Introduction
Cities are one of the most profoundly altered ecosystems on the planet (Collins et al., Reference Collins, Kinzig, Grimm, Fagan, Hope, Wu and Borer2000), characterised by an intricate mosaic of land uses with different levels of structural complexity and heterogeneous spatial disposition (Andersson, Reference Andersson2006). About 55% of the world's population lives in towns and cities, and the level of urbanisation is projected to be almost 70% by 2050 (UN, 2014). Argentina ranks among the most urbanised countries in the world, with 92% of its population residing in urban settlements, surpassing the regional average of 83% (CEPAL, 2017). These environments are also home to a wide diversity of wild and domestic species. Among them, mosquitoes (Diptera: Culicidae) play a significant role due to their abundance, diversity and sanitary relevance, with certain species specifically adapted to human-altered habitats (Hopken et al., Reference Hopken, Reyes-Torres, Scavo, Piaggio, Abdo, Taylor, Pierce and Yee2021).
Urban land uses are characterised by a unique combination of resources that support mosquito survival and reproduction, such as high density of containers, vegetation cover, different types and quantity of water bodies, shelter, nectar and blood sources, and a particular level of connectivity between optimal habitat patches. The growth of the urban sprawl is happening at an alarming pace, and putting pressure over green spaces, which are becoming increasingly scarce in Latin America (Romanello et al., Reference Romanello, McGushin, Di Napoli, Drummond, Hughes, Jamart, Kennard, Lampard, Solano Rodriguez, Arnell, Ayeb-Karlsson, Belesova, Cai, Campbell-Lendrum, Capstick, Chambers, Chu, Ciampi, Dalin, Dasandi, Dasgupta, Davies, Dominguez-Salas, Dubrow, Ebi, Eckelman, Ekins, Escobar, Georgeson, Grace, Graham, Gunther, Hartinger, He, Heaviside, Hess, Hsu, Jankin, Jimenez, Kelman, Kiesewetter, Kinney, Kjellstrom, Kniveton, Lee, Lemke, Liu, Liu, Lott, Lowe, Martinez-Urtaza, Maslin, McAllister, McMichael, Mi, Milner, Minor, Mohajeri, Moradi-Lakeh, Morrissey, Munzert, Murray, Neville, Nilsson, Obradovich, Odhiambo-Sewe, Oreszczyn, Otto, Owfi, Pearman, Pencheon, Rabbaniha, Robinson, Rocklöv, Salas, Semenza, Sherman, Shi, Springmann, Tabatabaei, Taylor, Trinanes, Shumake-Guillemot, Vu, Wagner, Wilkinson, Winning, Yglesias, Zhang, Gong, Montgomery, Costello and Hamilton2021). While a minimum of 9 m2 of green space per inhabitant is recommended within urban areas (Ramos et al., Reference Ramos, Nunes and dos Santos2020), the average in the Capital City of Argentina is 6.3 m2, with marked differences among neighbourhoods (Marconi et al., Reference Marconi, Perelman and Salgado2022). Urban green spaces play a central role in recreational activities, improving the quality of life of citizens (Groenewegen et al., Reference Groenewegen, Van den Berg, De Vries and Verheij2006; Marconi et al., Reference Marconi, Perelman and Salgado2022). Many studies have reported that the urban mosquito community differs between open green and domestic environments (e.g. de Carvalho et al., Reference de Carvalho, Malafronte Rdos, Miti Izumisawa, Souza Teixeira, Natal and Marrelli2014; Silva et al., Reference Silva Santos, Roberto Pie, Carneiro da Rocha and Navarro-Silva2019; Hopken et al., Reference Hopken, Reyes-Torres, Scavo, Piaggio, Abdo, Taylor, Pierce and Yee2021). In temperate Argentina, the effects of urban habitat features on mosquito distribution and abundance have been previously recognised on vectors of dengue (DENV), St. Louis encephalitis (SLEV) and West Nile virus (WNV) (e.g. Rubio et al., Reference Rubio, Cardo and Vezzani2011; Cardo et al., Reference Cardo, Rubio, Junges, Vezzani and Carbajo2018).
Haematophagy, which is a prerequisite for vitellogenesis in most mosquito species (Clements, Reference Clements1999), is a key component of arboviral transmission dynamics. This is because mosquito blood-feeding patterns represent the connection between vertebrate hosts that can potentially become infected (Lyimo and Ferguson, Reference Lyimo and Ferguson2009). Blood-feeding patterns can be influenced by intrinsic and extrinsic factors. The former includes innate tendencies or genetics, the nutritional state of the mosquito, issues related to flight and behavioural characteristics. Extrinsic factors are those dependent on the hosts which they feed on, habitat characteristics and climatic variables (Takken and Verhulst, Reference Takken and Verhulst2013). It is postulated that innate tendencies are modulated by the spatio-temporal availability of potential sources of blood meals and by different levels of heterogeneity at the local and regional scales (Savage et al., Reference Savage, Aggarwal, Apperson, Katholi, Gordon, Hassan, Anderson, Charnetzky, McMillen, Unnasch and Unnasch2007).
The blood-feeding pattern and the degree of contact between mosquitoes and their vertebrate hosts can be inferred by analysing the blood meal content of field-collected females. Traditionally, this was performed by serological methods but in recent years molecular techniques have been employed (Melgarejo-Colmenares et al., Reference Melgarejo-Colmenares, Cardo and Vezzani2022). These techniques primarily rely on polymerase chain reaction (PCR) and Sanger sequencing of the PCR product. By comparing the obtained DNA sequence against a reference database, it is possible to accurately identify the animal host at a species level (Kent, Reference Kent2009). These methods are relatively simple to perform and have high sensitivity, making them a valuable tool for blood meal analysis.
Although a simple list of hosts for each mosquito species could be valuable, different approaches have been used to reach a deeper comprehension of these interactions. Bipartite networks have recently been applied with an epidemiological approach to the study of ‘host-biting’ communities (Bellekom et al., Reference Bellekom, Hackett and Lewis2021). These networks can be used to describe the central or peripheral role of vertebrates that are part of transmission cycles, predict pathways of disease transmission and identify potential vectors. The present study aims to identify the blood meal source of mosquitoes captured in contrasting settings (i.e. domestic and open green environments) within a megalopolis and an intermediate city, both located in temperate Argentina. Molecular techniques were employed to determine the vertebrate host at the specific level, and bipartite networks were used to describe the interconnections between biting mosquito species and their hosts.
Materials and methods
Study area
The study was carried out in two urbanisations from temperate Argentina, the Área Metropolitana de Buenos Aires (AMBA) and Tandil city, located in the centre of Buenos Aires Province. In AMBA, which is the second megalopolis in South America, sampling was performed in Ciudad Autónoma de Buenos Aires (CABA; 34°36'S, 58°23’W), and in three neighbouring municipalities to the northwest (San Isidro, Vicente López and San Martín). AMBA has an average of 3.2 m2 of green spaces per inhabitant (Observatorio AMBA, Reference Observatorio2023). The region has a temperate humid climate with marked seasonality; the average annual precipitation is 1112 mm and the mean temperature is 17.3 °C (Climate-data.org, 2023). The total area covered by CABA and the three municipalities is approximately 341 km2 and the population is 4,153,234 inhabitants (INDEC, 2022). Tandil (37°04'S, 59°08’W) is an intermediate city that plays a strategic role between rural areas and urban networks (Albaladejo et al., Reference Albaladejo, Barthe, Bustos, Iscaro, Petrantonio and Taulelle2017; Migueltorena, Reference Migueltorena2019). This locality has 24.3 m2 of green spaces per inhabitant (Municipio de Tandil, 2023) with a total population of 150,162 inhabitants (INDEC, 2022). It has an annual cumulative precipitation of 827 mm and a mean annual temperature of 14.2 °C (Climate-data.org, 2023).
Mosquito collection
Engorged mosquitoes were collected in two periods, from November 2019 to March 2020 (spring–summer) and from April to May 2021 (autumn), covering the period favourable for mosquito activity in temperate areas of Argentina. The autumn season could not be carried out during the year 2020, due to the health emergency caused by COVID-19. Collection sites were dwellings, cemeteries, equestrian centres, parks and natural reserves, which were grouped based on the permanent or occasional presence of humans during the day and according to the use given by people, which could modify mosquito and vertebrate composition. Dwellings, cemeteries and equestrian centres were named domestic environments; these sites present artificial containers, impervious surfaces and built structures. Parks and natural reserves were named open green environments, which lack artificial containers and built structures, and present a main herbaceous coverage.
Regarding domestic environments, mosquito collections were performed both indoors and outdoors. Indoor spaces included living rooms, kitchens and bathrooms for dwellings, offices, public bathrooms and galleries for cemeteries, and horse barns, sheds (stocking tools and hay) and public bathrooms for equestrian centres. Domestic outdoor spaces were patios, among vegetation, surroundings of houses, paddocks and among graves. In parks and natural reserves, as there were no indoor environments, sampling was only performed outdoors among mainly herbaceous vegetation.
Sampling sites included a total of 16 domestic (10 dwellings, 3 cemeteries, 3 equestrian centres) and six open green sites (3 parks and 3 natural reserves) in AMBA, and four dwellings and seven parks in Tandil. Samplings were not systematised; however, at least once a month during the two collection periods, all sites of AMBA and Tandil were inspected. At each site, an active search for adult resting mosquitoes was conducted between 7:00 and 13:00 h using nets and manual aspirators (Silver, Reference Silver2008), and/or a battery-powered handheld aspirator adapted from Vazquez-Prokopec et al. (Reference Vazquez-Prokopec, Galvin, Kelly and Kitron2009). Unlike the original Prokopack, a gel battery (12v 4.5AH) and a ventilating motor (model TA-450-DC-L-12v1) were used. Between four and eight collections lasting 5 min each were performed according to the size and structure of each site. Field-collected mosquitoes were transported to the laboratory in a 12 V portable freezer at 10–15 °C.
Mosquito processing
Within a maximum of 24 h following the collection, mosquitoes were killed by freezing for approximately 10 min. Engorged females were morphologically identified to the species level under an 80× stereomicroscope using dichotomous keys by Rossi et al. (Reference Rossi, Mariluis, Schnack and Spinelli2002). Females were individually placed in 1.5 ml Eppendorf tubes containing ethanol 96% and stored at −20 °C until molecular analyses. The abdomen of each specimen was separated from the head and thorax on a glass petri dish sterilised with ethanol 96% using tweezers and flame-sterilised forceps. For the specimens belonging to the Culex pipiens complex, whose blood meal source was successfully identified (as described below), the remaining parts of the specimen (head, thorax and legs) were processed to determine the member of the complex. This was performed as previously described by Cardo et al. (Reference Cardo, Rubio, Junges, Vezzani and Carbajo2020a) using PCR protocols targeting the acetylcholinesterase-2 gene (Smith and Fonseca, Reference Smith and Fonseca2004) and the flanking region of the CQ11 microsatellite (Bahnck and Fonseca, Reference Bahnck and Fonseca2006). Culex pipiens complex members that could not be identified by molecular techniques were designated as Cx. pipiens s.l. For the purpose of the results presented herein, Cx. pipiens s.s. and Cx. quinquefasciatus will be referred to as ‘species’ in a general sense.
Blood meal identification
Host DNA was isolated from each engorged female using a HotShot protocol (Truett et al., Reference Truett, Heeger, Mynatt, Truett, Walker and Warman2000), followed by PCR amplification specific for vertebrate hosts using previously published primer sequences Mod_RepCOI_F (5’-TNTTYTCMACYAACCACAAAGA-3’) and VertCOI_7216_R (5’-CARAAGCTYATGTTRTTYATDCG-3’), premix concentrations and cycling conditions as in Reeves et al. (Reference Reeves, Gillett-Kaufman, Kawahara and Kaufman2018). Each reaction included a negative control in which sterile distilled water replaced extracted DNA to monitor for potential contamination, as well as a positive control established in preliminary tests. For each PCR product, 5 μl plus 1 μl of loading buffer 6× were loaded into a well in an ethidium bromide-stained 1.5% agarose gel, and electrophoresed for approximately 30 min at 90 V. Reaction products were subsequently visualised under ultra violet light to determine amplification success. A 50-base pair (bp) DNA ladder (PB-L®) loaded alongside PCR products was used to determine if amplification was successful based on the presence of a band at the expected fragment length (around 244 bp). If the obtained concentration was below 20 ng/μl, as determined by visual comparison with the ladder, PCR products were subjected to an additional cycle under the same conditions to increase the DNA concentration. PCR products were shipped to Macrogen Korea for purification and sequencing. The sequences were analysed using the ApE software version 0.55 (Davis, Reference Davis2021) and identified to species by comparison with the GenBank DNA sequence database (NCBI, 2022). Sample sequences with >95% identity to published sequences were considered a match for the corresponding species.
Network analysis
A bipartite network was constructed for each setting (domestic and open green environments) of AMBA and Tandil. Four interaction-weighted matrices were built, with mosquito and vertebrate species in rows and columns, respectively. The value in each cell of the matrix was the total number of identified blood meals originated from each vertebrate species for each mosquito species during the entire collection period.
To characterise mosquito–blood source networks structure, the following indices were used: (1) connectance, the realised proportion of possible links, calculated as the ratio between the actual and the maximal number of links (Dunne et al., Reference Dunne, Williams and Martinez2002); (2) network-level specialisation (H2), ranging between 0 (no specialisation) and 1 (complete specialisation) (Blüthgen et al., Reference Blüthgen, Menzel and Blüthgen2006); and (3) Nestedness measure based on Overlap and Decreasing Fill (NODF), a measure of nestedness that considers the overlap between pairs of nodes, ranging between 0 (non-nestedness) and 100 (complete nestedness) (Almeida-Neto et al., Reference Almeida-Neto, Guimarães, Guimarães, Loyola and Ulrich2008).
The significance of observed network metrics was determined by comparison against expected estimates obtained by 1000 randomly generated network matrices (null models) using the algorithm proposed by Patefield (Reference Patefield1981). This algorithm generates network matrices with a randomised distribution of interactions while maintaining fixed marginal values. Z-scores were calculated to quantify the statistical significance of each observed metric in comparison with the distribution of null model values, calculated for each index as:

with i being each index (connectance, H2, NODF) and j either AMBA-domestic, AMBA-open green, Tandil-domestic or Tandil-open green. Each observed value was plotted against the distribution of random values and then tested to quantify how much standard deviation each observed metric was from the mean of the null models values, assuming a Gaussian distribution.
Also, several indices were calculated at the species level. Species strength was obtained for vertebrate hosts to quantify a species’ relevance across all its partners, ranging between 0 (minimum relevance) and the number of species in the other group (maximum relevance) (Bascompte et al., Reference Bascompte, Jordano and Olesen2006). For mosquito species, the Paired Difference Index (PDI) was calculated, this is a weighted estimator of the number of interactions of each mosquito species with the vertebrate level and takes values between 0 (perfect generalist) and 1 (perfect specialist) (Poisot et al., Reference Poisot, Lepennetier, Martinez, Ramsayer and Hochberg2011). Also, the effective number of vertebrates bitten by each mosquito species was calculated using the effective partners index.
Results
Mosquito composition and abundance
A total of 329 engorged mosquitoes were collected (AMBA: 264, Tandil: 65). In AMBA, specimens belonged to 10 mosquito species; almost 73% (193) were members of the Cx. pipiens complex, followed by Ae. albifasciatus (11%), Ae. aegypti (8%), Cx. apicinus (3%), Cx. chidesteri (2%), Ae. crinifer (1%) and Cx. maxi (1%), all of which were present at both domestic and open green environments. Blood fed Cx. bidens, Cx. dolosus and Cx. lahillei were collected only in open green environments, comprising <1% of all captures for each one (table 1). In Tandil, five mosquito species were collected; Ae. albifasciatus accounted for 34% of total captures and was the only species collected in open green environments, whereas in domestic ones the members of the Cx. pipiens complex (31%), Ae. aegypti (19%), Cx. apicinus (13%) and Cx. dolosus (3%) were also captured (table 1). Successful identification of the members within the Cx. pipiens complex was achieved for 57 out of the 66 engorged females (AMBA: 44/52, Tandil: 13/14). In both urbanisations, Cx. quinquefasciatus, Cx. pipiens molestus and the hybrid between the two were identified, albeit in different proportions (table 2).
Table 1. Number of engorged females per mosquito species collected in domestic and open green environments from Área Metropolitana de Buenos Aires (AMBA) and Tandil, Argentina
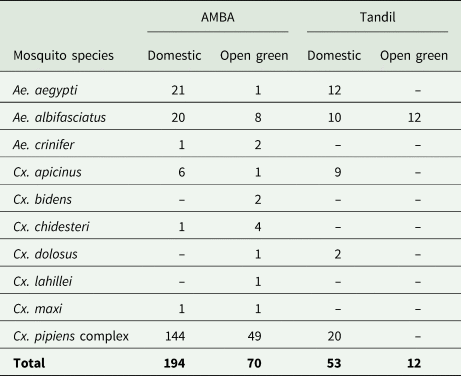
Table 2. Number of blood meals identified per mosquito species collected in domestic and open green environments from Área Metropolitana de Buenos Aires (AMBA) and Tandil, Argentina
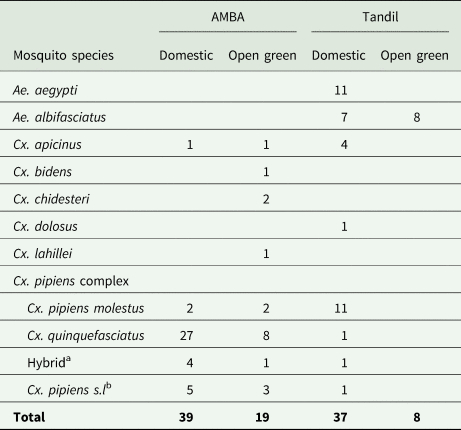
a Hybrid form between Cx. pipiens molestus and Cx. quinquefasciatus.
b Indicates members of the Cx. pipiens complex could not be identified by molecular techniques.
Blood meal identification
A total of 103 blood meals were identified, 58 from AMBA and 45 from Tandil (table 2), as the remaining specimens did not return a visible PCR band. Overall, blood from 23 vertebrate species (18 birds, 5 mammals) was isolated from Ae. aegypti, Ae. albifasciatus, Cx. apicinus, Cx. bidens, Cx. chidesteri, Cx. dolosus, Cx. lahillei and members of the Cx. pipiens complex (fig. 1). None of the samples from Ae. crinifer (n = 3) and Cx. maxi (n = 2) could be identified.
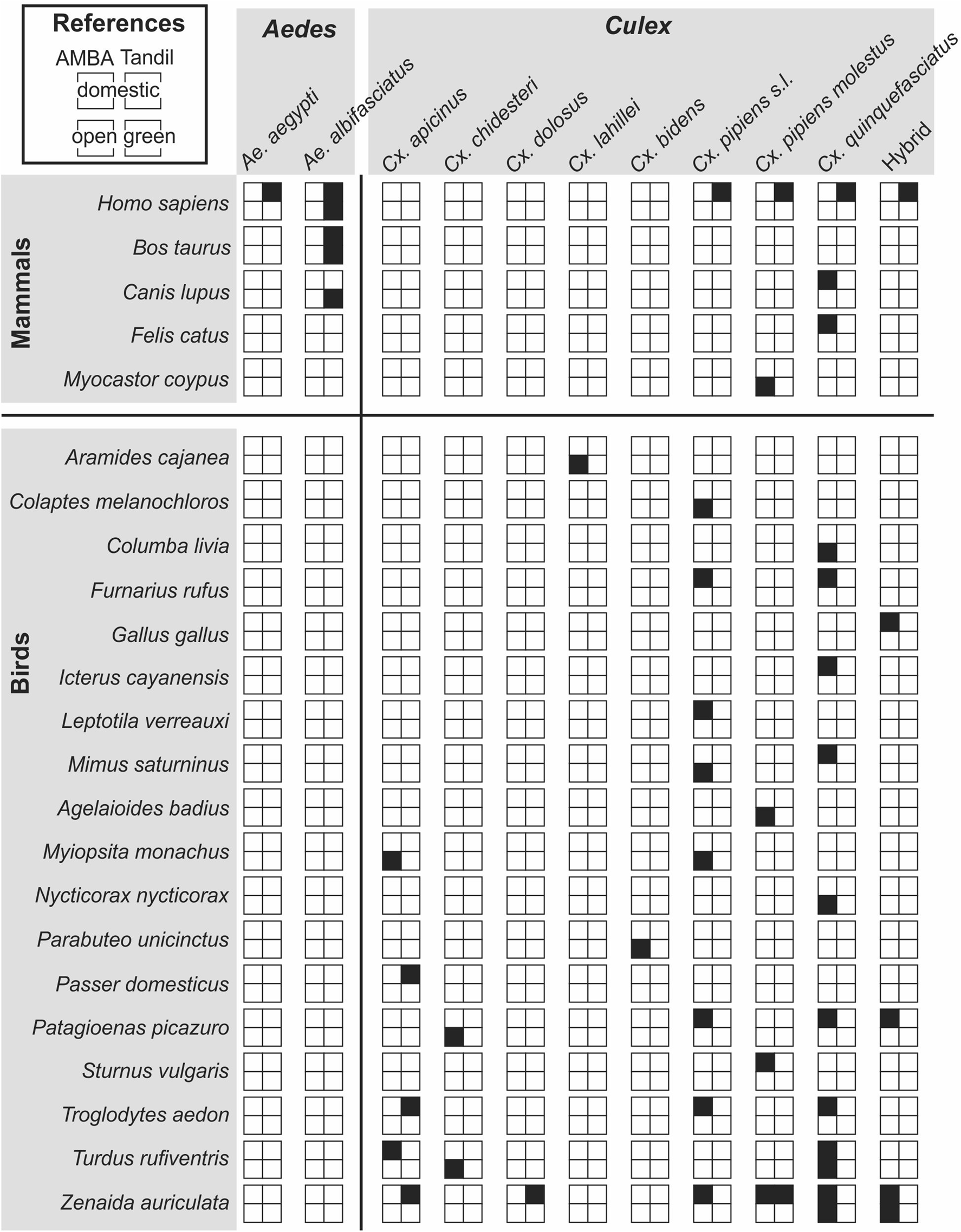
Figure 1. Blood sources of mosquitoes collected in domestic and open green environments from Área Metropolitana de Buenos Aires and Tandil, Argentina.
In AMBA, blood from 20 vertebrate species (17 birds, 3 mammals) was identified from six mosquito species plus the hybrid between Cx. quinquefasciatus and Cx. pipiens molestus (fig. 1). Birds were represented by 13 families (Ardeidae, Accipitridae, Columbidae, Furnariidae, Icteridae, Mimidae, Picidae, Phasianidae, Psittacidae, Rallidae, Sturnidae, Troglodytidae, Turdidae). In domestic environments (n = 39), 36 blood meals from 10 avian species were identified; Zenaida auriculata (Columbiformes: Columbidae) (14), Turdus rufiventris (Passeriformes: Turdidae) (6), Mimus saturninus (Passeriformes: Mimidae) (5), Patagioenas picazuro (Columbiformes: Columbidae) (3), Furnarius rufus (Passeriformes: Furnariidae) (2), Troglodytes aedon (Passeriformes: Troglodytidae) (2), Icterus cayanensis (Passeriformes: Icteridae) (1), Sturnus vulgaris (Passeriformes: Sturnidae) (1), Gallus gallus (Galliformes: Phasianidae) (1) and Leptotila verreauxi (Columbiformes: Columbidae) (1). In open green environments (n = 19), 18 blood meals from 11 avian species were identified; Z. auriculata (5), Columba livia (Columbiformes: Columbidae) (2), Myiopsitta monachus (Psittaciformes: Psittacidae) (2), T. rufiventris (2), Ageloides badius (Passeriformes: Icteridae) (1), Aramides cajanea (Gruiformes: Rallidae) (1), Colaptes melanochloros (Piciformes: Picidae) (1), M. saturninus (1), Nycticorax nycticorax (Ciconiiformes: Ardeidae) (1), P. picazuro (1) and Parabuteo unicinctus (Accipitriformes: Accipitridae) (1). Regarding mammalian hosts, Canis lupus (Carnivora: Canidae) (2) and Felis catus (Carnivora: Felidae) (1) were identified from Cx. quinquefasciatus specimens collected in domestic environments, while Myocastor coypus (Rodentia: Echimyidae) (1) was found in a Cx. pipiens molestus specimen from open green environments (figs 1 and 2).
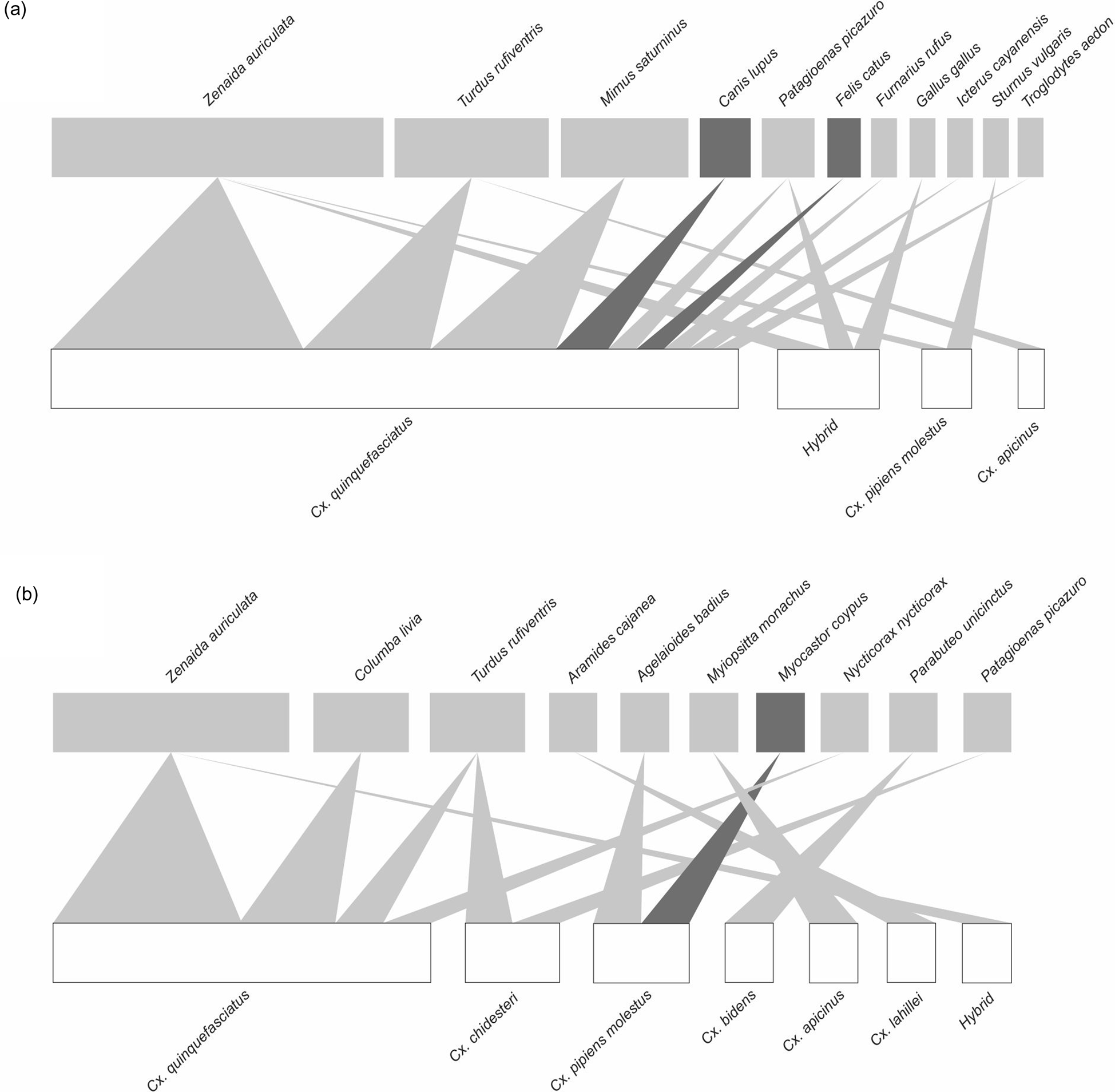
Figure 2. Bipartite networks of mosquito populations (low level) and their vertebrate hosts (high level) in (A) domestic and (B) open green environments of AMBA (Argentina) from November to May 2019–2020 and April to May 2021–2021. In the low level, Culex species are represented in white. High-level colours are dark grey for mammalian hosts and light grey for avian hosts. The width of the connection lines indicate the strength of the interactions (in relative numbers of feeds).
In Tandil, blood from six vertebrate species (3 birds, 3 mammals) was identified from six mosquito species plus the hybrid between Cx. quinquefasciatus and Cx. pipiens molestus (figs 1 and 3). Birds were represented by three families (Columbidae, Passeridae and Troglodytidae). In domestic environments (n = 37), seven avian blood meals were recorded, most of them corresponding to Z. auriculata (5) and a unique blood meal from Passer domesticus (Passeriformes: Passeridae) and T. aedon (figs 1 and 3). Among the mammals, 29 samples corresponded to Homo sapiens (Primates: Hominidae) from Ae. aegypti (11), Ae. albifasciatus (6) and the Cx. pipiens complex (12), and Bos taurus (Artiodactyla: Bovidae) with one blood meal from Ae. albifasciatus. In open green environments (n = 8), blood from three mammalian species was identified; these were H. sapiens (4), B. taurus (3) and C. lupus (1) (figs 1 and 3).
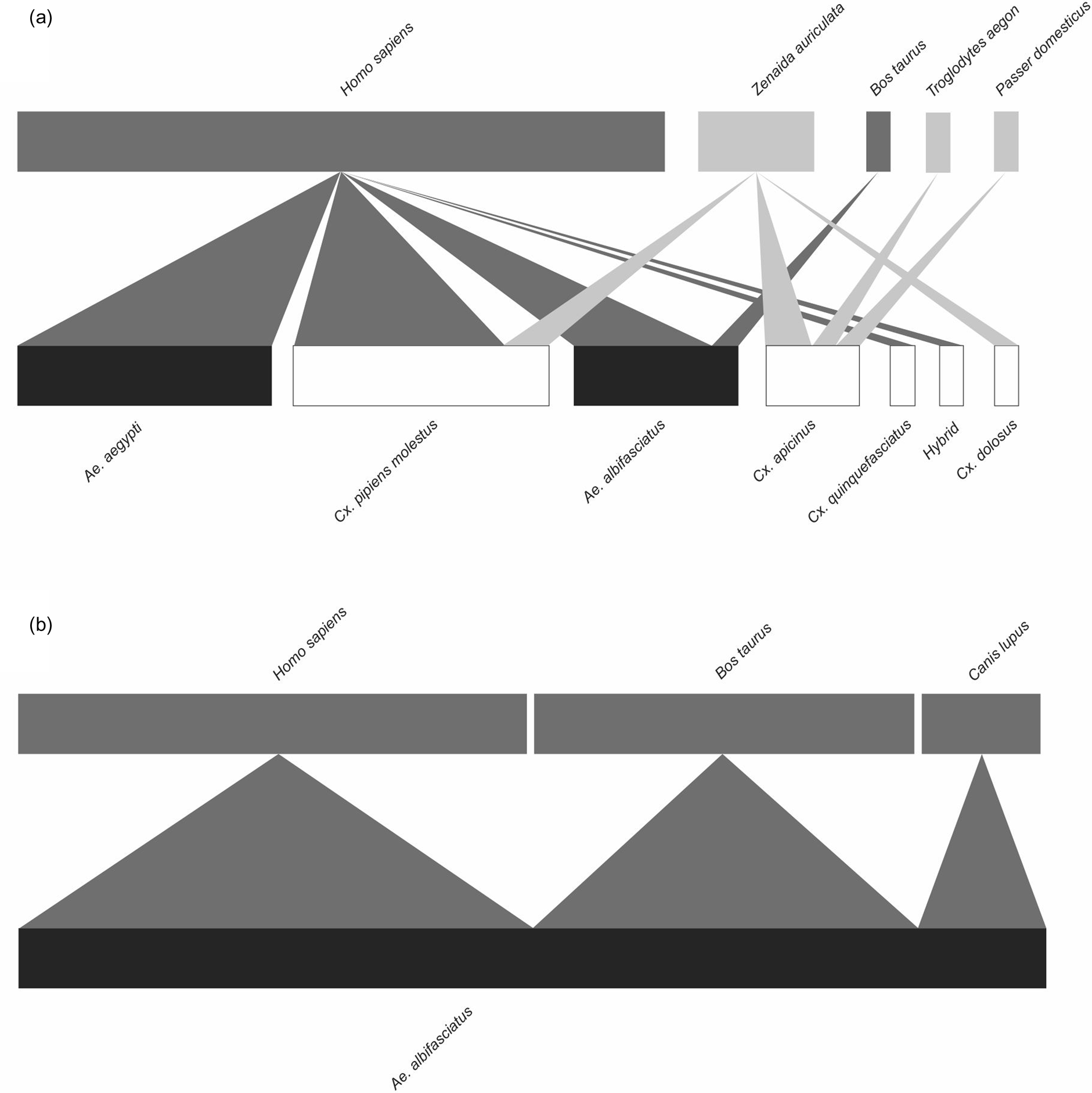
Figure 3. Bipartite networks of mosquito populations (low level) and their vertebrate hosts (high level) in (A) domestic and (B) open green environments of Tandil (Argentina) from November to May 2019–2020 and April to May 2021–2021. Low-level colours are black for Aedes species and white for Culex species. High-level colours are dark grey for mammalian hosts and light grey for avian hosts. The width of the connections line indicates the strength of the interactions (in relative numbers of feeds).
Mosquito–animal blood source network
In AMBA, the mosquito–blood source network in open green environments was composed by more mosquito species than in domestic ones, whereas both presented similar number of vertebrate hosts (fig. 2). In contrast, in Tandil the mosquito–blood source network in open green environments was extremely small and composed by a single blood fed mosquito species (fig. 3), which precluded the calculation of network indices.
The proportion of realised links was lower in open green than in domestic environments from AMBA as reflected by connectance values (table 3). For the AMBA-open green and Tandil-domestic environments, the connectance index obtained was lower than the distribution of values simulated by null models, suggesting some degree of host selection. In accordance, the observed network-level specialisation metric H2 was higher than that expected by chance, while the observed NODF index estimate did not differ significantly than that expected by chance (table 3).
Table 3. Characterisation of the host–mosquito biting interactions in domestic and open green environments from Área Metropolitana de Buenos Aires (AMBA) and Tandil, Argentina

Network level indices are connectance, network level specialisation (H2) and nestedness measure based on overlap and decreasing fill (NODF); mosquito species level indices are Paired Differences Index (PDI) and effective partners
a Lower than expected compared to null models.
b Higher than expected compared to null models.
c Not possible to calculate index due to small web size.
d Hybrid form between Cx. pipiens molestus and Cx. quinquefasciatus.
At the species level, at both settings from AMBA Cx. quinquefasciatus exhibited the lowest value of PDI and highest value of effective partners, suggesting a generalist biting behaviour (table 3, fig. 2). In Tandil, a similar pattern was observed for Cx. apicinus which was more connected with (avian) hosts and presented lowest PDI value and highest value of effective partners (table 3, fig. 3). Aedes species showed a high number of identified feeds exclusively from mammals, in particular Ae. aegypti exhibited a perfect specialisation for humans (table 3). It is noteworthy that within the Cx. pipiens complex, in Tandil the most connected species was Cx. pipiens molestus.
Regarding vertebrate hosts, in both settings from AMBA two avian species, Z. auriculata and T. rufiventris, presented highest strength values. In contrast, in domestic environments from Tandil, H. sapiens appears to be the keystone blood source for mosquito among other animals, with highest strength value, followed by Z. auriculata (table 4).
Table 4. Vertebrate species strength of host–mosquito biting interactions in domestic and open green environments from Área Metropolitana de Buenos Aires (AMBA) and Tandil, Argentina

Discussion
Information regarding the origin of mosquito blood meals collected in urban environments is fundamental for linking mosquito–host contacts and understanding the transmission networks of infectious diseases that may affect humans, domestic animals and wildlife living in urban areas. In this study, we reported the collection and analysed the meal origin of engorged mosquito specimens in two urbanisations from temperate Argentina. To the best of our knowledge, this is the first published study that examined mosquito feeding patterns in different urban environments from Argentina using molecular techniques. More importantly, we provide the first regional data on blood meal sources for Ae. aegypti and Cx. bidens, and the first human blood meal records for Ae. aegypti, Ae. albifasciatus and Cx. pipiens molestus in South America (see Melgarejo-Colmenares et al., Reference Melgarejo-Colmenares, Cardo and Vezzani2022). Mammals comprised 41% of total feeds, with blood meals derived from human, cow, dog, cat and coypu. Among avian hosts, the best represented species were eared dove and rufous-bellied thrush. Several of the mosquito species collected have been previously incriminated in the transmission of DENV, SLEV, WNV, western equine encephalitis and the dog heartworm Dirofilaria immitis (Contigiani et al., Reference Contigiani, Díaz, Spinsanti, Berón, Campos, Gleiser, Díaz Nieto, Salomón and Schweigmann2016; Vezzani and Eiras, Reference Vezzani, Eiras, Berón, Campos, Gleiser, Díaz Nieto, Salomón and Schweigmann2016).
Despite the fact that many studies have mentioned the preference of Ae. aegypti for humans and its prominent role as vector of Flaviviruses in South America, no previous studies have been conducted on field-collected specimens in the continent (Melgarejo-Colmenares et al., Reference Melgarejo-Colmenares, Cardo and Vezzani2022). Herein, humans were the only blood meal source identified. Previous researches have shown that Ae. aegypti rarely feeds on other animals such as birds (chicken) and other mammals (cat, dog and rabbit) in low abundance compared with humans (e.g. Jansen et al., Reference Jansen, Webb, Graham, Craig, Zborowski, Ritchie, Russel and van den Hurk2009; Sivan et al., Reference Sivan, Shriram, Sunish and Vidhya2015). The fact that Ae. aegypti shows a preference for and frequently feeds on humans increases the potential risk for pathogen transmission by infected mosquitoes in urban areas (Harrington et al., Reference Harrington, Edman and Scott2001). Our results confirm that Ae. aegypti has a high level of contact with humans, which promotes virus transmission in temperate Argentina. This is in line with recent DENV and CHIKV epidemics in the country, which have reported over 121,000 and 1600 cases, respectively, notably about half of them in the temperate region (BEN, 2023).
Ae. albifasciatus was the sole engorged species collected in both settings of both urbanisations. All identified blood meals were from mammals (humans, cows and dogs), in agreement with previous results in the country (Hack et al., Reference Hack, Torales, Bar and Oscherov1978; Cardo and Vezzani, Reference Cardo and Vezzani2023). Other studies carried out in South America showed a great capacity for feeding on different hosts (reviewed by Melgarejo-Colmenares et al., Reference Melgarejo-Colmenares, Cardo and Vezzani2022). The fact that Ae. albifasciatus feeds on domestic animals is of veterinary interest, as it can contribute to the transmission of pathogens resulting in severe animal diseases, such as dirofilariasis and SLEV.
The members of the Cx. pipiens complex are widely recognised vectors of arboviral encephalitides. In particular, Cx. quinquefasciatus is the most connected vector of mosquito-borne pathogens (Yee et al., Reference Yee, Bermond, Reyes-Torres, Fijman, Scavo, Nelsen and Yee2022), and its synanthropic nature of this species further emphasises its significant role as a vector in close association with human habitation (Taipe-Lagos and Natal, Reference Taipe-Lagos and Natal2003). The present study provides evidence that both members present in the region feed on various vertebrates including mammals, wild and domestic birds. For Cx. pipiens molestus, several hosts are reported for first time; i.e. H. sapiens, M. coypus, Z. auriculata, S. vulgaris and A. badius. In turn, for Cx. quinquefasciatus new host records correspond exclusively to avian species, namely C. livia, F. rufus, I. cayanensis, N. nycticorax and T. aedon. Although Cx. pipiens molestus and Cx. quinquefasciatus have been traditionally described in the literature as mammophilic (Vinogradova, Reference Vinogradova2000), more recent studies have reported that both also feed on birds (e.g. Gomes et al., Reference Gomes, Sousa, Vicente, Pinho, Calderón, Arez, Almeida, Donnelly and Pinto2013; Cardo et al., Reference Cardo, Carbajo, Mozzoni, Kliger and Vezzani2023), highlighting its potential role in the dispersal of avian malaria (Whiteman et al., Reference Whiteman, Goodman, Sinclair, Walsh, Cunningham, Kramer and Parker2005). Given the occurrence of human and horse blood meals, they could also act as bridge vectors of arboviral encephalitides to mammals. Therefore, feeding patterns and the role of the Cx. pipiens complex in disease transmission cycles should be constantly monitored. The bioform Cx. pipiens pipiens could complete the complex network of encephalitides transmission cycles, but until the present it has not been documented in South America (Cardo et al., Reference Cardo, Rubio, Vezzani and Carbajo2020b).
Of the 18 avian species identified as hosts in the present study, eight have been found naturally infected with SLEV and/or WNV and four only for SLEV (Díaz et al., Reference Díaz, Quaglia, Flores and Contigiani2011, Reference Díaz, Flores, Quaglia and Contigiani2018). In AMBA, eared dove and rufous-bellied thrush showed the highest strength values, while in Tandil, eared dove was the second key host, after humans. Eared dove is one of the most relevant species for virus spread and amplification and an effective target for its surveillance (Díaz et al., Reference Díaz, Flores, Quaglia and Contigiani2018). It is also the most widespread and abundant dove in South America, found in open and disturbed habitats including towns and cities (Leveau and Zuria, Reference Leveau and Zuria2017). Our current findings indicate a potential risk of SLEV transmission and support the hypothesis that the requirements and conditions for amplification and enzootic maintenance of SLEV may exist in urban areas of temperate Argentina. Further studies are needed to investigate the relationship between locally abundant bird species and mosquitoes to fully understand their role in the enzootic cycle of SLEV.
The low number of identified blood meals from Cx. apicinus, Cx. bidens, Cx. chidesteri, Cx. dolosus and Cx. lahillei precludes the possibility of establishing feeding patterns for these species. Previous studies conducted in South America have shown that some of them feed on birds (as was observed here), mammals (human, horse, rabbit), tortoise and toad (Almirón and Brewer, Reference Almirón and Brewer1995; Melgarejo-Colmenares et al., Reference Melgarejo-Colmenares, Cardo and Vezzani2022; Cardo and Vezzani, Reference Cardo and Vezzani2023). Even though in the present study, no human feeds were identified for these species, their potential role as regional vectors of zoonotic relevance should not be dismissed, as for instance Cx. apicinus and Cx. dolosus have been involved in the transmission of SLEV (Díaz et al., Reference Díaz, Albrieu Llinás, Vázquez, Tenorio and Contigiani2012) and D. immitis (Yee et al., Reference Yee, Bermond, Reyes-Torres, Fijman, Scavo, Nelsen and Yee2022) respectively. Regarding Cx. bidens, Cx. chidesteri and Cx. lahillei, whose potential role in diseases transmission is unknown, it is still important to obtain information on their diet at the local scale. New vector diseases are constantly emerging in different regions of the globe and for many of them transmission cycles, including their vectors, are still unknown.
In line with Pickett et al. (Reference Pickett, Cadenasso, Grove, Boone, Groffman, Irwin, Kaushal, Marshall, Mcgrath, Nilon, Pouyat, Szlavecz and Warren2011), who stated that urbanisation has a profound effect on many aspects of the environment (e.g. energy flux, impermeable surfaces, altered flora) and these features negatively impact biodiversity, in AMBA the host-biting network presented a smaller number of mosquitoes in open green than in domestic environments, while that the number of vertebrate hosts was similar in the two. In Tandil, the host-biting network was composed for more mosquito species and hosts in domestic environments, while in open green it consisted only of one mosquito and three mammals. Connectance and H2 indices obtained for AMBA-open green and Tandil-domestic indicated the occurrence of aggregated patterns and suggested some degree of host selection; Aedes mosquitoes tend to feed on mammals, while Culex mosquitoes display opportunistic feeding patterns. Also, in both settings of AMBA, indices calculated at the species level indicated that Cx. quinquefasciatus was the least specialised mosquito, suggesting a generalist biting behaviour. In Tandil, a similar pattern was observed for Cx. apicinus which was more connected with different avian species. Generalist mosquitoes have special public health relevance, since they are more prone to act as bridge vectors for zoonotic infections by transmitting a pathogen from a reservoir host to other susceptible hosts (Hoyos et al., Reference Hoyos, Carrasquilla, León, Montgomery, Salyer, Komar and González2021). In this scenario, we hypothesise that the host-biting network at each setting and urbanisation is mainly determined by the composition of the mosquito community, which is in turn influenced by the diversity of available aquatic habitats (e.g. type and stability of water bodies and mosquito oviposition strategies; Cardo et al., Reference Cardo, Vezzani and Carbajo2011, Reference Cardo, Vezzani and Carbajo2012). Although blood- and oviposition-seeking mosquitoes often fly far distances (5–10 km) when they look for a potential host or larval habitat (Charlwood et al., Reference Charlwood, Graves and de Marsharll1988; Goodman et al., Reference Goodman, Egizi, Fonseca, Leisnham and LaDeau2018), a blood meal imposes a heavy burden on a mosquito in terms of its dispersal ability; therefore, after feeding, specimens typically remain within 1 km from their blood source (Orsborne et al., Reference Orsborne, Furuya-Kanamori, Jeffries, Kristan, Mohammed, Afrane, O'Reilly, Massad, Drakeley, Walker and Yakob2019; Hernandez-Colina et al., Reference Hernandez-Colina, Gonzalez-Olvera, Lomax, Townsend, Maddox, Hesson, Sherlock, Ward, Eckley, Vercoe, Lopez and Baylis2021).
Limitations of this study include, first, the low number of mammalian feeds identified due to the lack of amplification of blood meals by Aedes species in AMBA. This could be due to inadequate handling of mosquitoes during laboratory procedures and prolonged storage times that could affect the performance of the PCR. Second, this study utilised all mosquitoes whose abdomens contained any amount of blood. Mosquitoes collected in AMBA had Sella indices ranging from II to V, indicating varying amounts of blood in the abdomens, while most mosquitoes captured in Tandil had a full abdomen (Sella Index II). It has been reported that the Sella Index is significantly correlated with amplification success (Cardo et al., Reference Cardo, Carbajo, Mozzoni, Kliger and Vezzani2023). Finally, the small numbers of engorged mosquito monthly captured preclude any seasonal comparison, which could be a key stone in the understanding of the transmission cycles. Despite these caveats, our results provide valuable insights into mosquito host-feeding patterns in urban areas of temperate Argentina. A host-biting network approach for haematophagous insects and their vertebrate hosts can contribute to the understanding of the ecological dynamics of mosquito-borne diseases at the local scale, and can lead future epidemiological research and vector control strategies.
Competing interests
None.