Crassigyrinus scoticus was a large stem tetrapod from the coal swamps of the Carboniferous of Scotland. Based on its long body, reduced limbs, large size and large orbits, Crassigyrinus seems to have been fully aquatic (Panchen Reference Panchen1985). This contrasts with many other Carboniferous (and even late Devonian) tetrapods, which show changes in bone morphology that indicate shifts toward more weight-bearing limb functions and probably at least some modest degree of terrestrial locomotor abilities (Daeschler et al. Reference Daeschler, Shubin, Thomson and Amaral1994; Coates et al. Reference Coates, Jeffery and Ruta2002, Reference Coates, Ruta and Friedman2008; Clack Reference Clack2012; Pierce et al. Reference Pierce, Clack and Hutchinson2012; Smithson et al. Reference Smithson, Wood, Marshall and Clack2012). It has been suggested that Crassigyrinus either represents an ancestrally more aquatic side branch in early tetrapod evolution that remained aquatic or that Crassigyrinus became secondarily aquatic. It is difficult to test these hypotheses with the available Crassigyrinus material because of its aberrant mix of ancestral and derived features (see page 2), and the uncertainty of its phylogenetic position.
Whilst the aquatic locomotion of Crassigyrinus may have been retained from aquatic ancestors, the reduced forelimbs are not a plesiomorphic feature but rather an autapomorphy. The limbless aïstopods are another example of secondary limb reduction in early tetrapods. A recent phylogenetic analysis placed them deep on the tetrapod stem, suggesting that stem tetrapods exhibited a wider range of appendicular morphologies than was previously thought (Pardo et al. Reference Pardo, Szostakiwskyj, Ahlberg and Anderson2017). It is worth noting that the aïstopods share many features with Crassigyrinus, such as the long body, reduced limbs and large orbits (Anderson et al. Reference Anderson, Carroll and Rowe2003), all of which have been used to support the hypothesis that Crassigyrinus was aquatic. However, unlike Crassigyrinus, the aïstopods are thought to have been terrestrial (Germain Reference Germain2008). Their smaller body size relative to Crassigyrinus might have enabled them to move on land despite having reduced limbs. It has become clear that early tetrapods were a morphologically, functionally and ecologically disparate group of organisms. Here, we sought to gain insight into the morphology of Crassigyrinus in this context of disparity among stem tetrapods.
Skeletal material of Crassigyrinus scoticus has been found in late Viséan (∼330-million-year-old) and early Namurian (∼326-million-year-old) deposits of Gilmerton and Cowdenbeath, Scotland, although a new Crassigyrinus-like partial jaw UMZC (University Museum of Zoology, Cambridge) 2011.9.1 from Burnmouth, Scotland, might extend the temporal range of Crassigyrinus back into the Tournasian (Smithson et al. Reference Smithson, Wood, Marshall and Clack2012; Clack et al. Reference Clack, Porro and Bennett2018). Table 1 provides an overview of Crassigyrinus specimens and institutional abbreviations. The Gilmerton Ironstone specimens are all cranial material and Crassigyrinus was the most common tetrapod collected at this site. Godfrey (Reference Godfrey1988) also tentatively attributed a ribcage and ventral scales (CMNH (Cleveland Museum of Natural History) 11230) from the Viséan of Greer, West Virginia, to Crassigyrinus, but we think this identification is unlikely because the scales from Greer (Godfrey Reference Godfrey1988, fig. 7) are much more rounded at one end than the narrow scales of Crassigyrinus. The first known postcranial material of Crassigyrinus is the NHMUK (Natural History Museum, London) VP R10000 specimen, which was discovered in the Dora bonebed from the Namurian of Cowdenbeath, Fife. Further Crassigyrinus material (NMS G (National Museums of Scotland, Department of Geology, Edinburgh) 1975.5.5, NMS G 1984.15.1-3), including the only hindlimb bones discovered, was reported from the same site by Panchen & Smithson (Reference Panchen and Smithson1990). The minimum number of individuals is two, because there are two left ischia and two right ilia (duplicate elements in NMS G 1984.15.3 and NMS G 1975.5.5).
Table 1 Specimens attributed to Crassigyrinus. Abbreviations: CMNH=Cleveland Museum of Natural History; GSE=British Geological Survey, Edinburgh; NEWHM=Newcastle Hancock Museum, Newcastle upon Tyne; NHMUK VP=Natural History Museum, Department of Vertebrate Palaeontology, London (formerly the British Museum); NMS G=National Museums of Scotland, Department of Geology, Edinburgh (formerly the Royal Scottish Museum); UMZC=University Museum of Zoology, Cambridge.

*Identification as Crassigyrinus is doubtful.
In his analysis of the NHMUK VP (Natural History Museum, Department of Vertebrate Palaeontology, London) R10000 Crassigyrinus specimen, Panchen (Reference Panchen1985) noted the mosaic of plesiomorphic and derived features. Panchen judged the autapomorphies of Crassigyrinus to be the large quadrangular orbits, strongly constricted frontals and parietals, deep jugals and lacrimals and the reduced forelimbs (Panchen Reference Panchen1985). The morphology of the palate shows similarities to tetrapodomorph fish, but the presence of tabular horns and a large intertemporal bone are more characteristic of anthracosaurian tetrapods. The latter was thought by Panchen to be an early tetrapod plesiomorphy retained in anthracosaurs but reduced or lost in most temnospondyls. However, it is now thought that the large intertemporal may have evolved independently in various post-Devonian tetrapod clades, as this trait is absent in earlier tetrapods such as Ichthyostega and Acanthostega (Clack Reference Clack1998). Panchen (Reference Panchen1985) determined the large supratemporal sutured to the postparietal to be an ancestral character for tetrapods, also retained in temnospondyls but differing from the anthracosaur arrangement. After considering these contradicting characters, Panchen (Reference Panchen1985) proposed Crassigyrinus to be a sister taxon to anthracosaurs, based on four synapomorphies: tooth morphology, dermal ornamentation, tabular horn and lack of post-temporal fossae.
The phylogenetic analysis of Lebedev & Coates (Reference Lebedev and Coates1995), which placed Crassigyrinus in a polytomy with Tulerpeton, Proterogyrinus and Westlothiana, demonstrated the uncertainty in the phylogenetic position of Crassigyrinus that persists today. Lombard & Bolt (Reference Lombard and Bolt1995), in an analysis including Whatcheeria, placed Crassigyrinus directly stemward of [Whatcheeria + Anthracosauria]. Coates (Reference Coates1996) found Crassigyrinus to be directly crownward of Whatcheeria and sister to [Westlothiana + (Proterogyrinus+Archeria)], but noted the weak support for this node. Clack's (Reference Clack1998) analysis based on a redescription of cranial material concluded that Crassigyrinus and Whatcheeria are sister taxa, forming a sister clade to the anthracosaur Proterogyrinus. However, Clack (Reference Clack1998) noted the instability of this node, because these taxa only share two synapomorphies. The first synapomorphy is double tabular facets for the braincase (no fossil evidence exists for Whatcheeria, but this character was reconstructed by the phylogenetic algorithms to be present). The second synapomorphy is a single median parasphenoid depression.
Recent discoveries of early tetrapods have increased our understanding of their diversity. However, despite the new discoveries and tools enabling large-scale analyses, the position of Crassigyrinus remains unresolved (Fig. 1). In different phylogenetic analyses, Crassigyrinus has shifted between being the sister taxon of Whatcheeria (Ruta et al. Reference Ruta, Coates and Quicke2003: reweighted analysis), being directly stemward of Whatcheeria and Pederpes (Ruta et al. Reference Ruta, Coates and Quicke2003; Ruta & Coates Reference Ruta and Coates2007) or being directly crownward of Whatcheeria and Pederpes (Clack Reference Clack2002; Ruta & Coates Reference Ruta and Coates2007: reweighted analysis; Pardo et al. Reference Pardo, Szostakiwskyj, Ahlberg and Anderson2017). In the Ruta & Coates Reference Ruta and Coates2007 analysis, Ossinodus is the sister group of Whatcheeria and Pederpes, but none of the other analyses corroborated this relationship. A recent study including new Tournasian taxa suggested that Whatcheeria and Pederpes are not the closest relatives of Crassigyrinus (Clack et al. Reference Clack, Bennett, Carpenter, Davies, Fraser, Kearsey, Marshall, Millward, Otoo, Reeves, Ross, Ruta, Smithson, Smithson and Walsh2016). However, depending on the type of analysis used, the closest relative of Crassigyrinus shifted and there was no consistent relationship with any of the new taxa. Even with an increase in the number of characters and taxa in datasets over time, there is no clear trend towards one of the above phylogenetic hypotheses. Furthermore, the position of Crassigyrinus is dependent on the characters used (see Clack & Finney (Reference Clack and Finney2005) for a comparison of the datasets used by Clack (Reference Clack2002) and Ruta et al. (Reference Ruta, Coates and Quicke2003)).

Figure 1 Summary of several phylogenetic analyses, demonstrating that the position of Crassigyrinus remains unresolved. Phylogenies adapted from the respective papers. (a) Crassigyrinus more crownward than [Whatcheeria + Pederpes] (Clack Reference Clack2002). (b1) Crassigyrinus more stemward than [Ossinodus+(Whatcheeria + Pederpes)]. (b2) (reweighted by consistency index) Crassigyrinus more crownward than [Pederpes+Whatcheeria] (Ruta & Coates Reference Ruta and Coates2007). (c) Crassigyrinus more crownward than [Whatcheeria+Pederpes], more closely related to other early tetrapods, relationships are dependent on type of analysis (c1: single most parsimonious tree; c2: strict consensus; c3: Bayesian) (Clack et al. Reference Clack, Bennett, Carpenter, Davies, Fraser, Kearsey, Marshall, Millward, Otoo, Reeves, Ross, Ruta, Smithson, Smithson and Walsh2016). (d) Crassigyrinus more crownward than [Pederpes + Whatcheeria] (Pardo et al. Reference Pardo, Szostakiwskyj, Ahlberg and Anderson2017).
The morphology of the Dora specimens (NHMUK R10000, NMS G 1975.5.5 and NMS G 1984.15.1-3) remains incompletely studied, because the specimens are buried in slabs of rock, and not all bones have been prepared out. In NHMUK VP R10000, extensive scales and other bones on the surface cover the rest of the skeleton, so manual preparation would destroy the depositional context of the fossil and risk damaging it. Here, we used computed tomographic (CT) scanning as a non-destructive method to further examine the postcranial morphology of Crassigyrinus scoticus. CT scans have revealed previously hidden bones in early tetrapods. For example, an ulna and possible sternebrae were discovered in Ichthyostega (Pierce et al. Reference Pierce, Clack and Hutchinson2012, Reference Pierce, Ahlberg, Hutchinson, Molnar, Sanchez, Tafforeau and Clack2013), an atlas–axis complex was discovered in the stem amniote Orobates pabsti (Nyakatura et al. Reference Nyakatura, Allen, Lauströer, Andikfar, Danczak, Ullrich, Hufenbach, Martens and Fischer2015) and an entirely new tetrapod species, Aytonerpeton microps, was discovered by chance from micro-CT scans of a lungfish specimen (Clack et al. Reference Clack, Bennett, Carpenter, Davies, Fraser, Kearsey, Marshall, Millward, Otoo, Reeves, Ross, Ruta, Smithson, Smithson and Walsh2016). New material could provide more (or refined) characters for phylogenetic analysis. Finally, new discoveries can be analysed in a comparative framework to investigate the morphological disparity present in tetrapods during the Carboniferous. Why did some animals remain aquatic, or become secondarily aquatic, while their contemporaries became increasingly terrestrial? The aim of our study is to help fill in the gaps in our understanding of the palaeobiology of the enigmatic tetrapod Crassigyrinus by contributing new data on its morphology.
1. Materials and methods
We scanned all available postcranial material of Crassigyrinus scoticus, including manually prepared elements and pieces of slab containing fossil material (Table 2). For the NMS G 1984.15.1-3 and NMS G 1975.5.5 specimens, a micro-CT scanner was used to obtain high-resolution scans. However, of the NHMUK VP R10000 slab, only two small slab pieces and the manually prepared elements could be scanned with a micro-CT scanner. The main slab had to be scanned with a regular medical CT scanner, since it was too large to fit in a micro-CT scanner, but these scans yielded no new information because of their poor resolution. Bones from the micro-CT scans were segmented in Mimics 19.0 (Materialise Inc., Leuven, Belgium) software to remove the matrix and create a three-dimensional (3D) model of each bone (Fig. 2). CT image stacks and final 3D models are available on Figshare (https://figshare.com/projects/Crassigyrinus_stem_tetrapod_fossil_CT_scan_data/38249).
Table 2 Crassigyrinus scan information. Abbreviations: NHMUK VP=Natural History Museum, Department of Vertebrate Palaeontology, London (formerly the British Museum); NMS G=National Museums of Scotland, Department of Geology, Edinburgh (formerly the Royal Scottish Museum); CT=computed tomography.
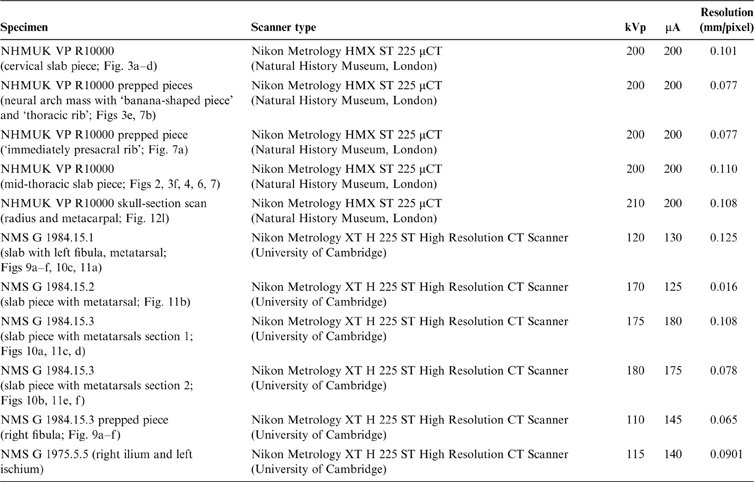
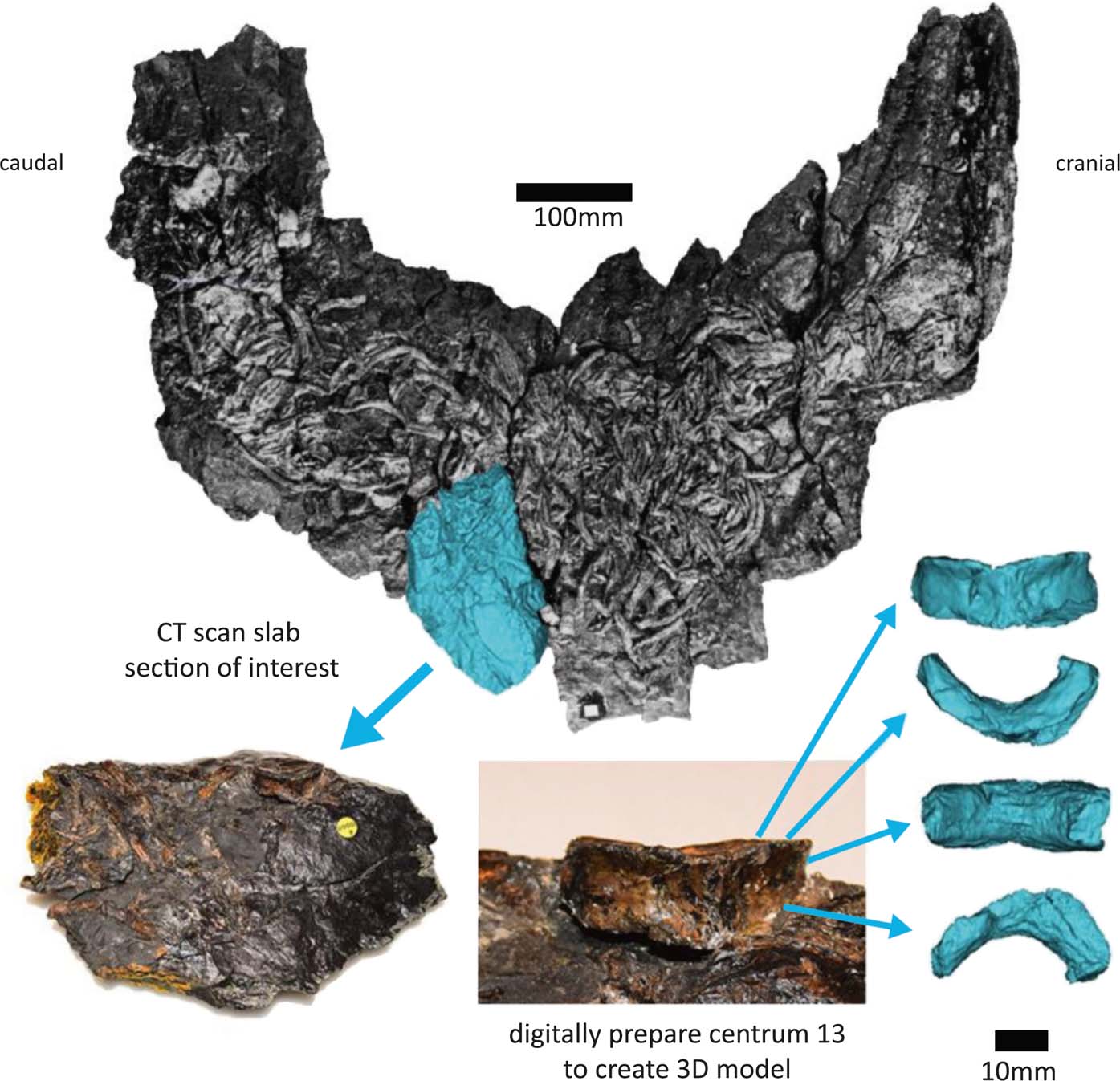
Figure 2 Segmentation process of centrum 13. Model created from segmented CT scans in blue. Full specimen photo from Panchen (Reference Panchen1985).
2. Results and discussion
The digital preparation revealed previously undiscovered bones in both the NHMUK VP R10000 and the NMS G 1984.15.1-3 and NMS G 1975.5 specimens (Table 3), as described in more detail in the following sections.
Table 3 New material found through segmentation of CT scans. Abbreviations: NHMUK VP=Natural History Museum, Department of Vertebrate Palaeontology, London (formerly the British Museum); NMS G=National Museums of Scotland, Department of Geology, Edinburgh (formerly the Royal Scottish Museum); CT=computed tomography.

1 Discovered by Panchen (Reference Panchen1985) (bones partially exposed on slab surface) but greater detail revealed by segmentation.
2 Bone previously described by Panchen & Smithson (Reference Panchen and Smithson1990), but identified as left sacral rib.
2.1. Axial skeleton
2.1.1. Possible pleurocentra
Small boat- or wedge-shaped elements were found in the NHMUK VP R10000 specimen (Fig. 3). We tentatively identify these elements as pleurocentra, although they might also be fragments of other bones. Panchen (Reference Panchen1985) did not note the presence of pleurocentra; however, he did refer to a small ‘banana-shape' piece of bone (Figs 3e, 4f) that could potentially be a pleurocentrum. Yet, in the absence of any other similar bones, he concluded it was more likely to be a fragment of the apex of an intercentrum, and described Crassigyrinus as being monospondylous.
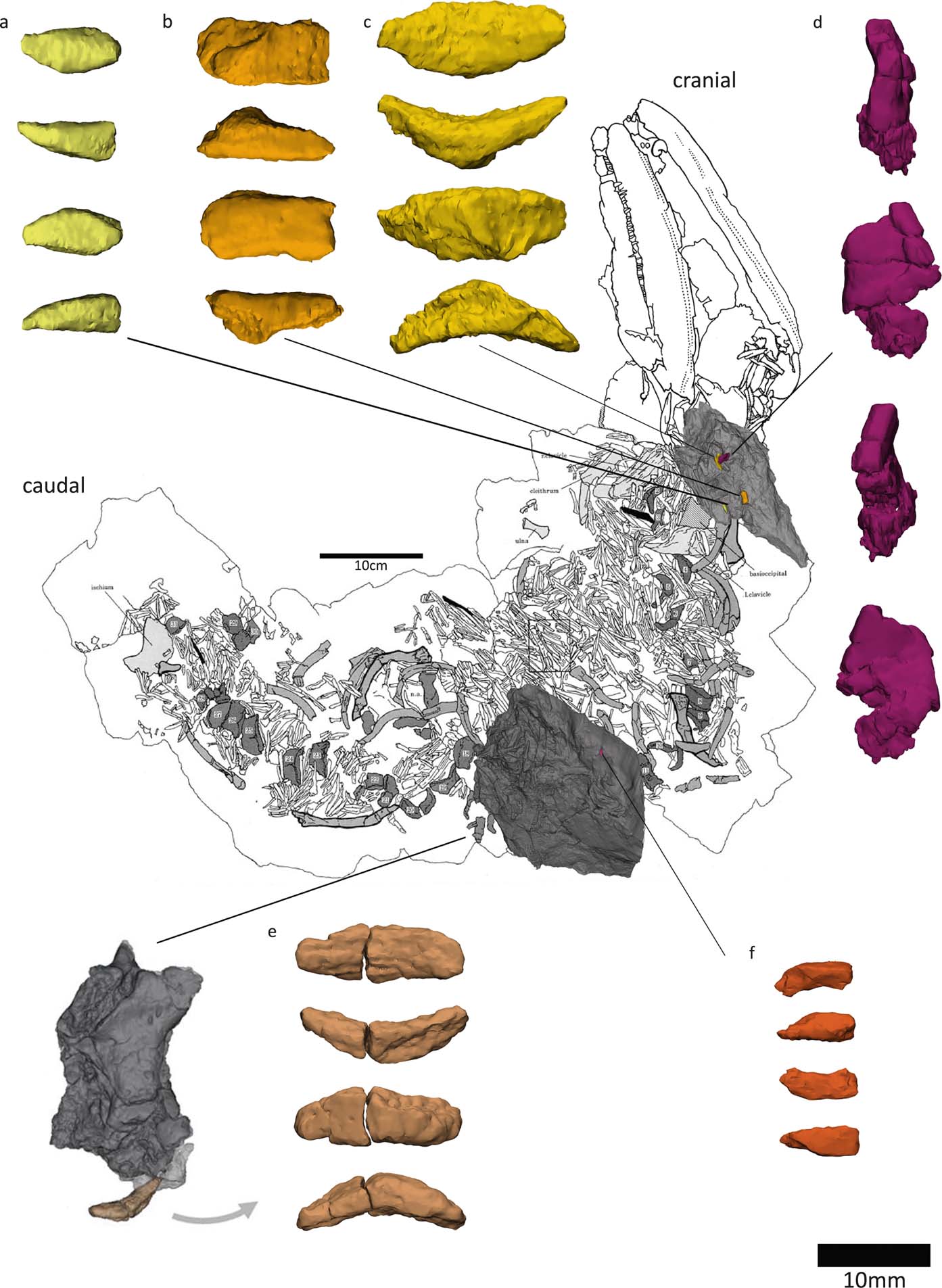
Figure 3 Elements (a–f) discovered from micro-CT scans of two slab sections of the NHMUK VP R10000 specimen, one from the cervical region and the other from the mid-thoracic region. Line drawing from Panchen (Reference Panchen1985).
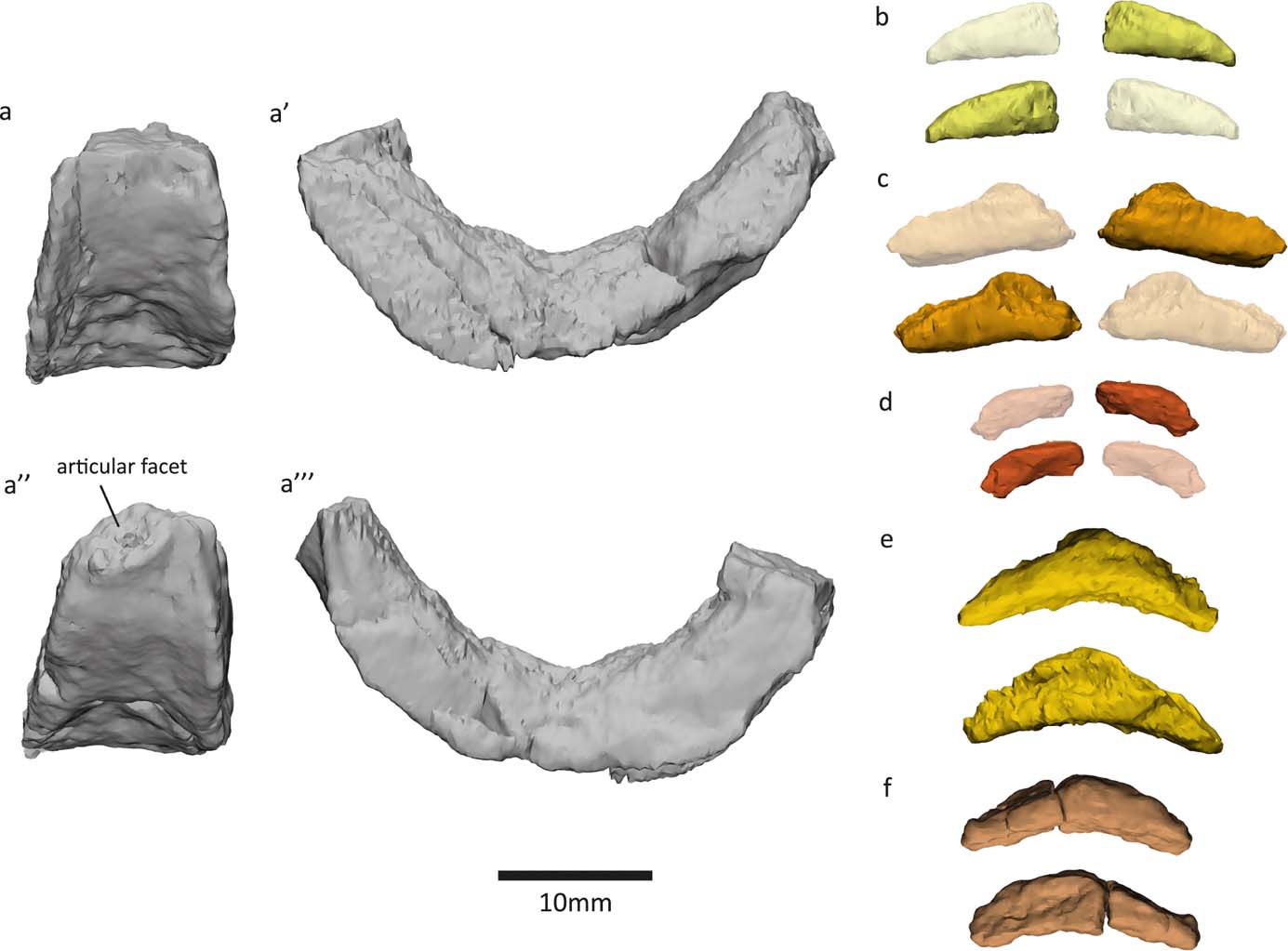
Figure 4 New elements discovered in the NHMUK VP R10000 specimen. (a) Centrum 13 (Panchen Reference Panchen1985). (b, d–f) Possible pleurocentra. (c) Probably an intercentrum fragment.
Digital segmentation of the ‘banana-shaped' piece has clarified its morphology, and we discovered another bone that could potentially be a serial homologue of this element. This element (Figs 3c, 4e) is slightly smaller than Panchen's (Reference Panchen1985) putative pleurocentrum, which is in agreement with its more anterior location along the vertebral column, near the skull (compared to Panchen's piece, which was found near intercentrum 17). We also discovered two additional elements that, in size and morphology, conceivably are pleurocentrum ossification centres that did not fuse in the midline (Figs 3a, f, 4b, d). The apparent scarcity of these elements may be a preservational artefact, but it is also worth noting that ossified pleurocentra are not necessarily present in every vertebral element. For example, it appears that Acanthostega only had pleurocentra from the eighth presacral to around the 22nd caudal vertebra (Coates Reference Coates1996), and in Ichthyostega it appears that the anterior thoracic pleurocentra ossified after the posterior ones (Pierce et al. Reference Pierce, Ahlberg, Hutchinson, Molnar, Sanchez, Tafforeau and Clack2013).
If these elements are indeed pleurocentra, it is unclear whether the elements in Figure 3c and 3e are each two ossifications that have fused in the midline, or whether they each represent one ossification centre. Danto et al. (Reference Danto, Witzmann, Pierce and Fröbisch2017) provided a valuable overview of pleurocentra and intercentra morphology in early tetrapods, noting that in stem-tetrapods and temnospondyls, the pleurocentra ossify from two dorsal ossification centres which sometimes fuse in the midline. Ontogenetic variation in the degree of ossification of pleurocentra has been reported in Proterogyrinus (Holmes Reference Holmes1984) and the seymouriamorph Discosauriscus (Klembara & Bartik Reference Klembara and Bartik1999). In addition to ontogenetic factors, and more relevant to our analysis of the NHMUK VP R10000 specimen, there can also be regional variation in the degree of fusion in a single individual. For example, Osteolepis exhibits such change along the vertebral column, with the anterior pleurocentra being a single piece and the mid-trunk pleurocentra being paired (Panchen Reference Panchen, Andrews, Miles and Walker1977). Intercentra fuse from two ossification centres, and different stages of fusion can be observed in the growth series of Greererpeton intercentra (Godfrey Reference Godfrey1989). The intercentra of Crassigyrinus also change in the degree of coossification along the vertebral column – the anterior intercentra have a midline constriction that indicates coossification of bilateral halves, but such a constriction is not visible in the posterior intercentra (Panchen Reference Panchen1985). It is worth noting, however, that the pleurocentra of Crassigyrinus do not display a correlation between the degree of fusion and apparent position along the vertebral column. For example, in a slab section near the skull, we found both a bilateral half and a potentially fused pleurocentrum (Fig. 3). This could be due to taphonomic disturbance, as none of the pleurocentra were found in articulation with any other elements. Another explanation is a high degree of plasticity in the amount of fusion of pleurocentra, unrelated to position. The elements we discovered are cautiously interpreted as pleurocentra, but it remains unclear if the two ossification centres fused in the midline in some pleurocentra.
If these additional elements that we discovered are indeed pleurocentra, Crassigyrinus was diplospondylous (possessing both intercentra and pleurocentra) instead of monospondylous (having a single repeated centrum down the length of the vertebral column). Crassigyrinus could have had rhachitomous vertebrae (Fig. 5c), in which there is an anterior, large horseshoe-shaped intercentrum ventral to the notochord and a pair of smaller pleurocentra dorsal to the notochord and posterior to the intercentrum (shown in Eryops in Fig. 5a). A rhachitomous vertebral pattern in present in the whatcheerids Whatcheeria and Pederpes (Lombard & Bolt Reference Lombard and Bolt1995; Clack & Finney Reference Clack and Finney2005), to which Crassigyrinus has been proposed to be closely related (but see page 3 about the instability of this relationship). In Whatcheeria, fusion of the paired pleurocentra was noted in the lumbar region of a specimen (Lombard & Bolt Reference Lombard and Bolt1995). No such fusion is present in Pederpes (Pierce et al. Reference Pierce, Ahlberg, Hutchinson, Molnar, Sanchez, Tafforeau and Clack2013). In shape, the intercentra and some putative pleurocentra (Figs 3c, e, 4e, f) of Crassigyrinus look similar to those of Pederpes (Pierce et al. Reference Pierce, Ahlberg, Hutchinson, Molnar, Sanchez, Tafforeau and Clack2013), although the pleurocentrum size, relative to intercentrum size, is smaller in Crassigyrinus (Fig. 4). Furthermore, the Crassigyrinus intercentrum is a more slender wedge shape in lateral view than the intercentra of Pederpes. Note that the left dorsal edge of centrum 13 (Fig. 4a) has been sheared off, so the morphology is distorted. The right side of centrum 13, and centra 14 and 17 (Panchen Reference Panchen1985, fig. 17b, c) show the tapering dorsal edge.

Figure 5 Vertebral patterns. (a) Eryops with typical rhachitomous pattern (based on Moulton Reference Moulton1974). (b) Proterogyrinus with gastrocentrous pattern (based on Holmes Reference Holmes1984). (c) Inferred rhachitomous pattern for Crassigyrinus. Abbreviations: PC=pleurocentrum; IC=intercentrum.
The putative pleurocentra of Crassigyrinus differ from those of Whatcheeria in that the latter have large anterior facets and small posterior facets for articulation with the neural arches (Lombard & Bolt Reference Lombard and Bolt1995), a morphology which is also found in Eryops (Moulton Reference Moulton1974). No facets are visible in the Crassigyrinus pleurocentra, except maybe the slight depression seen in Figure 3f. This piece looks similar to the Eryops pleurocentra in overall shape (Moulton Reference Moulton1974, fig. 13d). A rhachitomous pattern in Crassigyrinus is conceivable, because in this case the intercentra would hide the pleurocentra if the vertebral column is viewed from the ventral side, which is the side that is exposed in the NHMUK VP R1000 specimen. We rule out a gastrocentrous vertebral pattern, such as in Proterogyrinus (Fig. 5b; Holmes Reference Holmes1984), because in this pattern the pleurocentra are positioned ventrally, and no pleurocentra are visible between the intercentra found in articulation on the exposed surface of the NHMUK VP R1000 slab. In Ichthyostega, the ‘reverse' rhachitomous vertebral pattern was not identified until the material was micro-CT and synchrotron scanned by Pierce et al. (Reference Pierce, Ahlberg, Hutchinson, Molnar, Sanchez, Tafforeau and Clack2013). The main argument against the identification of the new Crassigyrinus elements as pleurocentra became apparent with the discovery of element b in Fig. 3 (c in Fig. 4). We initially interpreted this to be another contender as a pleurocentrum piece, and it even bears a large facet. However, upon comparison with the intercentra, we realised that the facet shape is exactly like the intercentrum facet, and this piece is probably the broken dorsal edge of an intercentrum. We tested an articulation with the broken edge of centrum 13 (Fig. 4a), and while the fit was not exact, it confirmed that element b in Fig. 3 (c in Fig. 4) is likely to be part of an intercentrum. The lack of an obvious fracture line led us to reconsider the other elements. Our identification of them as pleurocentra rested, in part, on the absence of a shear line, so we deemed them complete; but we think it is also possible that they are fractured pieces, hence our caution here. Note that another element in Figure 3, element d (Fig. 3d), was found near element c (Fig. 3c), but is definitely not a pleurocentrum. It could be a distorted neural arch, but looks most like a rib-end fragment.
2.1.2. Discussion of vertebral pattern
Early tetrapods exhibit several different arrangements of centra (Pierce et al. Reference Pierce, Ahlberg, Hutchinson, Molnar, Sanchez, Tafforeau and Clack2013; Danto et al. Reference Danto, Witzmann and Fröbish2016, Reference Danto, Witzmann, Pierce and Fröbisch2017), and the ancestral pattern is unclear. Eusthenopteron, Whatcheeria and Acanthostega were described as having rhachitomous vertebrae (Andrews & Westoll Reference Andrews and Westoll1970; Lombard & Bolt Reference Lombard and Bolt1995; Coates Reference Coates1996). This arrangement was long thought to be the ancestral condition (Romer Reference Romer1960; Carroll Reference Carroll1988; but see Panchen (Reference Panchen, Andrews, Miles and Walker1977) for a discussion of alternate hypotheses, and Gardiner (Reference Gardiner1983) for an overview of hypotheses of vertebral homologies). However, a recent micro-CT analysis of Ichthyostega and Acanthostega revealed a ‘reverse rhachitomous' pattern, in which the paired pleurocentra are fused to or articulate with the intercentrum posterior to them (Ichthyostega and Acanthostega, respectively) (Pierce et al. Reference Pierce, Ahlberg, Hutchinson, Molnar, Sanchez, Tafforeau and Clack2013). Such a ‘reverse rhachitomous' pattern is not unusual in early tetrapods; ‘reverse rhachitomous' fusion is also found in Whatcheeria (Lombard & Bolt Reference Lombard and Bolt1995) as well as a range of other tetrapods such as Dvinosaurus, Platyops and Dendrerpeton (Shishkin Reference Shishkin1989). Even in Eryops, which is often used as an example of a typical rhachitomous pattern, the pleurocentra coossified with the intercentrum posterior to them in one specimen (Moulton Reference Moulton1974).
Vertebral arrangement has historically been used as a characteristic to diagnose taxa (van Zittel Reference von Zittel1911; Watson Reference Watson1919; Romer Reference Romer1960). This is also evident in the names of groups such as Embolomeri, Stereospondyli and Phyllospondyli, all of which are based on vertebral pattern (Holmes Reference Holmes1989). Romer approached the question of amphibian relationships by examining vertebral patterns. He divided Amphibia into two main groups: the Lepospondyli (including fossil lepospondyls and the extant lissamphibians Urodela and Apoda) and the Apsidospondyli (including the ‘Labyrinthodontia' and the amniotes and Anura) (Romer Reference Romer1960). However, caution is warranted when making phylogenetic inferences based on vertebral element morphology alone. A recent histology study by Danto et al. (Reference Danto, Witzmann and Fröbish2016) demonstrated that patterns of vertebral development have a weak phylogenetic signal, and phylogeny is not always a reliable indicator of vertebral pattern. For example, based on phylogenetic position, we would expect the Plagiosauridae to possess a large disc-shaped intercentrum with reduced or absent pleurocentra, like other members of Stereopondyli. Instead, the Plagiosauridae possess a single spool-shaped centrum, and the neural arch is positioned between two centra (Danto et al. Reference Danto, Witzmann and Fröbish2016).
Panchen proposed that in seymouriamorphs, anthracosaurs (except embolomeres), reptiles and microsaurs, the pleurocentrum is large relative to the intercentrum because it, and the neural arch it is associated with, supports the vertebral column during weight-bearing (Panchen Reference Panchen, Andrews, Miles and Walker1977). Temnospondyls, on the other hand, have a larger intercentrum, the centrum most closely linked with the myoseptum and ribs. This arrangement would be more suited to support lateral movement during swimming (Panchen Reference Panchen, Andrews, Miles and Walker1977). Clack (Reference Clack2012) discussed two examples that corroborate Panchen's idea about vertebral function: the secondarily aquatic embolomeres, such as Archeria, increase the size of the intercentrum, and the more aquatic of the temnospondyls, such as Mastodonsaurus (Moser & Schoch Reference Moser and Schoch2007), reduce the size of their pleurocentrum – in both cases, the more aquatic environment correlates with a trend to increase the size of the intercentrum, which often becomes a complete disc.
However, Clack (Reference Clack2012) noted that rhachitomous vertebrae do not necessarily imply aquatic locomotion. For example, Eryops, interpreted to be capable of at least some degree of terrestrial locomotion, has rhachitomous vertebrae (Clack Reference Clack2012). Furthermore, not all aquatic organisms are rhachitomous (see discussion of embolomeres and nectrideans in Carroll (Reference Carroll1988)). There may also be a size effect. Danto et al. (Reference Danto, Witzmann and Fröbish2016) discussed the spool-shaped centra in the miniaturised Microbrachis and Doleserpeton. These two taxa are not closely related and they are thought to be aquatic and terrestrial, respectively. Therefore, because phylogeny and environment are controlled for, Danto et al. (Reference Danto, Witzmann and Fröbish2016) concluded that the spool-shaped centra are associated with small body size. Vertebral pattern appears to be a plastic trait influences by various factors that also include ontogeny.
If the elements we discovered are really pleurocentra, and Crassigyrinus is rhachitomous, this does not inform the phylogenetic placement of Crassigyrinus, because the rhachitomous pattern is plesiomorphic for tetrapods. Furthermore, the pieces are very small, so they are unlikely to have constricted the notochord much more than the much larger intercentrum and neural arch already did.
2.1.3. Neural arch
We discovered a neural arch in the slab piece near centrum 13 of the NHMUK VP R10000 specimen (Fig. 6). All neural arches found are bilateral halves, with no midline fusion. This contrasts with Whatcheeria, in which all neural arches are fused (Lombard & Bolt Reference Lombard and Bolt1995). In Pederpes, at least some neural arch halves were probably unsutured (Clack & Finney Reference Clack and Finney2005). In Acanthostega, only a region of the vertebral column, from the fourth presacral to about the 22nd caudal vertebra, shows fused neural arch pairs (Coates Reference Coates1996). The neural arches are poorly ossified. Based on the structure of the neural arch and intercentrum, it appears that the notochordal and neural canals were connected, which is also evident in Acanthostega (Coates Reference Coates1996).

Figure 6 Newly discovered half of neural arch in (a) anterior, (b) medial, (c) posterior and (d) lateral views.
The transverse process of the neural arch projects at almost a right angle to the dorsoventral axis of the spine. This also holds true for the most well-preserved neural arch from this specimen (neural arch 14 in Panchen Reference Panchen1985, fig. 17g), so it is unlikely to be a preservational artefact. Neural arch 14 and the new neural arch were found in close proximity to each other, so this morphology seems to be present in the mid-thoracic area of the vertebral column of Crassigyrinus. However, there may be regional variation, because the NMS G 1984.15.3 neural arch (Panchen & Smithson Reference Panchen and Smithson1990, fig. 8f) projects more ventrally.
The transverse process of the new neural arch projects extremely laterally, relative to the more ventrally facing transverse processes in Acanthostega, Ichthyostega and Pederpes (Pierce et al. Reference Pierce, Ahlberg, Hutchinson, Molnar, Sanchez, Tafforeau and Clack2013). However, the transverse processes of the posterior thoracic vertebrae of Ichthyostega face laterally (Pierce et al. Reference Pierce, Ahlberg, Hutchinson, Molnar, Sanchez, Tafforeau and Clack2013), similar to those in Crassigyrinus. Smithson (Reference Smithson1985) also reported varying orientations for the transverse processes of Eoherpeton, pointing either dorsolaterally or ventrolaterally in anterior view. He interpreted the latter to come from a more posterior position, because both Eryops (Moulton Reference Moulton1974) and probably also Eogyrinus (Panchen Reference Panchen1966) exhibit an anteroposterior shift from lateral to ventrolaterally oriented transverse processes along the vertebral column. Note, this is slightly different from Ichthyostega where the transverse processes of the anterior thoracic vertebrae also face ventrally (Pierce et al. Reference Pierce, Ahlberg, Hutchinson, Molnar, Sanchez, Tafforeau and Clack2013).
There is a small postzygapophysis and a small prezygapophysis. As in the Crassigyrinus neural arch 14 and 17 (Panchen Reference Panchen1985, figs. 17g, 18b), the postzygapophysis is an uneven posterior section of bone (Smithson & Clack Reference Smithson and Clack2018).
2.1.4. Ribs
We discovered several ribs in the NHMUK VP R10000 specimen, near centrum 13 (Fig. 7). Only one of these ribs was figured in Panchen's (Reference Panchen1985) illustration of the entire specimen, and none were prepared out until now. Many of the newly recognised ribs are at the edge of the slab and may be broken. However, the distal shaft ends of several of the ribs (see Fig. 7) look similar and do not appear to be fractured, so it seems that these ribs might be complete (although it is possible that the shaft continued as a cartilaginous extension, as has been proposed for ribs of Eryops (Moulton Reference Moulton1974)).

Figure 7 Crassigyrinus rib morphology. (a) Panchen's (Reference Panchen1985) ‘immediately presacral rib'. (b) ‘Thoracic rib'. (c–f) New ribs discovered in NHMUK VP R10000. (g) Closeup of the most complete new rib, with breakages mended. Based on comparative material, the views are most likely: (c) anterior; (d) distal; (e) posterior; (f) proximal.
Furthermore, we investigated the fossil as well as the lower-resolution medical CT scans of the rest of the NHMUK VP R10000 specimen, and no ribs have two expanded ends, so they are either all broken, or the ribs are complete and lack a distal expansion. For example, rib g (Fig. 7g) tapers to such a rod-like point, and has only a slight curvature. It is very similar to the posterior thoracic rib of Ossinodus. The posterior thoracic rib of Ossinodus, which was described as being complete, terminates distally without any flaring (Warren & Turner Reference Warren and Turner2004, fig. 8k–n). Rib ends that are rod-like and do not have a distal expansion are also known from the thoracic region of Proterogyrinus, Colosteus and Ossinodus (Hook Reference Hook1983; Holmes Reference Holmes1984; Warren & Turner Reference Warren and Turner2004). This shape supports our interpretation that Crassigyrinus ribs did not have flared distal ends, except for possibly the ‘thoracic rib' described by Panchen (Reference Panchen1985), but no other such rib has been found.
All newly recognised ribs have a crest on one side of the proximal end and a concave depression on the other. The crest is also visible in Ossinodus, Proterogyrinus and Eoeherpeton (Holmes Reference Holmes1984; Smithson Reference Smithson1985; Warren & Turner Reference Warren and Turner2004). The smaller, more triangular ribs resemble a rib tentatively identified by Panchen as being an immediately presacral rib (Panchen Reference Panchen1985, fig. 19b; Fig. 7a). These ribs also look similar to the posterior trunk ribs in Acanthostega (Coates Reference Coates1996, fig. 10g, h). In Crassigyrinus, these short, almost triangular ribs are preserved near centra 13–16, in the mid-trunk region of the animal, although this location could be due to taphonomic displacement, as no ribs are found in articulation with vertebrae, and several rib morphologies were found in this slab section. Regardless of their location along the vertebral column, there was some amount of variation in the ribs of Crassigyrinus (Fig. 7), although the proximal ends all look similar, and only the length of the shaft seems to vary. A greater amount of rib variation along the vertebral column has been described in several early tetrapods, such as Proterogyrinus, Pederpes, Acanthostega and Ichthyostega (Holmes Reference Holmes1984; Coates Reference Coates1996; Jarvik Reference Jarvik1996; Clack & Finney Reference Clack and Finney2005).
Coates (Reference Coates1996) noted that regional variation in rib morphology is not only present in early tetrapods but also in the tetrapodomorph Eusthenopteron, in which the anterior 3 ribs are much shorter and broader than the more posterior ribs. The ribs of Tiktaalik also show regional variation: most ribs have plate-shaped flanges that project caudally, but the flanges become triangular in the posterior ribs (which are also shorter), and the anterior-most ribs have uncinate processes projecting cranially from the shaft (Daeschler et al. Reference Daeschler, Shubin and Jenkins2006).
In all discovered ribs, the proximal end is spatulate and does not bear two distinct rib heads. This is similar to the rib-head shapes in many early tetrapods, such as Acanthostega, Pederpes, Ichthyostega and Ossinodus (Coates Reference Coates1996; Jarvik Reference Jarvik1996; Warren & Turner Reference Warren and Turner2004; Clack & Finney Reference Clack and Finney2005). The ribs of Crassigyrinus also resemble those of Acanthostega in the lack of strong ventral curvature or uncinate processes (Coates Reference Coates1996). Uncinate processes or strongly developed mid-shaft flanges are present on the thoracic ribs of Whatcheeria and Ichthyostega, and these flanges are located more distally in the posterior ribs (Lombard & Bolt Reference Lombard and Bolt1995; Ahlberg et al. Reference Ahlberg, Clack and Blom2005). The only expanded distal end in Crassigyrinus is seen in Panchen's ‘thoracic' rib, which resembles Pederpes ribs 11–13 (Clack & Finney Reference Clack and Finney2005). The anterior thoracic ribs of Acanthostega also show this morphology. Our discoveries do not include any ribs with both proximal and distal flared ends as Panchen's ‘thoracic' rib does, although this is possibly because many of the longer (presumably mid-thoracic) ribs we discovered are fractured mid-shaft.
2.1.5. Reconstruction of axial articulations
The intercentra of Crassigyrinus have small facets on the dorsolateral surface of each side. The facets are present on all intercentra that were manually prepared (centra 1 and 14–17 in Panchen (Reference Panchen1985), although on centrum 1 they are not distinct). We also found a facet on centrum 13, but only on one side, because the dorsal apex on the other side is sheared. Panchen interpreted these facets as neural arch facets, and noted that they were positioned on the anterior or posterior sides of the apex (Panchen Reference Panchen and Panchen1980, Reference Panchen1985).
However, the intercentra (except for the degree of midline fusion) and facets are similar to those of Ichthyostega, in which the facets face posterolaterally and are areas of articulation with the ribs rather than with the neural arch (Jarvik Reference Jarvik1996). Posteriorly facing rib facets are also present on the intercentra of Eusthenopteron, Greererpeton, Whatcheeria, Pederpes and Ichthyostega (Andrews & Westoll Reference Andrews and Westoll1970; Godfrey Reference Godfrey1989; Lombard & Bolt Reference Lombard and Bolt1995, fig. 3; Clack & Finney Reference Clack and Finney2005; Pierce et al. Reference Pierce, Ahlberg, Hutchinson, Molnar, Sanchez, Tafforeau and Clack2013). All of these animals had a rhachitomous (or reverse rhachitomous) vertebral pattern, so it appears that in rhachitomous vertebrae, a posterior facet for rib articulation is usually found near the apex of the horseshoe-shaped intercentrum. Therefore, we conclude that the articular facets on the Crassigyrinus intercentra also face posterolaterally and articulate with the ribs rather than the neural arch.
We can use this facet orientation and the structure of the neural arch, specifically the structure of the transverse process, to examine how the ribs may have articulated with the vertebrae in Crassigyrinus. Although the rib shape of Ichthyostega differs from Crassigyrinus in that Ichthyostega has very wide, overlapping thoracic ribs (Jarvik Reference Jarvik1996), Ichthyostega provides the best analogue for constructing the rib-head articulation with the intercentrum and neural arch, because, in the posterior thoracic vertebrae, the transverse processes of the neural arches of Ichthyostega project laterally (Pierce et al. Reference Pierce, Ahlberg, Hutchinson, Molnar, Sanchez, Tafforeau and Clack2013, fig. 1c). This resembles the transverse processes in Crassigyrinus and is unlike the condition in most other early tetrapods (see Section 2.1.3). The intercentrum shape and rib facet position is also similar in Crassigyrinus and Ichthyostega. Therefore, we hypothesise a similar rib articulation in Crassigyrinus as is present in the posterior thoracic vertebrae in Ichthyostega (Pierce et al. Reference Pierce, Ahlberg, Hutchinson, Molnar, Sanchez, Tafforeau and Clack2013, fig. 1c).
For our reconstruction, we chose to use centrum 13 and a neural arch and rib (Fig. 7g) that we discovered in close proximity to this centrum, to account for variation in size along the vertebral column. We mended the breaks in this rib to reconstruct the morphology. Figure 8 shows four possible arrangements of the costal–vertebral articulation. The rib articulates with the transverse process of the neural arch and the articular facet on the posterolateral surface of the centrum. Because the elements were found disarticulated and we did not know for certain whether the rib was a left or right rib, we tested several different orientations of the rib, as well as its antimere. Reconstructions b and c (Fig. 8) have the crest of the rib facing posteriorly; a ridge on the posterior surface of the proximal rib has been described for Eoherpeton and Proterogyrinus (Holmes Reference Holmes1984; Smithson Reference Smithson1985). No early tetrapods show the ribs curving anteriorly, so reconstruction b (Fig. 8) is unlikely. Based on these criteria, reconstruction c (Fig. 8) is the most likely. However, it is worth noting that in Ossinodus, the ridge was described to be on the anterior side of the rib (Warren & Turner Reference Warren and Turner2004), although we are not sure how posterior and anterior surfaces were identified for this disarticulated rib. Reconstructions a and d (Fig. 8) were, therefore, included to account for all possible arrangements. Overall, it appears that the ribs of Crassigyrinus projected more laterally than ventrally, and that Crassigyrinus was, therefore, a fairly flat-bodied animal.
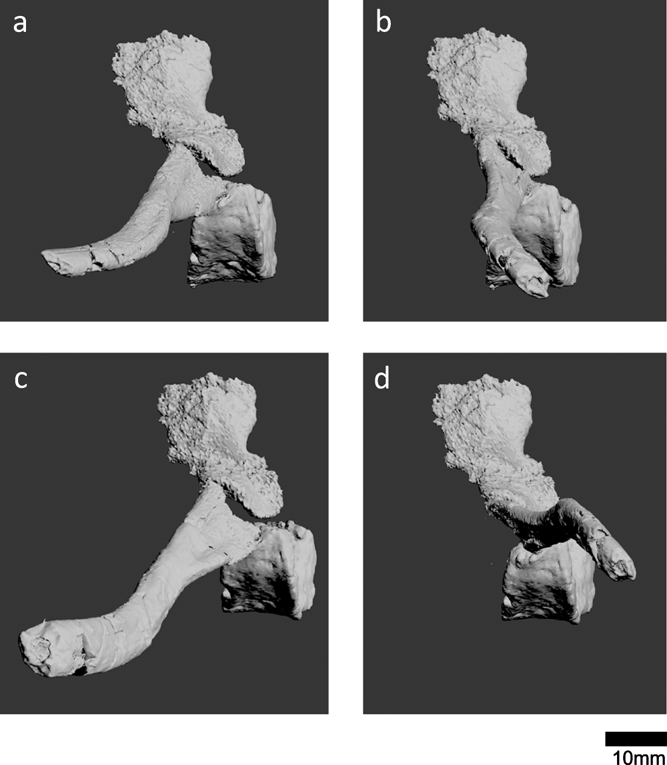
Figure 8 Potential reconstructions of the costal–vertebral articulations of Crassigyrinus, based on centrum 13 and a new neural arch and rib found near centrum 13. (a, b) Reconstruction assuming the rib is a right rib. (c, d) Reconstruction assuming the rib is a left rib; rib has been mirrored to reconstruct the right-side articulation.
2.2. Appendicular skeleton
2.2.1. Left radius
We also segmented the left radius of the NHMUK VP R10000 specimen. Previously, only the morphology of the proximal articular surface and what was described as the extensor/dorsal surface (Fig. 9a) were known (Panchen Reference Panchen1985, fig. 21). This latter surface is slightly concave, whereas the previously hidden surface (Fig. 9c), which would be the flexor/ventral surface according to Panchen's identification, is convex. The convex surface projects more distally than the exposed surface. In Archeria, Proterogyrinus, Acanthostega, Ichthyostega, Ossinodus and Pederpes, the extensor/dorsal surface is convex and projects more distally than the flexor/ventral surface (Romer Reference Romer1957; Holmes Reference Holmes1984; Coates Reference Coates1996; Jarvik Reference Jarvik1996; Warren & Ptasznik Reference Warren and Turner2002; Clack & Finney Reference Clack and Finney2005). Therefore, we reinterpret the exposed, concave surface of the Crassigyrinus radius (Fig. 9a) as being the flexor/ventral surface, and identify the previously hidden, convex, distally expanding surface (Fig. 9c) as the extensor/dorsal surface. The radius was associated with the left humerus, and we agree with Panchen's identification of the radius as a left radius. The lateral section of the distal end was in close association with an unknown bone, possibly a branchial element, discussed in Panchen (Reference Panchen1985, fig. 21).

Figure 9 Crassigyrinus left radius (a–f) and metacarpal (g), both found near the skull of NHMUK VP R10000 (Panchen Reference Panchen1985, fig. 21). Left radius in (a) flexor/ventral view; (b) lateral view; (c) extensor/dorsal view; (d) medial view; (e) proximal view and (f) distal view; (g) metacarpal in extensor view.
Panchen (Reference Panchen1985) described a lateral ridge, which probably divided flexor and extensor musculature, and noted that it was similar but not as pronounced as that found in Archeria (Romer Reference Romer1957). With our reinterpretation of dorsal and ventral sides, this ridge (Fig. 9d) is actually on the medial side of the bone, and we interpret it to be homologous to the ventromesial ridge (sensu Warren & Ptasznik Reference Warren and Turner2002) found in Proterogyrinus, Acanthostega, Baphetes and Ossinodus (Holmes Reference Holmes1984; Coates Reference Coates1996; Milner & Lindsay Reference Milner and Lindsay1998; Warren & Ptasznik Reference Warren and Turner2002). This ridge is probably also homologous to the medial ridge in Archeria (Romer Reference Romer1957). Bishop (Reference Bishop2014) suggested that the ventromesial ridge is the attachment site for the brachialis inferior. On the Crassigyrinus radius, there is a small protrusion near the proximal end of the ventromesial ridge (Fig. 9d) – a similar rugosity was interpreted as the insertion of the humeroradialis and possibly the biceps (if present) in Archeria (Romer Reference Romer1957).
Our segmentation also revealed a sharp ridge on the ventrolateral side of the bone (Fig. 9b), which we interpret to be homologous to the ventral radial crest (sensu Coates Reference Coates1996), and which has been described in several early tetrapods such as Proterogyrinus, Acanthostega, Baphetes and Ossinodus (Holmes Reference Holmes1984; Coates Reference Coates1996; Milner & Lindsay Reference Milner and Lindsay1998; Warren & Ptasznik Reference Warren and Ptasznik2002). This ridge is probably also homologous to the lateral ridge in Archeria (Romer Reference Romer1957), which is keel-shaped like the ventral radial crest in Crassigyrinus. In Ossinodus, this crest faces ventrally, whereas in Crassigyrinus it faces more laterally. The ventral radial crest of Ossinodus shows no muscle scarring, and might, therefore, not be an area of muscle attachment (Bishop Reference Bishop2014). Crassigyrinus lacks the other two ridges – the dorsomesial ridge and the proximoventral ridge (sensu Warren & Ptasznik Reference Warren and Ptasznik2002) – that are found in the radii in Ossinodus, Pederpes, Acanthostega, Greererpeton and Baphetes (Molnar et al. Reference Molnar, Diogo, Hutchinson and Pierce2017).
Overall, the morphology of the radius is very similar to that of Ossinodus (Warren & Ptasznik Reference Warren and Ptasznik2002). At the distal end, the lateral surface is more concave than the medial surface, and the dorsomedial surface extends more distally than the ventrolateral surface (Warren & Ptasznik Reference Warren and Ptasznik2002). In both Crassigyrinus and Ossinodus, the distal surface appears to be abraded (Warren & Turner Reference Warren and Ptasznik2004), and it is likely that both radii were finished in cartilage.
2.2.2. Metacarpal
We segmented a metacarpal found near the radius and humerus of the NHMUK VP R10000 specimen (Fig. 9g). This element was previously figured in outline (Panchen Reference Panchen1985, fig. 21), but here we show greater detail. The metacarpal is ∼9mm long – about half the length of the metatarsals. This is to be expected, because the forelimb is smaller than the hindlimb. There is a break in the metacarpal, and the flexor surface was compressed with the bone beneath, so we were only able to segment the extensor surface. It appears that the metacarpal, like the metatarsals (see Section 2.2.5), was asymmetrical, although taphonomic factors might be responsible for part of this asymmetry.
2.2.3. Potential ossified pubis
In the specimen NMS G 1975.5.5, the ventral/distal end of the right ilium is compacted with an irregular mass of bone (Fig. 10). The irregular mass might be an ossified pubis. One surface of the bone (Fig. 10b) has a layer of dense, laminar bone (see cross section in Fig. 10e), while the other surface is more irregular and less dense (Fig. 10′, d). The ‘histology' of the laminar layer resembles the shaft of the ilium, whereas the looser layer looks similar to the ischium's bone structure. The NMS G 1984.15.1 ilium does not have this mass of bone on its ventral/distal side.
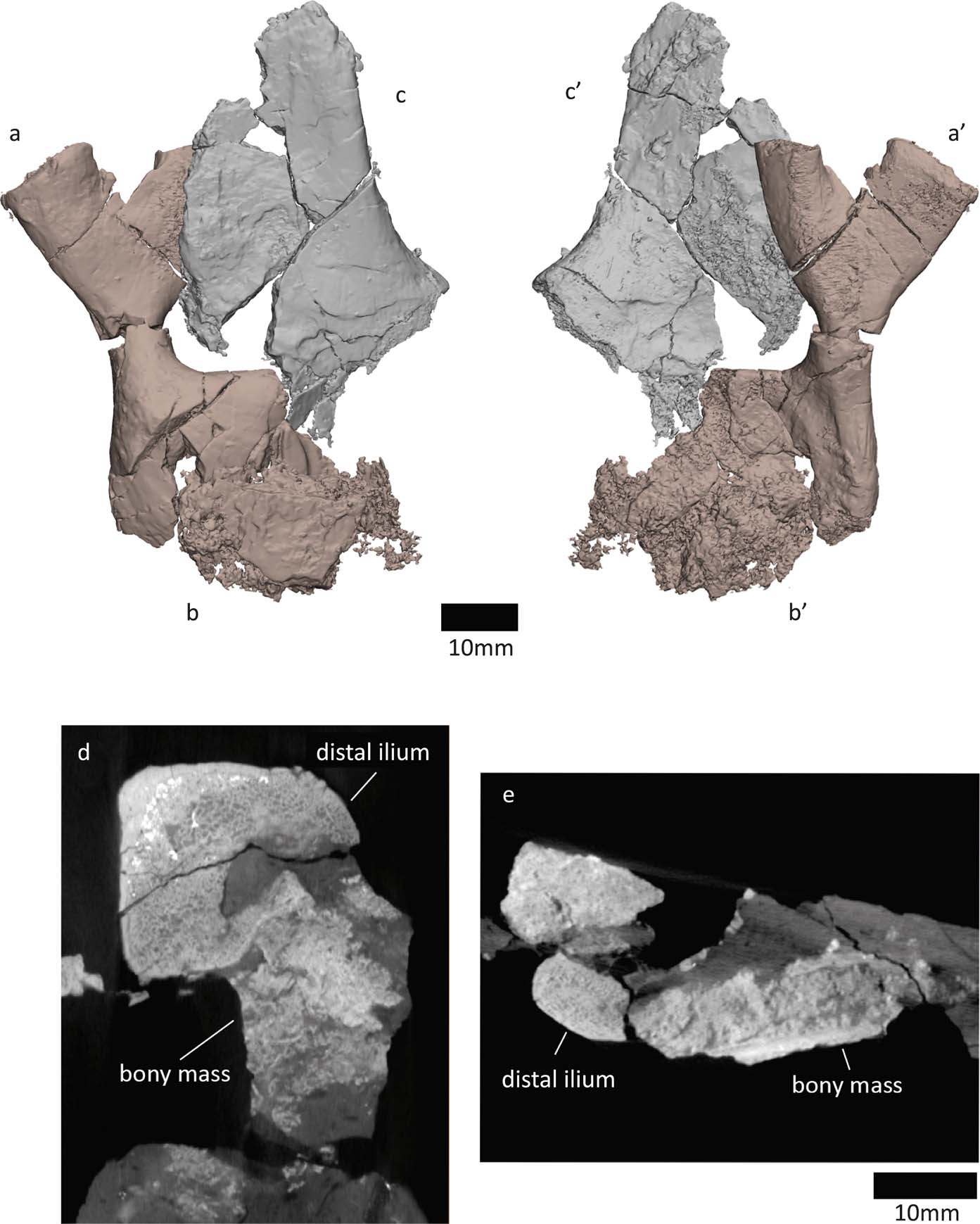
Figure 10 NMS G 1975.5.5 specimen, right ilium in (a) medial and (a′) lateral views. (b, b′) Mass of bone, potentially an ossified pubis. Left ischium in (c) lateral and (c′) medial views. (d) Axial section along distal ilium and bony mass. (e) Transverse section along distal ilium and bony mass.
If this mass of bone is indeed an ossified pubis, it is difficult to determine which side it is from. While it is associated with the right ilium, the finished surface of the pubis, which might even bear some ornamentation (see Fig. 10b), faces the same side as the medial surface of the right ilium. Ornament on the medial side would be surprising, based on the lateral location of ornament on the NMS G 1985.15.3 ischium (the ornamentation is less apparent in the 1975.5.5 ischium). If we assume the ornamentation of the pubis to be on the lateral side, this would suggest that the ossified pubis is from the left side and was compacted with the right ilium during taphonomic processes. Taphonomic distortion could also be responsible for the unusual appearance of the bone's structure.
Several Carboniferous tetrapods did not ossify their pubis (Clack Reference Clack2012). However, the ossified pubis of Greererpeton and of NSM005GF045.001 (Nova Scotia Museum, Halifax) from Romer's Gap of Blue Beach (Godfrey Reference Godfrey1989; Anderson et al. Reference Anderson, Smithson, Mansky, Meyer and Clack2015) look very similar to the element we discovered in Crassigyrinus.
2.2.4. Left fibula
Examination of the ‘left sacral rib' (Panchen & Smithson Reference Panchen and Smithson1990) in NMS G 1984.15.1 revealed that this bone is actually a left fibula. Indeed, in their description, Panchen & Smithson (Reference Panchen and Smithson1990) noted that this element resembles an epipodial. It shares several key osteological features with the right fibula in NMS G 1984.15.3 (Panchen & Smithson Reference Panchen and Smithson1990, fig. 11). Both bones have a flexor ridge, an anterior ridge, concave anterior and posterior surfaces and a triangular fossa on the posterodistal medial/flexor surface. The degree of torsion between proximal and distal surfaces is similar (Fig. 11e, e′, f, f′). The anterior proximodistal length (measured between the two most anterior points) measures 22mm in both elements. The main difference between the two bones is that the posterior proximodistal length is greater in the right fibula (29mm versus 23mm, measured between the two most posterior points). This might be due to variation in the ossification of the ends of the bone, which are unfinished and presumably were covered in cartilage. Variation between bones can be due to variation between individuals (age, sex, idiosyncratic), and can occur between left and right sides of a single individual. The slabs containing the two elements were found in close proximity, and there are no duplicate elements between the two slabs, so we infer that the two fibulae are from the same individual. The variation between the two bones may also be due to taphonomic distortion.

Figure 11 Right fibula at top, left fibula (previously identified as left sacral rib by Panchen & Smithson (Reference Panchen and Smithson1990)) at bottom. (a, a′) Lateral (extensor) view. (b, b′) Medial (flexor) view. (c, c′) Posterior view. (d, d′) Anterior view. (e, e′) Proximal view. (f, f′) Distal view. Abbreviations: l=lateral; m=medial; a=anterior; p=posterior.
Reassessment of this element means that no sacral rib has been found for Crassigyrinus. Therefore, we do not have direct evidence for the inference that ‘Crassigyrinus had a fully-formed sacrum to transmit the thrust in swimming from the hind limbs to the trunk' (Panchen & Smithson Reference Panchen and Smithson1990, p. 40). A sacral rib is absent in Tiktaalik but present in the Devonian tetrapod Acanthostega, and sacral ribs have been found in numerous Carboniferous tetrapods (Clack Reference Clack2012). It is likely that Crassigyrinus had a sacral rib (based on phylogenetic inference from its closest relatives among stem tetrapods), but the morphology and articulation with the pelvis is difficult to infer, because the precise phylogenetic position of Crassigyrinus is unclear.
2.2.5. Metatarsals and other pes elements
Panchen & Smithson (Reference Panchen and Smithson1990) tentatively identified three metatarsals (of about 15mm in length) and four phalanges (of 5–12mm in length) in specimens NMS G 1984.15.2 and NMS G 1984.15.3. We segmented these (Fig. 12 a1–5, b1, b3), because only a general shape and size have previously been described (Panchen & Smithson Reference Panchen and Smithson1990). Three of the ‘phalanges' (Figs 12a1, a2, b1) could actually be metatarsals: in several early tetrapods, for example Proterogyrinus, Greererpeton, Silvanerpeton and Pederpes, the metatarsal of the shortest digit is similar in size and shape to the proximal phalanges of the larger digits (Holmes Reference Holmes1984; Godfrey Reference Godfrey1989; Clack Reference Clack1994, Reference Clack2002). The fourth potential phalanx (Fig. 12a4) is associated with the distal end of a metatarsal (Fig. 12a5), so it could be the corresponding proximal phalanx. However, it is sheared off at one end, so it could also be another metatarsal.
We identified two additional metatarsals (Figs 12b2, c1, 13a, e) previously hidden in the matrix of the NMS G 1984.15.1 and 1984.15.3 slabs. The greatest proximodistal lengths of these new elements (∼17 and ∼14mm, respectively) are similar to the metatarsal length (15mm) reported by Panchen & Smithson (Reference Panchen and Smithson1990). The shape also agrees with the description in Panchen & Smithson (Reference Panchen and Smithson1990), as follows: the medial and lateral sides of the metatarsal are concave, and the proximal and distal ends are mediolaterally expanded. In the new metatarsals, one end (presumably distal) is slightly less broad than the other (presumably proximal) end. In the NMS G 1984.15.1 metatarsal, the proximal end is ∼10mm wide (measured as the greatest distance along the mediolateral axis), and the distal end is ∼12mm wide. In the NMS G 1984.15.3, the proximal end is ∼9mm wide and the distal end is ∼8mm wide. The flexor surface of the metatarsals is slightly concave, and the extensor surface is convex. The metatarsals are longer than they are wide, like those of Pederpes, but unlike those of Whatcheeria (Clack & Finney Reference Clack and Finney2005). At the proximal end, the flexor surface extends more proximally than the extensor surface (by about 1.5mm.

Figure 12 Crassigyrinus pes elements. (a, b) NMS G 1984.15.3 (a3, a5, b2, b3: metacarpals; a1, a2, a4, b1: small metatarsals or phalanges). (c) NMS G 1984.15.1 (c1: metatarsal; c2: metatarsal fragment or neural arch fragment). (c′) Long-axis cross section view. (c′1, c″1: metatarsal (shown in c1)). (c″) Transverse cross-section view (c′2, c″2: fragment that may be a metatarsal or neural arch (shown in c2)). Note, b2, c1 and c2 are new discoveries.
The most noteworthy feature of the metatarsals is the presence of bilateral asymmetry (Fig. 13). This asymmetry is also evident in the Carboniferous tetrapods Pederpes, Greererpeton, Silvanerpeton, Proterogyrinus and Ossinodus (Clack Reference Clack2002; Warren & Turner Reference Warren and Turner2004; Clack & Finney Reference Clack and Finney2005). It has been proposed that this asymmetry is associated with a more derived pedal orientation better suited for terrestrial locomotion than the lateral foot orientation of earlier animals such as Acanthostega and Ichthyostega, which have symmetric metatarsals (Clack Reference Clack2002; Clack & Finney Reference Clack and Finney2005). Therefore, it is unusual that the aquatic Crassigyrinus has asymmetric metatarsals. However, if asymmetry is indeed associated with terrestriality, it could support the hypothesis that Crassigyrinus is secondarily aquatic. Further comparisons of metatarsal asymmetry in aquatic and terrestrial tetrapods are needed to clarify this, but there is cause for caution in assuming that metapodial asymmetry indicates (increased) terrestriality.

Figure 13 Crassigyrinus metatarsals. (a) NMS G 1984.15.1 (new discovery). (b) NMS G 1984.15.2. (c–f) NMS G 1984.15.3 (e is a new discovery). Note that the extreme asymmetry at the distal end of the metatarsal in the third row is due to shearing of the bone, and is a taphonomic artefact. However, note the bilateral symmetry evident in the metatarsals. Abbreviations: med. = medial; lat. = lateral; prox. = proximal; dist. = distal.
We also found another fragment next to the newly recognised metatarsal in NMS G 1984.15.3 (Fig. 12c2). This piece is broken, but in cross section, the trabecular and cortical bone is visible (Fig. 12c′2, c″2). The cross section looks similar to the metatarsal's (Fig. 12c′1, c″1), but this piece might also be a neural arch, because a possible transverse process and prezygapophyses are present (see section 2.1.3).
3. Conclusion
CT scanning of the Crassigyrinus specimens enabled us to discover several previously unrecognised elements. The presence of pleurocentra is possible, which would make Crassigyrinus rhachitomous. Further research and scanning are needed to test the hypotheses that the small elements are pleurocentra. Crassigyrinus's ribs, which seem to be complete in some cases, end in a tapered shaft. The rib and vertebral structures suggest that Crassigyrinus had a relatively flat body, although there might have been some variation in the rib orientation along the vertebral column. The metatarsals show asymmetry, which might support the hypothesis of Crassigyrinus being secondarily aquatic, but more comparative work is needed to test whether there is a functional relationship between metatarsal asymmetry and locomotor mode. A mass of bone at the distal ilium may be evidence of an ossified pubis, and we show further details of the morphology of the radius. These new discoveries give us a better understanding of the anatomy of this aberrant animal (Fig. 14) and the morphological variation present in early tetrapods.
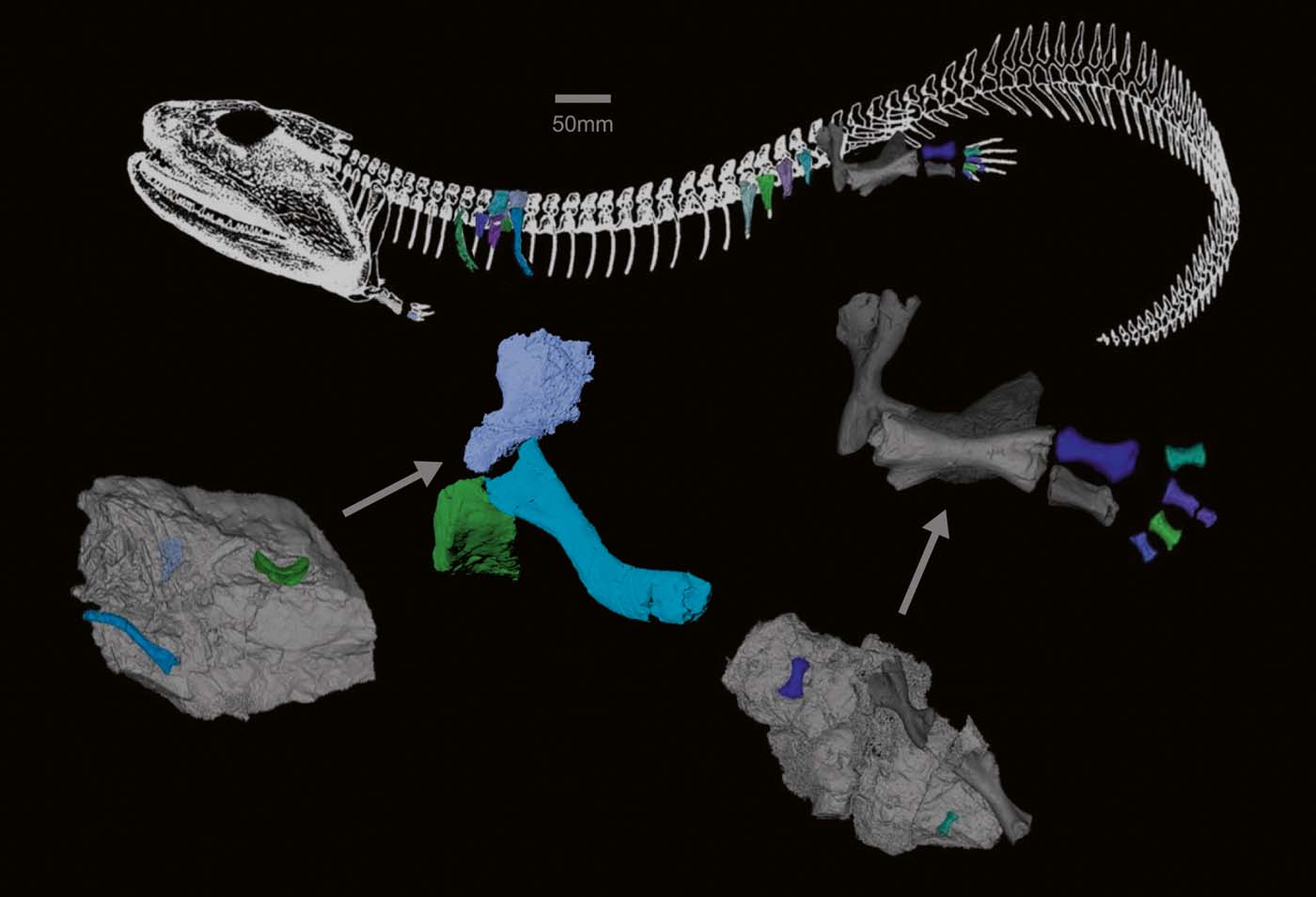
Figure 14 Models of newly discovered or described bones, mapped onto Panchen's (Reference Panchen1985) reconstruction of Crassigyrinus. Locations of vertebral elements and ribs based on comparative material.
4. Acknowledgements
We thank the two peer reviewers and editor for their very helpful feedback on the previous draft of the manuscript. We thank Jenny Clack, Tim Smithson, Stephanie Pierce and Per Ahlberg for discussions of the Crassigyrinus material; Peter Bishop for valuable discussions about the radius; Sandra Chapman for access to the Natural History Museum, London collections; Stig Walsh of the National Museums of Scotland and Tim Smithson, Jenny Clack and Mathew Lowe of the UMZC for access to the NMS G specimens; and Keturah Smithson of the UMZC for scanning these specimens. JRH was funded by the Natural Environment Research Council grant number NE/K004751/1, and ECH was funded by a Royal Veterinary College, University of London, PhD studentship.