Breast milk is considered a complete food for the newborn and breastfeeding is known to provide a wide range of health benefits to both mother and the baby. The WHO recommends exclusive breastfeeding for the first 6 months of an infant’s life, and continued breastfeeding with complementary foods for up to 2 years of age(1). However, when a mother’s milk is not available, infant milk formula is considered as an effective alternative. Cows’ milk-based infant formulas are widely used, but goats’ milk-based products are becoming increasingly popular as goats’ milk is considered to be more similar to human milk compared with cows’ milk. This includes higher levels of oligosaccharides in goats’ milk relative to milk of other mammalian species(Reference Urashima and Taufik2) and lower levels of α-S1 casein(Reference Sanz Ceballos, Morales and de la Torre Adarve3). Goats’ milk is also reported to exhibit significant homology in lactoferrin N-glycans with human milk(Reference Le Parc, Dallas and Duaut4).
Human milk is known to contain high concentrations (5–20 g/l in mature milk) and diversity of oligosaccharides(Reference Gabrielli, Zampini and Galeazzi5,Reference Ruhaak and Lebrilla6) . Several studies have shown that oligosaccharides, particularly human milk oligosaccharides (HMO), are effective in selectively promoting the growth of bifidobacteria and shaping the composition of intestinal microbiota, preventing adhesion of pathogens to intestinal mucosa and reducing the risk of bacterial, viral and parasitic infections, modulating immune and intestinal immune cell function, lowering the risk of necrotising enterocolitis and providing nutrients (sialic acid) for brain development and cognition(Reference Asakuma, Hatakeyama and Urashima7–Reference Sela and Mills12). Especially, 2’-fucosyllactose (2’-FL) has been reported to be important in protecting infants against gut infections(Reference Bode and Jantscher-Krenn13,Reference Newburg, Ruiz-Palacios and Morrow14) . This has stimulated efforts by infant formula manufacturers to produce infant formulas that closely resemble human milk in composition and performance(15). However, this process is challenging given that oligosaccharides in goats’ or cows’ milk exhibit limited diversity and are present at significantly lower concentrations compared with those in human milk(Reference Jandal16–Reference Martinez-Ferez, Rudloff and Guadix20). Importantly, the complexity of HMO makes it almost impossible for these to be duplicated in infant formulas(Reference Moro, Stahl and Fanaro21). To overcome this challenge, modern infant formulas are commonly fortified with fructo-oligosaccharides and galacto-oligosaccharides (GOS)(Reference Oozeer, van Limpt and Ludwig22). However, their effectiveness in conferring health benefits similar to those of breast milk remains to be proven(Reference Holma, Juvonen and Asmawi23,Reference Moreau, Martin and Toquet24) .
It has previously been shown that high heat treatment could cause degradation or change to the structure of some oligosaccharides(Reference Böhm, Kaiser and Trebstein25,Reference Matusek, Merész and Khanh Diem Le26) . How the heat treatments applied during manufacture of infant formula (milk pasteurisation and exposure to temperatures of 180–220°C during spray drying(Reference Skanderby, Westergaard, Patridge and Tamime27)) affect the presence and functionality of naturally present or added oligosaccharides in infant formula remains unknown. Only a handful of studies to date have attempted to quantify the amount and types of milk oligosaccharides present in infant formula products. The primary objective of the present study was to investigate the diversity and concentration of oligosaccharides present in goats’ milk and goats’ milk-based infant formula and their prebiotic and anti-pathogen adhesion properties.
Materials and methods
Oligosaccharide standards
Oligosaccharide standards were purchased from Dextra Laboratories. These included: 3’-sialyllactose, 6’-sialyllactose, 3’-sialyl-N-acetyllactosamine, 6’-sialyl-N-acetyllactosamine, 2’-FL, 3’-fucosyllactose, lacto-N-hexaose, 3’-galactosyllactose, 4’-galactosyllactose, 6’-galactosyllactose and disialyllactose. Oligosaccharide standards which were received in powder form were reconstituted with Milli-Q water (22 ± 2 °C) to make a stock solution at a concentration of 1 g/l. Stock solutions were further diluted with Milli-Q water to give a final concentration range of 0·1–0·001 g/l.
Preparation of oligosaccharides
Oli6 stage 1 (S1-GIF) and stage 2 goats’ milk infant formulas (S2-GIF) and raw goats’ milk (pooled milk from a group of ten Saanen goats) were obtained from Nuchev Pty Ltd. The GOS mixture was purchased from New Francisco Biotechnology Corporation Ltd (King-Prebiotics® GOS-700-P, batch number 17003). GIF was reconstituted according to manufacturer’s instructions. GOS was reconstituted to 20 g/l, whilst concentrations of milk oligosaccharides extracted from infant formula were adjusted to match typical levels in reconstituted GIF. Milk samples were centrifuged at 4000 g for 30 min at 4°C to remove remaining milk lipids. Equal volumes of MilliQ water were added to defatted milk and subsequently filtered through a 10 kDa molecular weight cut-off filter (Amicon® Centrifugal Filters) in a centrifuge (4000 g for 30 min). The clear filtrate was assumed to contain all oligosaccharides. Extracts were filtered with Millipore 0·45 µm syringe filter.
Oligosaccharide quantification by LC/MS
Analysis of oligosaccharides was performed based on the protocols of Liu et al. (Reference Liu, Moate and Cocks28); this method was found to be highly accurate and sensitive in detecting oligosaccharides (limit of detection of <0·1 ng and accuracy of 95–105 % for a variety of spiked oligosaccharides).
Chromatographic separation of milk oligosaccharides was achieved via a Kinetex hydrophilic Interaction liquid chromatography column (150 × 4·6 mm, 2·8 µm, Phenomenex) on an Agilent 1290 Infinity HPLC system (Agilent). Components of the LC/MS included a degasser, binary pump, temperature controlled auto-sampler (maintained at 4°C) and column compartment (maintained at 30°C). The mobile phase (A+B) consisted of water containing 5 mM ammonium acetate (A) and acetonitrile with 0·1 % formic acid (B). The flow rate was 0·6 ml/min with a gradient elution of 3–50 % of solution (A) over 35 min. Analyte detection was performed via mass spectrometry LTQ Orbitrap Velos (Thermo Scientific) with a heated electrospray ionisation source maintained at 270°C by a heating source of 350°C. The sheath, auxiliary and sweep gases were at 40, 15 and 8 units, respectively. Voltage source was fixed at 3·2 kV in negative mode. Data were collected in profile data acquisition mode over the mass range from 200 to 2000 mass/charge (m/z) in negative Fourier transform mode (resolution of 60 000) and processed using the Xcalibur software package (Thermo Scientific).
Evaluation of prebiotic properties
Two Bifidobacterium strains (B. animalis subsp. lactis BB12 and B. longum BB536) and two Lactobacillus strains (L. casei 2607 and L. acidophilus 4461) were used in these experiments(Reference Otieno and Shah29–Reference Pham and Shah31). Strains were grown anaerobically in De Man, Rogosa and Sharpe (MRS) broth(Reference De Man, Rogosa and Sharpe32) with filter-sterilised cysteine (0·05 %, final concentration) added for the Bifidobacterium strains. For growth experiments, modified MRS was used in which glucose was omitted unless otherwise indicated. Lactose (5 g/l) was also included as a positive control. Bacterial suspensions of log-phase cells in 2× modified MRS were adjusted to an optical density at 600 nm (OD600nm) of 0·11, and then 100 μl of the cell suspension was added to wells in a sterile ninety-six-well plate. Each well was pre-filled with an equal volume of milk oligosaccharide extract and gently mixed after addition of cells. The final oligosaccharide concentration was adjusted to match that of the reconstituted goats’ milk-based infant formula (ready-to-use as per the manufacturer’s instructions) in order to maintain the same concentration and relative proportions of the oligosaccharides. Plates were incubated anaerobically at 37°C for 24 h. OD600nm readings of the suspensions were taken before and after growth using a plate reader. The extent of growth was determined by the increase in OD600nm from t = 0. Each strain/substrate combination and controls in wells were assayed in triplicate. Sterile GOS (20 g/l) was included as the positive control, while negative controls contained only bacteria and the medium without added oligosaccharides. Dextrose and lactose were used as comparison benchmarks for simple sugar utilisation by the bacteria.
Cell culture
Caco-2 cells, human colorectal epithelial adenocarcinoma cells which exhibit high cellular differentiation, were selected as a human gut adhesion model. The Caco-2 cells were cultured in Dulbecco’s modified Eagle’s medium supplemented with 10 % fetal bovine serum and 1 % of an antibiotic-antimycotic solution (10 000 units/ml of penicillin, 10 000 μg/ml of streptomycin and 25 µg/ml of Gibco® Amphotericin B; Life Technologies). Cells were incubated at 37°C, 95 % humidity in an atmosphere containing 5 % carbon dioxide. At 80 % confluence, cells were passaged as follows: after aspiration of culture media, cells were washed with 5 ml pre-warmed PBS at pH 7 (Life Technologies), 5ml of trypsin-EDTA (0·25 %, Life Technologies) was added and then the cells incubated for 5–10 min. Cells were washed with fresh media (10 ml) and centrifuged at 815 g for 5 min to remove trypsin and EDTA. The cell pellet was reconstituted with 1 ml fresh media. A cell count was performed and the cells recultured in a sterile flask pre-filled with fresh media (density of 105 cells/ml). For anti-adhesion studies, twenty-four-well plates were seeded with 5 × 105 cells per well and incubated as before to obtain confluent monolayers. At 1 d prior to experimentation, the media was replaced with fresh media without any antibiotic-antimycotic.
Anti-adhesion study with Caco-2 cell monolayer
The protocol used was based on those of earlier studies with slight modifications(Reference Altamimi, Abdelhay and Rastall33,Reference Coppa, Zampini and Galeazzi34) . Escherichia coli National Collection of Type Cultures (NCTC) 10418 and Salmonella typhimurium were obtained from RMIT University’s culture collection. A single colony isolate of each strain was inoculated into 9 ml nutrient broth (Amyl Media) in a sterile tube and incubated at 37°C for 2 h with shaking for cells to reach exponential growth phase. Each suspension was then adjusted to an OD600nm of 0·3 and oligosaccharides (prepared as described previously) added at equal volumes (final oligosaccharide concentration equivalent to that of the ready-to-use reconstituted goats’ milk-based infant formula) and the cell suspensions incubated at 37°C for 120 min. In addition, sterilised GOS (20 g/l) and Milli-Q water were used as positive and negative controls, respectively. Lactose, in non-growth-limiting concentrations (5 g/l), was also included as one of the treatments to distinguish its effect from oligosaccharides. The bacteria–oligosaccharide suspension was added to each well in a sterile twenty-four-well tissue culture plate containing a Caco-2 monolayer and incubated aerobically at 37°C for 90 min. Post-incubation, the Caco-2 cells were washed three times with PBS and then lysed with cold 0·1 % Triton-X to release adherent bacterial cells. Serial dilutions of lysates were prepared and plated onto nutrient agar plates which were incubated anaerobically for 24 h or until colonies were evident. Numbers of colonies were then counted. Each experimental treatment was conducted in triplicate. Anti-adhesion percentage was calculated as:

Statistical analysis
Results for LC/MS analyses, and growth and adhesion assays are expressed as means with their standard errors. For experiments involving bacterial cultures, the difference between each treatment group was tested using one-way ANOVA and Tukey’s multiple comparison tests. Statistical significance was defined as a P value of <0·05. All statistical analyses were performed using GraphPad Prism (GraphPad Prism version 8 for Windows, GraphPad Software).
Results
LC/MS quantification of milk oligosaccharides
The high throughput analytical method used in the present study was effective in identifying oligosaccharides present in significant quantities without requiring sample concentration. A total of fourteen oligosaccharides were found to be present in S1-GIF and S2-GIF. This included seven major (predominant) and seven minor (present in small amounts) oligosaccharides (Table 1; Figs. 1 –3). 3’-galactosyllactose and 6’-galactosyllactose were grouped together as triose sugars due to their complex chromatograph profiles (Fig. 4). Of the fourteen oligosaccharides, five (2’-FL, 3’-sialyllactose, 6’-sialyllactose, lacto-N-tetraose and lacto-N-hexaose) were structurally similar to those found in human breast milk (Table 1). There was no difference in the chromatographic profiles of S1-GIF and S2-GIF (data not shown).
Table 1. Concentration of major and minor oligosaccharides detected in goats’ milk-based infant formula and their presence in human milk
(Mean values with their standard errors; relative percentages)
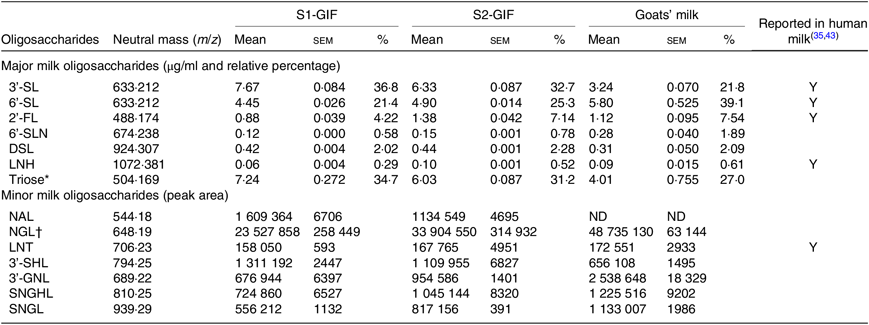
S1-GIF, stage 1 goat milk infant formula; S2-GIF, stage 2 goat milk infant formula; 3'-SL, 3'-sialyllactose; Y, yes; 6'-SL, 6'-sialyllactose; 2'-FL, 2'-fucosyllactose; 6'-SLN, 6'-sialyl-N-acetyllactosamine; DSL, disialyllactose; LNH, lacto-N-hexaose; NAL, N-acetyl-glucosaminyl-lactose; ND, not detected; NGL, glycolyl-neuraminyl-lactose; LNT, lacto-N-tetraose; 3'-SHL, sialyl-galactosyl-lactose; 3'-GNL, glycolyl-neuraminyl-lactosamine; SNGHL, N-glycolyl-neuraminyl-hexosyl-lactose; SNGL, sialyl-N-glycolyl-neuraminyl-lactose.
* Total putative concentration reported for 3’-galactosyllactose and 6’-galactosyllactose.
† Relative abundance reported as a total sum of two isomers.

Fig. 1. Ion chromatograms of extracted oligosaccharide standards and their respective retention times (shown on the X-axis). The m/z of each deprotonated ion was calculated to ±0·01. SL, sialyllactose; FL, fucosyllactose; GSL, galactosyllactose; SLN, sialyl-N-acetyllactosamine; DSL, disialyllactose; LNH, lacto-N-hexaose.

Fig. 2. Ion chromatogram of major oligosaccharides extracted from (A) goats’ milk and (B) stage 1 goats’ milk infant formula (S1-GIF).

Fig. 3. Ion chromatogram of oligosaccharides (minor oligosaccharides, without standards) extracted from (A) goats’ milk and (B) stage 1 goats’ milk infant formula (S1-GIF). NGL, glycolyl-neuraminyl-lactose; LNT, lacto-N-tetraose; SHL, sialyl-galactosyl-lactose; GNL, glycolyl-neuraminyl-lactosamine; SNGHL, N-glycolyl-neuraminyl-hexosyl-lactose; SNGL, sialyl-N-glycolyl-neuraminyl-lactose; NAL, N-acetyl-glucosaminyl-lactose.

Fig. 4. Ion chromatograms of extracted oligosaccharides: (A) galacto-oligosaccharide (GOS), (B) stage 1 goats' milk infant formula (S1-GIF), (C) goats' milk and (D) oligosaccharide standards (3'-galactosyllactose (3'-GSL) and 6'-GSL).
The oligosaccharide profile of goats’ milk was similar in diversity to that of S1-GIF and S2-GIF with a few minor differences. One of the minor milk oligosaccharides, N-acetyl-glucosaminyl-lactose (m/z 544·18) was found to be absent in raw goats’ milk (Table 1, Fig. 3), and S2-GIF had slightly higher abundance of 6’-glycolyl-neuraminyl-lactose (m/z 648·19). Furthermore, the chromatographic profile of GOS was dominated by 3’-galactosyllactose and 6’-galactosyllactose that were also detected in goats’ milk (Fig. 4). It also implies that the total concentration of triose sugars identified in the present study included GOS. For both GIF prepared according to the manufacturer’s instructions, oligosaccharide concentrations were similar to those found in normal unpasteurised goats’ milk (Table 1).
Goat milk oligosaccharides promote Bifidobacterium and Lactobacillus growth
As shown in Fig. 5, the growth rate of B. animalis BB12 and B. longum BB536 was significantly enhanced, compared with controls, when strains were co-cultured in the presence of oligosaccharides derived from S1-GIF and S2-GIF. This growth-promoting effect was also significantly greater (P < 0·5), to that observed with GOS. Oligosaccharides purified from S1-GIF and S2-GIF were also effective at enhancing the growth of L. casei 2607 when compared with controls, lactose and GOS but not dextrose. In contrast, L. acidophillus 4461 exhibited similar growth rate when cultured in the presence of GOS, lactose, as well as S1-GIF- and S2-GIF-derived oligosaccharides. These data suggest that milk oligosaccharides derived from goats’ milk-based infant formula are effective at selectively promoting the growth of health-promoting bacteria in the gut. Nonetheless, clinical trials are needed (currently being planned) to confirm these benefits in human subjects.

Fig. 5. Extent of growth of (A) Bifidobacterium animalis BB12, (B) B. longum BB536, (C) Lactobacillus casei 2607, and (D) L. acidophilius 4461 after 24 h of co-incubation with milk oligosaccharides at 37°C anaerobically. D, dextrose (+/+ indicates presence of dextrose, whilst −/− indicates absence of dextrose in bacteria culture media); L, lactose; S1-GIF, stage 1 goats’ milk infant formula; S2-GIF, stage 2 goats’ milk infant formula; GOS, galacto-oligosaccharide; OD, optical density. Values are means, with standard errors represented by vertical bars. a,b,c,d Mean values with unlike letters are significantly different (P < 0·05).
Goat milk oligosaccharides prevent Escherichia coli and Salmonella typhimurium adhesion to Caco-2 monolayer
Adhesion of E. coli NCTC 10418 and S. typhimurium was found to be inhibited by oligosaccharides present in S1-GIF and S2-GIF (Fig. 6) and no statistically significant differences were observed between S1 and S2 formulas. Purified GOS was also effective at preventing adhesion of pathogens to Caco-2 cells, to at least the same degree. Interestingly, oligosaccharides purified from S1-GIF, S2-GIF and GOS appeared to be slightly more efficient at inhibiting E. coli NCTC 10418 adhesion to Caco-2 cells compared with S. typhimurium, at least for the isolates we examined here. In our study, lactose had no significant protective effect.

Fig. 6. Bacterial counts of (A) Escherichia coli NCTC 10418 and (B) Salmonella typhimurium adhered to Caco-2 monolayers. Pathogens were co-cultured with purified oligosaccharide for 2 h aerobically at 37°C prior to being co-incubated with Caco-2 cells for 90 min at 37°C. L, lactose; S1-GIF, stage 1 goats’ milk infant formula; S2-GIF, stage 2 goats’ milk infant formula; GOS, galacto-oligosaccharide; CFU, colony-forming units. Values are means, with standard errors represented by vertical bars. a,b Mean values with unlike letters are significantly different (P < 0·05).
Discussion
The diverse array of highly complex oligosaccharides present in human breast milk (HMO) is considered to be of vital importance in promoting the growth of beneficial bacteria in the infant gut, conferring protection against intestinal infections and promoting the development and maturation of the immune system(Reference Bode9,Reference Newburg, Ruiz-Palacios and Morrow14) . Compared with this, oligosaccharides found in bovine and caprine milk exhibit lesser diversity and complexity, and are found in relatively smaller amounts. As a result, the amount and nature of natural milk oligosaccharides present in either caprine or bovine milk-based infant formulas, and their effectiveness in conferring gut and immune health benefits on the infant, similar to those of breast milk, remain to be determined and are of significant research interest.
The results of the present study show that goats’ milk-based infant formula contains significant amounts and a diverse array of oligosaccharides, many of which are structurally similar to HMO. To our knowledge, this is the first such report showing such richness of oligosaccharides in goats’ milk-based infant formula and their similarity to HMO. Fourteen oligosaccharides, including seven major oligosaccharides, were detected in goats’ milk-based infant formula. Of these, five oligosaccharides are also found in human breast milk (Table 1). Goats’ milk was also found to contain a similar number of oligosaccharides. Notably, the number of oligosaccharides detected in the present study is significantly lower than that reported previously in goats’ milk(Reference Martinez-Ferez, Rudloff and Guadix20,Reference Albrecht, Lane and Marino36–Reference Tangvoranuntakul, Gagneux and Diaz42) . This is probably due to major differences in the methods used in these studies. The present study used a high throughput method that was aimed at detecting only quantifiable oligosaccharides; the samples used in the present study involved the reconstitution of powders according to manufacturer’s instructions for infant use and involved no sample concentration steps. In contrast, all earlier studies have employed methods involving significant enrichment of oligosaccharides with the aim to detect all oligosaccharides, including those present in trace amounts. Using a similar method, we detected thirty seven oligosaccharides in goats’ milk in earlier studies (Leong et al., unpublished results). Similar profile and diversity of oligosaccharides detected in raw goats’ milk and goats’ milk-based infant formula suggests that oligosaccharides are not affected by heat treatment used during manufacture of infant formula.
As in human breast milk, fucosylated and sialylated oligosaccharides were found to be the dominant oligosaccharides present in goats’ milk-based infant formula. 2’-FL is the most abundant oligosaccharide in human milk(Reference Albrecht, Lane and Marino36,Reference Thurl, Munzert and Boehm43) and is the focus of significant commercial and regulatory interest. It has been shown to play a significant role in anti-infection properties of breast milk; for example, infants with high levels of 2’-FL have been shown to exhibit greater resistance to stable toxin of E. coli and Campylobacter-associated diarrhoea(Reference Newburg, Ruiz-Palacios and Altaye44). Oligosaccharides resist digestion in the small intestine and reach the colon intact where they are known to influence the structure and function of gut microbiota. For example, several studies have shown that HMO are effective in selectively promoting the growth of beneficial bacteria, such as B. bifidum that dominates the gut microflora of breast fed infants(Reference LoCascio, Desai and Sela45–Reference Turroni, Bottacini and Foroni48). The ability of HMO and a mixture of fructo-oligosaccharides/GOS to promote the growth of bifidobacteria and lactobacilli in vitro also has been demonstrated(Reference Shen, Tuohy and Gibson49). In the present study, goat milk oligosaccharides were found to exhibit similar prebiotic properties. They were found to be effective in promoting the growth of both Bifidobacterium and Lactobacillus species. Furthermore, oligosaccharides derived from both stage 1 and stage 2 goats’ milk-based infant formula appeared to be more efficient at promoting the growth of B. longum BB536 and L. casei 2607 than GOS. Whether this suggests a stronger prebiotic activity of goat milk oligosaccharides compared with GOS generally or is simply a reflection of bacterial species and/or strain selectivity in substrate utilisation is not clear(Reference Garrido, Ruiz-Moyano and Jimenez-Espinoza50).
Differences in the ability of bifidobacteria and lactobacilli in their ability to utilise carbohydrates such as lactose, GOS and lactulose have also been previously reported(Reference Watson, O’Connell Motherway and Schoterman51). Lactose is highly likely to be present in the GIF oligosaccharide fractions, however, the relatively insignificant growth-promoting effect of lactose, especially for bifidobacteria, compared with that for S1 and S2 fractions, suggests that the growth enhancement observed with S1 and S2 was largely due to oligosaccharides. Furthermore, the GIF was found in our study to be rich in 3’-sialyllactose, 6’-sialyllactose and 2’-FL, and the ability of B. longum and B. infantis to selectively ferment these oligosaccharides together with similarities in the gut microbiota composition of infants fed goats’ milk-based infant formula compared with breast-fed babies has also been recently reported(Reference Tannock, Lawley and Munro52).
The results of our study further showed that oligosaccharide enriched fractions prepared from both stage 1 and stage 2 goats’ milk-based infant formula were effective in reducing the adherence of E. coli and S. typhimurium to Caco-2 cells, as similarly reported for HMO(Reference Tangvoranuntakul, Gagneux and Diaz42,Reference Thongaram, Hoeflinger and Chow47) . Lower incidence of gastrointestinal infections in breast-fed infants compared with formula-fed infants(Reference Victora, Bahl and Barros53) and a role for HMO in this protection have been reported(Reference Morrow, Ruiz-Palacios and Jiang54). We found differences in the anti-adhesion efficacy against two different enteric pathogens to Caco-2 cells when pre-incubated and co-cultured with the same GIF oligosaccharide fractions, although further strains or isolates of these pathogens need to be tested before making a definitive conclusion. However, it is significant in this regard that Coppa et al. (Reference Coppa, Zampini and Galeazzi34) found species differences between E. coli, Vibrio cholerae and S. fyris in experiments using HMO and (like us) Caco-2 cells. Whilst some recent studies have reported that oligosaccharides from human, cows’ and goats’ milk(Reference Facinelli, Marini and Magi55,Reference Urakami, Saeki and Watanabe56) are effective in inhibiting the adhesion of E. coli to gut cells, these experiments were conducted using much higher concentrations of oligosaccharides than we used in our study, suggesting the possibility of a dose-dependent effect. In our study, GOS was at least equally as effective as goat milk oligosaccharides in preventing the adhesion of E. coli and S. typhimurium (by 52·4 and 35 %, respectively, compared with the negative control; see Fig. 6).
Attachment of pathogens to specific receptors on the gut epithelial cells is a critical step in host colonisation and infection. Carbohydrate chains form a major component of cell surface membranes, and lactose being a core saccharide of glycolipids represents a major binding site for bacteria(Reference Karlsson57,Reference Sharon and Ofek58) . Some pathogens also bind to lectins in attempt to colonise gut epithelial surfaces and the lectin binding requires either sialyl or fucose groups to be present on the cognate glycan binding partners(Reference Newburg, Ruiz-Palacios and Morrow14). The resemblance of certain GOS to saccharide-containing glycoproteins used by many pathogens to attach to intestinal cells has also been reported(Reference Kunz, Rudloff and Baier59). Due to structural similarities between goats’ milk oligosaccharides and gut cell surface carbohydrate groups, these compounds, especially sialylated and fucosylated oligosaccharides, may reduce pathogen adhesion to gut epithelial cells by acting as soluble analogues of host cell receptors and/or changing the expression of such structures(Reference Ebersbach, Andersen and Bergstrom60–Reference Ofek, Hasty and Sharon62). In addition, oligosaccharides have also been shown to mediate protection against intestinal pathogens by enhancing immune function(Reference Triantis, Bode and Van Neerven11). Together, these results combined with the results of our study show that oligosaccharides present in goats’ milk-based infant formula have strong prebiotic and anti-infection properties and may confer protection against gastrointestinal infections to the infant. Further studies are required to confirm if this is so.
Acknowledgements
We thank Nuchev Pty Ltd for providing goat milk infant formula (Oli6) and raw goat milk samples and Dr Osaana Donker for providing Bifidobacterium and Lactobacillus strains. We also express our thanks to Justin Peace for helpful discussions.
H. G. and B. Z. had received funding from Nuchev Pty Ltd in the past to review nutritional composition and analyse oligosaccharides in goats’ milk. The research costs were covered by RMIT University funding for PhD student (A. L.) and an Entrepreneurs’ Programme – Innovation Connections Grant between the Australian Government and Nuchev Pty Ltd (ICG number 55140). Nuchev Pty Ltd had no role in the design of the study; in the collection, analyses or interpretation of data; in the writing of the manuscript; and in the decision to publish the results.
Contributions of authors were as follows: conceptualisation: H. G., B. Z. and A. L.; data curation: A. L.; formal analysis: A. L. and Z. L.; investigation: A. L.; methodology: A. L., Z. L., C. P., H. G., B. Z. and H. A.; resources: H. G.; supervision: H. G. and B. Z.; validation: A. L.; writing original draft: A. L.; Writing–review and editing: H. G., C. P. and B. Z.
The authors received co-funding from Nuchev Pty Ltd for this research.