the time at which these items were used and deposited into the archaeological record, they ultimately date the time at which they were manufactured. This makes these innovative techniques useful complements to existing relative chronologies, but with less precision than the AMS radiocarbon-based methods employed here.
Recent research on the chemical composition and distribution of trade copper has indicated that trade and exchange patterns among the Indigenous peoples in Québec and Ontario were more complex than was previously thought and that the population inhabiting the Ball site may have had preferential access to European material in the early contact era (Pavlish et al. Reference Pavlish, Michelaki, Moreau, Farquhar, Fox, Anselmi, Garrad, Walker, Warrick, Knight and Aufreiter2018). This research also recognizes that different communities had heterogeneous trading connections (see also Jones et al. Reference Jones, Birch, Williamson, Abel, Speakman and Lesage2018), further supporting the need for chronology building that is independent of trade goods and the inherent assumptions involved in the associated scholarly tradition (see also Manning et al. Reference Manning, Birch, Conger, Dee, Griggs, Hadden, Hogg, Ramsey, Sanft, Steier and Wild2018). Trade good chronologies, including the Ontario glass bead chronology, are ultimately relative dating techniques, which are underlain by acculturative assumptions about the way goods of European origin filtered through Indigenous trade networks. For these static chronologies to hold true, European goods would have to have spread through Indigenous networks in a time-and-space transgressive manner, uninhibited by cultural preferences, differing Indigenous attitudes, and differential access to trade routes and trading partners. Clearly, acquiring many more secure and absolute radiocarbon dates from contexts associated with trade beads and other chronologically distinct assemblages will help confirm Ball and Warminster's dates of occupation and refine the larger contact-era chronology for the region, including inferences about spatial and temporal trade good distributions. Such independent, refined chronologies are a necessary precursor to further systematic assessment of absolute dates for historical sites and associated material culture through radiocarbon dating, both in Iroquoia and North America more broadly (see also Thompson et al. Reference Thompson, Jeffries and Moore2019).
Samples and Methods
Samples and Radiocarbon Dates
We recognize that secure stratigraphic provenance for radiocarbon samples from such shallow and usually one-phase Iroquoian sites is sometimes problematic. Our aim was to avoid any material merely from the locus (thus potentially from a contaminated surface find) and to date material stated or reasonably assumed to come from the archaeological excavation of the site (nonsurface finds). Where possible (as in the research reported here), we avoided faunal remains (like deer elements) that could have derived from natural or nonsite occupation hunting episodes. Although it is difficult to assert our findings with total confidence, the fact that we achieve sets of coherent age estimates with only occasional outliers (see the later discussion) suggests we are likely dating the Iroquoian contexts of interest for each site.
The current study (see also Manning et al. Reference Manning, Birch, Conger, Dee, Griggs, Hadden, Hogg, Ramsey, Sanft, Steier and Wild2018) is the first to radiocarbon date materials from Ball or Warminster. Two published dates on charcoal samples exist for Benson (Rutherford et al. Reference Rutherford, Wittenberg and Wilmeth1981:126) and one for Sopher (Buckley Reference Buckley1976:184). Samples of carbonized maize (Zea mays) were acquired from cultural features in three houses (Houses 6, 10, and 14) and a midden (Midden 63) at the Benson site (Figure 2a): these contexts comprise an original or earlier site phase and then a subsequent later site phase including a house extension (House 14) and the stratigraphically overlaying Midden 63 above the House 6 floor. Samples of carbonized maize were acquired from collections associated with excavations at the Sopher site (Noble Reference Noble1968), although they lack specific provenience below the site level. Samples of carbonized maize from the Ball site were acquired from curated feature contexts excavated within Houses 11 and 17 from the older, core site and from Houses 21 and 25 in the later expanded site area (Figure 2b). No associated field notes were available to provide additional contextual data for the samples.
For the Warminster site, both short-lived and dendrochronological samples were acquired, including the remains of a Larix laricina post associated with Feature 13 (Cat #27442) from House 4 at the site (Figure 2d–e). Feature 13 was described in the field notes as a “small, shallow stain” (Kathryn David, personal communication 2017). The location of this feature and its relatively narrow overall diameter suggest that it may have comprised a support for a bench or bunk line. Similar supports are visible in the House 4 plan to the east and south of Feature 13 (Figure 2e). William Fox (personal communication 2015), who participated in excavations at the site in the 1960s, recalled that palisade posts also were observed intact in the field, suggesting that similarly sized structural remains were likely present in the village itself. A dendro-wiggle-match date for the last preserved ring on the post, previously described by Manning and colleagues (Reference Manning, Birch, Conger, Dee, Griggs, Hadden, Hogg, Ramsey, Sanft, Steier and Wild2018), comprised 57 tree rings with inner tree rings and pith absent. There was no bark and the sample was only partially preserved, so the number of tree rings missing from the extant outer ring of the sample to the original bark is not known. Many of the tree rings from the post are narrow, so even a relatively small amount of missing outer wood, and thus rings, could easily represent a few decades of time. The modeled age for the last extant tree ring is thus likely an early TPQ for the occupation period of the site (Supplemental Figure 1). A sample of bean (Phaseolus sp.) from Feature 16B and a sample of wild plum (Prunus americana) from Feature 12 also were obtained, both from House 4. All three features are from the center area inside the longhouse (Sykes Reference Sykes1983:Figure 15). Additional samples of maize from House 9, Feature 30c and House 5, Feature 1 (Figure 2d) were acquired to provide additional context from the surrounding village. All Warminster samples are from the northern village.
We obtained radiocarbon dates on these samples (Table 1; Supplementary Table 1). The calibrated calendar probability distributions from each date are shown against the IntCal13 radiocarbon calibration curve (Reimer et al. Reference Reimer, Bard, Bayliss, Warren Beck, Blackwell, Ramsey, Buck, Cheng, Lawrence Edwards, Friedrich, Grootes, Guilderson, Haflidason, Hajdas, Hatté, Heaton, Hoffman, Hogg, Hughen, Felix Kaiser, Kromer, Manning, Niu, Reimer, Richards, Marian Scott, Southon, Staff, Turney and van der Plicht2013, the current calibration curve at the time of writing) in Figure 3. The nonmodeled calibrated ranges for these dates are shown in Figure 4. We observed, as expected, the ambiguous situation for radiocarbon dates in the period around AD 1500–1600 where, because of the reversal and plateau in the calibration curve, calibrated calendar dating probability can seem to offer multiple date possibilities over a wide (and thus unhelpful) range. For this reason, we applied Bayesian chronological modeling approaches to overcome this inherent ambiguity.

Figure 3. The calibrated calendar age probability distributions for the 45 radiocarbon dates in Table 1 shown against the IntCal13 radiocarbon calibration curve (Reimer et al. Reference Reimer, Bard, Bayliss, Warren Beck, Blackwell, Ramsey, Buck, Cheng, Lawrence Edwards, Friedrich, Grootes, Guilderson, Haflidason, Hajdas, Hatté, Heaton, Hoffman, Hogg, Hughen, Felix Kaiser, Kromer, Manning, Niu, Reimer, Richards, Marian Scott, Southon, Staff, Turney and van der Plicht2013). The ambiguity created by the “wiggle”/plateau between about 1500–1620 is evident. The calibrated probabilities from the three dates run some time before this project on charcoal samples are labeled “C.”
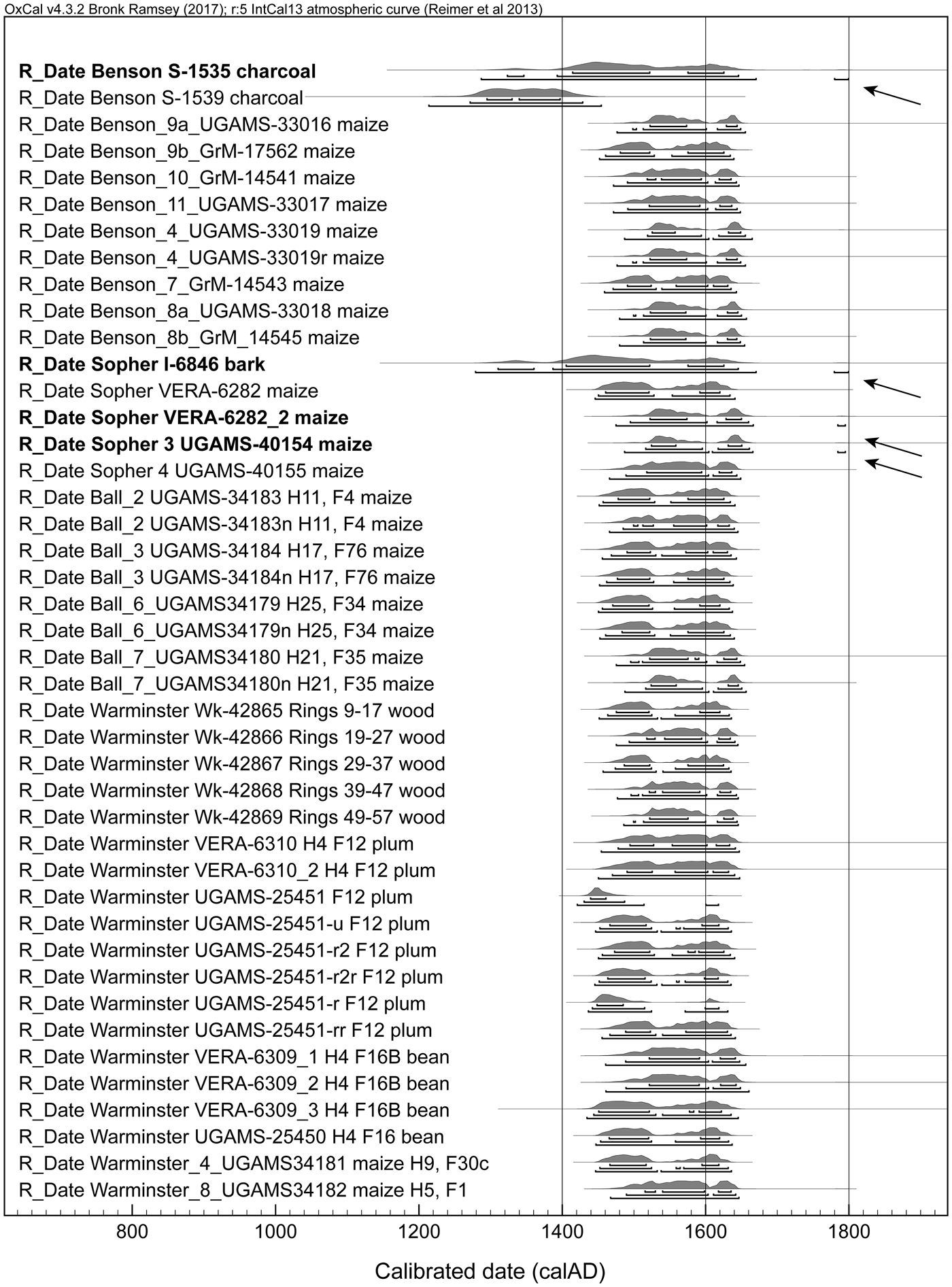
Figure 4. Individual nonmodeled calibrated calendar age ranges for the radiocarbon dates in Table 1 at 68.2%, 95.4%, and 99.7% probability from IntCal13 (Reimer et al. Reference Reimer, Bard, Bayliss, Warren Beck, Blackwell, Ramsey, Buck, Cheng, Lawrence Edwards, Friedrich, Grootes, Guilderson, Haflidason, Hajdas, Hatté, Heaton, Hoffman, Hogg, Hughen, Felix Kaiser, Kromer, Manning, Niu, Reimer, Richards, Marian Scott, Southon, Staff, Turney and van der Plicht2013) employing OxCal (Bronk Ramsey Reference Bronk Ramsey2009a) with curve resolution set at five years. The arrows indicate the four dates (in bold) where at extreme margins they could include post-1661 calibrated calendar date ranges. For ranges, see Supplemental Table 2.
Bayesian Chronological Modeling
We analyze the dataset using the IntCal13 radiocarbon calibration curve with curve resolution set at one year and employing Bayesian chronological modeling via OxCal 4.3 to incorporate prior information (Bronk Ramsey Reference Bronk Ramsey2009a, Reference Bronk Ramsey2009b). Bayesian modeling is a powerful statistical method that permits the incorporation of both prior information (archaeological and historical information) and new data (radiocarbon dates) to constrain possible solutions and so refine understandings of age estimates and associated questions (Bayliss Reference Bayliss2009; Bronk Ramsey Reference Bronk Ramsey2009a). It should be noted that, once calibrated, radiocarbon dates, and subsequent modeling thereof, have probability density functions that are not normally distributed, and therefore, results are presented as highest posterior density (hpd) ranges and not as a value within standard deviations.
Contrary to a situation where there is a known order of strata/phases from stratigraphy (the classic prior knowledge for Bayesian chronological modeling; Buck et al. Reference Buck, Kenworthy, Litton and Smith1991), for these Iroquoian sites, we can be independently sure of either the direct sequence relationships or the order of the sites only infrequently. If we are to avoid circular logic where we merely seek to confirm what is already assumed, we must instead consider the radiocarbon evidence first independently. Thus we begin with the radiocarbon data from our four sites and query whether these data suggest an order among the sites independent of prior assumptions. If we consider the four sites as independent sequences within an OxCal Phase (so the sites may overlap, be separate, or be contiguous in calendar time as the data indicate and not as we assume), we may use the Order function in OxCal to determine the probabilities of the order in calendar time of the dated and modeled elements. The order analysis considers the probability that term 1 (t1) is older than term 2 (t2). For this analysis, we exclude the three 14C dates run on charcoal or bark samples—it is unclear whether I-6846 is just outermost bark, and thus it is best considered as wood-charcoal: its 14C age is substantially older than the dates on short-lived samples from Sopher—both because they only offer clearly old TPQs versus date estimates for the site phase and all have large measurement errors.
There is one additional necessary assumption. As noted earlier, the sixteenth-century reversal/plateau in the 14C calibration curve offers multiple possible ranges for dates in the period we are addressing and spreads possible dating probability out over the calendar time scale (Figures 3 and 4; Supplemental Table 1). However, we have strong indications from ethnohistoric sources and archaeological considerations that typical Iroquoian settlements were not long-lived. Champlain's account of contact period village relocation suggests that “they sometimes change their village site every ten, twenty, or thirty years” (Biggar 1929:3:124). Although the very short earlier seventeenth-century village occupations that were ethnographically reported may not have been typical of earlier periods, no evidence points to occupation periods longer than several decades; for example, around 30 years (Fitzgerald et al. Reference Fitzgerald, Knight and Bain1995:127–131). Warrick's (Reference Warrick1988:47) analysis of wall post density in the longest-lived or “core” houses in a community suggests that the Ball and Warminster sites were occupied for “comparable” periods of time, some 13 and 17 years, respectively. Sykes (Reference Sykes1980) estimated that Warminster would have been abandoned after 12 years because of depleting crop yields, as determined based on soil types in the site's agricultural catchment. Based on syntheses of archaeological and ethnohistoric information, contact period village duration has been estimated at, on average, 15–20 years (Biggar Reference Biggar, Langton and Ganong1922–1936:3:124; Fitzgerald Reference Fitzgerald1986:120; Thwaites Reference Thwaites1896–1901:10:275, 15:153; Wrong Reference Wrong1939:92), and from the discussion of Warrick (Reference Warrick1988) in general, we might infer a maximum span of such villages at about 40 years. Thus, even if we regard this “short” lifetime as itself an ingrained assumption in need of some critical examination, everything indicates relatively short-lived sites. Long site durations appear culturally impossible, despite the 14C calibration curve taphonomy encouraging this possible outcome.
Hence, after an initial model with all data that demonstrates the potential problem of much too overlong phases in the absence of any duration constraints (Model 1, Supplemental Figure 2), with improbable calculated site phase durations at 68.2% and 95.4% probability from an example typical run (with Amodel 96, Aoverall 99) of Benson 0–74 years (68.2%), 0–171 years (95.4%); Sopher 0–143 years (68.2%), 0–514 years (95.4%); Ball 0–184 years (68.2%), 0–521 years (95.4%); and Warminster 0–54 years (68.2%), 0–120 years (95.4%) (Supplemental Figure 3; Supplemental Table 2), we constrain the period of time represented by each site phase (that is between the OxCal Boundaries for the start and end of the relevant OxCal Phase—we are assuming that the dates in each OxCal Phase are a set of estimates randomly sampled from a uniform distribution). On a conservative basis, we consider restricting possible total site phase durations to a uniform probability range of between 0–50 years to 0–120 years—thus, longer than the maximum expected 0–40-year range from ethnohistoric and archaeological assessments to three times this maximum range—by constraining an OxCal Interval query for each site phase (Model 2).
We checked for and downweighted the influence of outliers among the samples in our models using variously two or three of the outlier models in OxCal (Bronk Ramsey Reference Bronk Ramsey2009b). Outlier analysis of the dates in the wiggle-match employed the SSimple Outlier model. For the dates on charcoal and the Sopher “tree bark” date, which we assume may not only include the outermost bark, we applied the Charcoal Outlier model to allow approximately for the in-built age expected in these samples. Outlier analysis for the short-lived (annual) plant matter (individual dates or the R_Combine groupings) employed the General Outlier model. Where radiocarbon dates were run on the very same short-lived sample (and hence the 14C ages measured should each be estimates of the same real 14C age), we employed a weighted average age (Ward and Wilson Reference Ward and Wilson1978) using the R_Combine function, including an additional eight radiocarbon years to allow for annual-scale variation in this period (Stuiver and Braziunas Reference Stuiver and Braziunas1998). We employed the SSimple Outlier model for the individual data within the R_Combine and the General Outlier model for the R_Combine itself (following the coding examples in Higham et al. Reference Higham, Douka, Wood, Ramsey, Brock, Basell, Camps, Arrizabalaga, Baena, Barroso-Ruíz, Bergman, Boitard, Boscato, Caparrós, Conard, Draily, Froment, Galván, Gambassini, Garcia-Moreno, Grimaldi, Haesaerts, Holt, Iriarte-Chiapusso, Jelinek, Pardo, Maíllo-Fernández, Marom, Maroto, Menéndez, Metz, Morin, Moroni, Negrino, Panagopoulou, Peresani, Pirson, de la Rasilla, Riel-Salvatore, Ronchitelli, Santamaria, Semal, Slimak, Soler, Soler, Villaluenga, Pinhasi and Jacobi2014). Outlier analysis is reported with the first number as the posterior outlier probability (in percent) and second number as the prior outlier probability (e.g., O:3/5, 3 = posterior outlier probability, 5 = prior outlier probability). The date is considered a possible outlier when the posterior probability is greater than the prior level of p = 0.05, or 5% (i.e., the posterior probability of being an outlier is above the acceptable level of 5% probability). In the initial Model 1, considering each site independently to investigate likely site order, only one date was found to be a very clear outlier (>50% probability) over multiple runs: UGAMS-25451 (O:92/5 or 91/5), too old. Rerunning Model 1 minus UGAMS-25451, just one date remains as a larger outlier, being greater than 7%: UGAMS-25451-r (O:22/5). This is again too old—although in this case and for UGAMS-25451, this old age may reflect the calibration curve taphonomy and the marked wiggle ~1603–1607 (Manning et al. Reference Manning, Birch, Conger, Dee, Griggs, Hadden, Hogg, Ramsey, Sanft, Steier and Wild2018:Figure S5). We therefore exclude these two dates from the analysis in Model 2, which runs with no outliers above 7% probability and good Amodel and Aoverall OxCal diagnostic values. Model 2 forms the basis of our order analysis. It is important to note that complex OxCal models yield very slightly different results in each run, and sometimes runs do not converge. Here we show typical results from multiple runs and report only runs that completed with acceptable Amodel and Aoverall values (≥60) and with convergence (C) values for each dated element ≥95.
The analysis comprises two stages. In Stage 1 we consider whether a likely relative order could be determined among the four sites (Model 1; see Supplemental Figures 1–3, leading to Model 2). In Stage 2, we then analyze the site phases in terms of the relative order resolved in Stage 1 from Model 2 as a sequence in OxCal (Models 3–6). For Stage 1, the order probabilities determined from Model 2 are detailed for the start and end boundaries for each overall site phase and for a date estimate for each overall site phase across iterations of Model 2 with site phase OxCal Interval queries constrained to 0–50, 0–60, 0–70, 0–80, 0–90, 0–100, and 0–120 years in Supplemental Table 3. The OxCal Date estimate determines a hypothetical event that describes the full temporal extent of the phase. If the start and end boundaries were known exactly, then this date would cover the length of the phase; however, because the start and end boundaries are known only within uncertainties, the date is a combination of the phase period and these start/end uncertainties. Overall, the OxCal Date estimate offers a good summary of the phase. The order results with interval constraints from 0–50 to 0–90 years are very similar; increased ambiguity and then problematic outcomes occur from the 0–100 years to 0–120 years iterations (regarding Sopher versus Ball). However, total site durations longer than 0–50 to 0–80 years seem highly implausible (see the earlier discussion). Thus, the average order results for phase constraints of 0–50 to 0–80 years are shown in Table 2 and form the basis for the Stage 2 sequence. The OxCal runfiles for Model 1 and Model 2 (the version with the OxCal Interval constrained to 0–80 years) are listed in Supplemental Tables 4 and 5.
Table 2. Model 2 Average Order Analysis Probabilities for Site Phase Interval Constraints.

Note: Average of uniform probability OxCal Interval constraint models of 0–50 to 0–80 years, comparing the start and end boundaries for the OxCal Phase for each site and a site OxCal Date estimate; term 1 (t1) is older than term 2 (t2) when the probability is >0.5 (underlined) and vice versa.
In Stage 2, we employ the likely sequence order determined in Stage 1 (comparing order probabilities for start boundaries, date estimates and end boundaries: Table 2, Supplemental Table 3), which turns out to be consistent with the assumed order from the existing ethnohistoric and archaeological assessments (in temporal order from oldest to the most recent):
1. Benson (indicated as older than all the other sites), then, after the move from such late Trent Valley sites to the early Arendarhonon sites around the beginning of the historic Wendake settlement sequence
2. Sopher (indicated as older than Ball)
3. Ball (indicated as more recent than Sopher)
4. The Warminster site (clearly more recent than all the other sites)
Whereas consistent with the ethnohistoric data, we can consider Sopher, Ball, and Warminster as after Benson, and Warminster as after Sopher and Ball, it is less clear whether we should interpret Sopher as entirely earlier than Ball or merely partly earlier and perhaps potentially partly overlapping. The order probabilities (that Sopher is older than Ball) are less than clear-cut (p = 0.53). We thus consider both scenarios (Models 3 and 4 with contiguous phases, and Models 5 and 6 with overlapping Sopher and Ball phases).
We may therefore create refined Bayesian chronological models for the site sequences. In the cases of Benson and Ball we also have an intrasite sequence based on archaeological assessment: some dates relate to an earlier or original core area of the site (Knight Reference Knight1987; Ramsden Reference Ramsden, Keenleyside and Pilon2009), and others relate to a subsequent later stage or expansion. We acknowledge that this is more subjective than a stratigraphic sequence. However, such likely intrasite sequences indicate some measure of additional complexity of history and duration for these settlements that provide valuable information that helps avoid overcompression of time frames. To be conservative, we consider models both with no intrasite sequence information (Models 3 and 5), and with intrasite sequences for Benson and Ball (Models 4 and 6). Once the site phases are no longer considered in isolation, there is no need to constrain site phase duration directly because the ordered sequence requires relatively short site durations: maximum site phase duration found at 95.4% probability = 67 years; average maximum site phase duration = 35 years at 95.4% probability and 15 years at 68.2% probability. Because it is likely that the two sites of Ball and then Warminster are successive iterations of the same community, and so the end of one site will have overlapped with the beginning of its successor, we employ trapezoidal boundaries in this instance (Lee and Bronk Ramsey Reference Lee and Ramsey2012). The two dates identified as the larger outliers in Stage 1 are excluded in the Stage 2 models. For completeness, we include the dates run some time ago on charcoal or tree bark with the Charcoal Outlier model applied. The resulting four models (Models 3–6) covering all variations summarized are shown in full in Supplementary Figures 4–7. The OxCal runfiles for Models 3–6 are listed in Supplemental Tables 6–9.
Results
Results Stage 1: Likely Site Order
The average order probabilities from Model 2 with site phase intervals constrained between 0–50 and 0–80 years are shown in Table 2. These indicate that Benson is likely older than all the other sites and that Warminster is likely more recent than all the other sites. Sopher is indicated as slightly older than Ball, but may well partly overlap.
Results Stage 2: Site Sequence
Models 3–6 incorporate the order identified in Stage 1 and the variations of (1) whether to include the intrasite sequences for Benson and Ball and (2) whether to treat Sopher as earlier than Ball or as potentially overlapping with Ball (see the earlier discussion). Details of the four models are shown in Supplemental Figures 4–7. Selected elements (start and end boundaries and date estimates for each site phase) are listed in Table 3 and shown in Figures 5 and 6 where they are also compared with the 1615–1616 date for Champlain's visit at Cahiagué. It is evident that only the Warminster site is potentially compatible. The likely interval of time between the Benson site and the Sopher or Sopher and Ball sites is relatively short (maximum of 0–15 years at 68.2% hpd, 0–33 years at 95.4% hpd across the four models). The site occupations of Benson, and then one or both of Sopher and Ball, could therefore be approximately contiguous, or there could be an intervening site locus/loci in the Arendarhonon sequence. Only two dates are potentially very minor outliers (at the 6–8% level, varying by model and run): GrM-17562 and VERA-6282_2. Otherwise, the four models have satisfactory Amodel and Aoverall values and offer broadly similar results: Sopher necessarily dates earlier in the contiguous sequence Models 3 and 4.

Figure 5. Details of start and end boundaries and date estimates for the Benson, Sopher, Ball, and Warminster site phases from Models 3 and 4 (contiguous sequences of 1. Benson, then 2. Sopher, then 3. Ball. then 4. Warminster, with Model 4 including the intraphase sequences for Benson and Ball). The 1615–1616 period when Samuel de Champlain was at Cahiagué is indicated in each case. Data from OxCal 4.3.2 (Bronk Ramsey Reference Bronk Ramsey2009a; Reference Bronk Ramsey2009b) and IntCal13 (Reimer et al. Reference Reimer, Bard, Bayliss, Warren Beck, Blackwell, Ramsey, Buck, Cheng, Lawrence Edwards, Friedrich, Grootes, Guilderson, Haflidason, Hajdas, Hatté, Heaton, Hoffman, Hogg, Hughen, Felix Kaiser, Kromer, Manning, Niu, Reimer, Richards, Marian Scott, Southon, Staff, Turney and van der Plicht2013) with curve resolution set at one year. For the full results of Models 3 and 4, see Supplemental Figures 4–5.

Figure 6. Details of start and end boundaries and date estimates for the Benson, Sopher, Ball, and Warminster site phases from Models 5 and 6 (sequences of 1. Benson, then 2. Sopher & Ball in a phase, and then 3. Warminster, with Model 6 including the intraphase sequences for Benson and Ball). The 1615–1616 period when Samuel de Champlain was at Cahiagué is indicated in each case. Data from OxCal 4.3.2 (Bronk Ramsey Reference Bronk Ramsey2009a; Reference Bronk Ramsey2009b) and IntCal13 (Reimer et al. Reference Reimer, Bard, Bayliss, Warren Beck, Blackwell, Ramsey, Buck, Cheng, Lawrence Edwards, Friedrich, Grootes, Guilderson, Haflidason, Hajdas, Hatté, Heaton, Hoffman, Hogg, Hughen, Felix Kaiser, Kromer, Manning, Niu, Reimer, Richards, Marian Scott, Southon, Staff, Turney and van der Plicht2013) with curve resolution set at one year. For the full results of Models 5 and 6, see Supplemental Figures 6–7.
Table 3. Modeled Calendar Age Ranges for Selected Elements from Models 3–6.

The relevance of the intrasite sequences recognized archaeologically at Benson and Ball is evident. Such information helps mitigate against overcompression of the site durations. It is notable that the one model in which the 68.2% hpd date estimate for Warminster does not include Champlain's visit is one of the two models not employing the intrasite sequences (Model 5). We regard the models allowing for the internal sequences at Benson and Ball as most likely yielding our best age estimates (Models 4 and 6). The 68.2% and 95.4% hpd results from Models 4 and 6 are compared to some previous date estimates for the four sites in Figure 7 to illustrate the changes found.

Figure 7. Comparison of some existing date estimates for the Benson, Sopher, Ball, and Warminster sites (Ramsden Reference Ramsden2016a, Reference Ramsden, Loewen and Chapdelaine2016b; Warrick Reference Warrick2008) with the 68.2% hpd ranges from Models 4 and 6 as boxes and the 95.4% ranges indicated by the error bars around these (data from Table 2) and with the 1615–1616 date for Champlain's visit to Cahiagué. Question marks indicate the direction of movement or uncertainty versus some other date estimations in the literature (e.g., Fitzgerald Reference Fitzgerald1986, Reference Fitzgerald1990; Fitzgerald et al. Reference Fitzgerald, Knight and Bain1995; Noble Reference Noble1971; Ramsden Reference Ramsden2016a, Reference Ramsden, Loewen and Chapdelaine2016b).
Discussion
The available independent radiocarbon data indicate that the Warminster site was very likely occupied in the period 1615–1616 when Champlain overwintered in the principal town of the Arendarhonon nation. In contrast, the other sometimes proposed candidate, the Ball site, likely dates earlier, with the end of the Ball site phase boundary no later than cal AD 1598–1607 even at 95.4% probability (Table 2). This conclusion in terms of both the order of the sites and the date ranges is supported both independently from the radiocarbon analysis and from the existing archaeohistoric assessment. Warminster is therefore the current best (indeed only) candidate for Cahiagué of those sites proposed. This largely resolves previous hypotheses and debate. Our modeled age estimates further indicate that Champlain's visit occurred likely toward the later part of the village's overall occupation period and consistent with the stratigraphic associations of trade goods (McIlwraith Reference McIlwraith1947). When these results are considered in light of Sagard's statement—that the village was reduced in size, divided in two, and rebuilt some distance away ca. 1624—we might infer that this process of separation and relocation refers to processes that took place late in the Warminster site's occupation. As such, the statement refers to Cahiagué’s relocation and not its founding.
The Benson–Sopher–Ball–Warminster sequence and dates and other work on the West Duffins sequence comprising the Draper, Spang, and Jean-Baptiste Lainé (Mantle) sites and dates (Manning et al. Reference Manning, Birch, Conger, Dee, Griggs, Hadden, Hogg, Ramsey, Sanft, Steier and Wild2018) begin to open a new window onto a widespread reconsideration of previous archaeological synthesis and the potential resolution of past challenges. One example is the now venerable archaeometric study of Trigger and colleagues (Reference Trigger, Yaffe, Diksic, Galinier, Marshall and Pendergast1980) on a set of Iroquoian ceramics. Trigger and colleagues found what was—given the then-conventional chronology—a problem (see also the summary in Garrad Reference Garrad, Pilon and Fox2014:290). Trace elements in the clays from ceramics found at the Sidey-Mackey site, a large late sixteenth-century village, linked Sidey-Mackey to the Draper site, and Draper to Benson, implying that part of the Draper population resettled at Sidey-Mackey, Benson, or both. But this seemed impossible given the then-conventional chronology, which assumed that Draper dated to as much as a century earlier than both Sidey-Mackey and Benson. However, the new radiocarbon time frame changes this picture, providing similar earlier to mid-sixteenth-century redatings for both Draper (cal AD 1528–1544 68.2% hpd and 1523–1555 95.4% hpd; Manning et al. Reference Manning, Birch, Conger, Dee, Griggs, Hadden, Hogg, Ramsey, Sanft, Steier and Wild2018:Figure 4 model) and, very slightly later, Benson (within cal AD 1519–1572 68.2% hpd and 1507–1585 95.4% hpd: Table 2). Thus, the ceramic-informed scenario found in the analysis of Trigger and colleagues (Reference Trigger, Yaffe, Diksic, Galinier, Marshall and Pendergast1980) now appears quite plausible, given the radiocarbon evidence.
Regarding implications for trade good chronologies, the independent dating of sites in this study and in our previous work (Manning et al. Reference Manning, Birch, Conger, Dee, Griggs, Hadden, Hogg, Ramsey, Sanft, Steier and Wild2018) has shown that we have reason to question foundational assumptions about the timing of site occupations based primarily on trade good data, which assume that similarly dated sites will have similar assemblages of European trade goods. For example, the recognition that the Draper and Benson sites, and the Jean-Baptiste Lainé (Mantle) and Warminster sites were at least partly contemporary with one another is at odds with assumptions derived from the glass bead chronology. No trade goods were identified at Draper, whereas Benson has what would be considered a GBP1 assemblage, Jean-Baptiste Lainé (Mantle) has what would be considered a GBP1 assemblage, and Warminster has what has been considered a GBP2 assemblage. With AMS radiocarbon dates as the primary temporal referent for these sites, we can consider the variation in European trade good assemblages among them as a factor of various social, political, and economic processes. The differential distribution of trade goods on late sixteenth- and early seventeenth-century sites may have been related to a variety of factors including, but not limited to, differential participation in exchange networks, geopolitical maneuvering, differences between mortuary and residential site assemblages, taphonomic processes, or investigation methods. Our results demonstrate that the comparison of chronological assessments derived from independent methods such as radiocarbon analyses and trade good distributions warrants further attention from researchers.
The Arendarhonon are noted in the ethnohistoric literature as being the first Wendat group to encounter the French and, in keeping with Wendat custom, gained the privilege of being the sole traders (Thwaites Reference Thwaites1896–1901:10:225, 20:19). Although they were initially kinship based, early proprietary trading rights gave way to more extensive distribution as the volume of material increased, and the Huron-Wendat entered more fully into the expanding European world-system, as demonstrated by geochemical characterization of copper from early versus later contact period contexts (Pavlish et al. Reference Pavlish, Michelaki, Moreau, Farquhar, Fox, Anselmi, Garrad, Walker, Warrick, Knight and Aufreiter2018). The timing and tempo of this process and its variation between different Wendat communities are just several of the questions for which a refined absolute time frame for contact-era sites, as discussed here, would enable meaningful investigation.
The utility of radiocarbon dates in Iroquoian archaeology has at times been questioned based on the short durations of site occupation and the previously large uncertainties in radiocarbon age determinations. This is especially the case for the later precontact and early contact period (middle to late 1500s through the early 1600s) because of the shape of the radiocarbon calibration curve that spreads out possible date ranges in the absence of constraints (Figure 3). In previous decades, the discrepancies between often imprecise and ambiguous radiocarbon-based dates and those from assessments derived from ethnohistoric and trade goods analysis, and the extrapolation of these via ceramic serialization, created problems and often a wider perception that the radiocarbon analysis was less than helpful. The situation is now fundamentally changed. The combination of high-resolution AMS radiocarbon dating on carefully selected samples directly associated with the archaeological contexts of interest with the application of Bayesian chronological modeling permits a new tight, absolute chronological control and the building of local to regional time frames. Where available, even short dendrochronological sequences for 14C wiggle matching can provide key constraints. The Warminster case investigated in this article demonstrates the ability to date closely a site and to test (and likely confirm) a specific historical association (Champlain in 1615–1616; Figures 5 and 6). The analysis of the Benson–Sopher–Ball–Warminster site sequence demonstrates an ability to resolve and date sites and likely occupation spans at close to an historical-level scale (around decadal-level resolution), as does the West Duffins case (Manning et al. Reference Manning, Birch, Conger, Dee, Griggs, Hadden, Hogg, Ramsey, Sanft, Steier and Wild2018).
Our research agenda should now move forward, given the ability to achieve high-resolution calendar age estimates matching the relatively short life-spans of Iroquoian sites. Targeted radiocarbon dating of suitable samples from secure archaeological contexts should therefore be an objective of modern research agendas in the Northeast. These steps should be undertaken even in cases where trade good chronologies and ethnohistoric accounts point to possible links between the archaeological and historic records. Such independent age verification can help resolve ambiguities in these accounts and usefully test hypotheses based on qualitative data.
Acknowledgments
At the University of Toronto, St. George Campus, we thank Kathryn David for her assistance with access to and information on the post from Feature 13 and the seed samples, and Ted Banning for his permission. We thank William Fox for sharing his recollections of the Warminster excavations. Thanks to Trevor Orchard for facilitating access to the Ball site collections curated at the University of Toronto, Mississauga Campus. We thank Peter Ramsden for providing the Benson samples. We also thank Eva Maria Wild and the VERA Laboratory, University of Vienna; Alan Hogg and the University of Waikato Radiocarbon Laboratory; the Center for Isotope Research, University of Groningen; and the Center for Applied Isotope Studies, University of Georgia. We acknowledge support from the National Science Foundation award BCS 1727802 and thank our Dating Iroquoia collaborator Samantha Sanft. Support was also received from the Department of Classics and College of Arts & Sciences at Cornell University and the Office of the Vice President for Research, the Graduate School, and the Center for Archaeological Sciences at the University of Georgia.
Data Availability Statement
All data used in this paper are available from the text and supplemental materials. The radiocarbon dates used are listed in Table 1. The OxCal runfiles for the models are provided in Supplemental Tables 4–9.
Supplementary Materials
For supplementary material accompanying this article, visit https://doi.org/10.1017/aaq.2019.60
Supplemental Text File. Supplemental Figure Captions.
Supplemental Figure 1. The wiggle-match placement of the Feature 13 wood post, WAR-1, from Warminster.
Supplemental Figure 2. Model 1.
Supplemental Figure 3. The interval query results for the durations of each site phase in Model 1.
Supplemental Figure 4. Example Model 3 results.
Supplemental Figure 5. Example Model 4 results.
Supplemental Figure 6. Example Model 5 results.
Supplemental Figure 7. Example Model 6 results.
Supplemental Table 1. Individual Nonmodeled Calibrated Calendar Age Ranges for the Radiocarbon Dates in Table 1.
Supplemental Table 2. Order Analysis from Model 1.
Supplemental Table 3. Order Analysis from Model 2.
Supplemental Table 4. OxCal Runfile for Model 1.
Supplemental Table 5. OxCal Runfile for Model 2.
Supplemental Table 6. OxCal Runfile for Model 3.
Supplemental Table 7. OxCal Runfile for Model 4.
Supplemental Table 8. OxCal Runfile for Model 5.
Supplemental Table 9. OxCal Runfile for Model 6.