Introduction
Over the past three decades there has been an apparent increase in the occurrence and spread of jellyfish outbreaks (Legovic, Reference Legovic1987; Mills, Reference Mills2001; Attrill et al., Reference Attrill, Wright and Edwards2007; Brotz et al., Reference Brotz, Cheung, Kleisner, Pakhomov and Pauly2012). Whether this increase is a global trend or a phenomenon restricted to certain areas, the ecological consequences associated with the presence of large numbers of these voracious planktivores may be extensive. Predation on fish eggs and larvae (Purcell, Reference Purcell1985; Cowan & Houde, Reference Cowan and Houde1993; Paradis et al., Reference Paradis, Pepin and Brown1996; Cao et al., Reference Cao, Liu, Yu, Zhao, Shan, Zhuang and Dou2015; Tilves et al., Reference Tilves, Purcell, Fuentes, Torrents, Pascual, Raya, Gili and Sabates2016) and competition with fish for zooplankton (Purcell & Arai, Reference Purcell and Arai2001; Purcell & Sturdevant, Reference Purcell and Sturdevant2001; Brodeur et al., Reference Brodeur, Suchman, Reese, Miller and Daly2008) were shown to have both top-down and bottom-up effects which could lead to ecosystems dominated by jellyfish (Lynam et al., Reference Lynam, Gibbons, Axelsen, Sparks, Coetzee, Heywood and Brierley2006). Moreover, massive consumption of zooplankton by medusae could potentially alter phytoplankton dynamics (Stibor et al., Reference Stibor, Vadstein, Diehl, Gelzleichter, Hansen, Hantzsche, Katechakis, Lippert, Løseth, Peters, Roederer, Sandow, Sundt-Hansen and Olsen2004; Moller & Riisgard, Reference Moller and Riisgard2007) and even trigger a cascade effect on the food web, all the way down to the microbial communities (Riemann et al., Reference Riemann, Titelman and Bamstedt2006).
Since the mid-1980s, the Mediterranean coastal waters of Israel have experienced jellyfish swarms on an annual basis, dominated by the scyphomedusa Rhopilema nomadica. These swarms, of what is believed to be a Lessepsian migrant (Spanier & Galil, Reference Spanier and Galil1991), are estimated to consist of hundreds of thousands of individuals per square nautical mile and many of the medusae are large, weighing as much as 40 kg, with bells up to 80 cm in diameter (Lotan et al., Reference Lotan, Benhillel and Loya1992). In general, these swarms appear towards the end of May/beginning of June, and disappear by the end of July/beginning of August (Edelist et al., Reference Edelist, Guy-Haim, Kuplik, Zuckerman, Nemoy and Angel2020). This abundance has a negative effect on many coastal and marine operations, including local fisheries (Nakar, Reference Nakar2011; Angel et al., Reference Angel, Edelist and Freeman2016), industrial enterprises such as power and desalination plants (Rilov & Galil, Reference Rilov, Galil, Rilov and Crooks2009) and recreational activities (Ghermandi et al., Reference Ghermandi, Galil, Gowdy and Nunes2015). Despite its annual prominence in Israeli Mediterranean coastal waters and the potential impact medusae may have on food webs, studies concerning the ecology of R. nomadica are few and have focused mainly on its life cycle and expanding distribution (Lotan et al., Reference Lotan, Benhillel and Loya1992, Reference Lotan, Fine and Benhillel1994; Kideys & Gucu, Reference Kideys and Gucu1995; Yahia et al., Reference Yahia, Kefi-Daly Yahia, Gueroun, Aissi, Deidun, Fuentes and Piraino2013). Moreover, studies concerning its interactions with other members of the marine food web have not been conducted. This study is a first attempt to characterize R. nomadica's feeding patterns and to explore its role within the Israeli Mediterranean coastal food web. An assessment of R. nomadica's diet will be presented and compared with the composition of major ambient plankton taxa and considered with respect to this medusa's proliferation in a highly oligotrophic environment such as the Levant.
Materials and methods
Medusa samplings and processing
Gut contents of 41 specimens of the scyphomedusa Rhopilema nomadica (bell diameter size range: 10–57 cm) were sampled during summer 2015. Sampling surveys began shortly after medusae were first observed (end of May) and ended when they became scarce (end of July). Only individuals that appeared intact were sampled. Six sampling surveys on six different dates were executed 0.5–1 nautical mile offshore Mikhmoret, located on the central Mediterranean coastline of Israel (32°24′23″N 34°52′24″E). All samplings took place in the morning hours, 07–11:00 am, by means of a motor boat. Bottom depth at the sampling sites ranged from 12–35 m.
Medusae were captured individually and brought onboard by means of a 60 cm diameter dip net. Size (bell diameter, ±1 cm) and wet weight (±0.1 kg) of each medusa were recorded. Gut content of each medusa was collected immediately after bringing the jellyfish onboard. Sampling of the jellyfish gut to retrieve its prey was performed through the aboral surface; by dissecting and carefully removing the apex of the umbrella, thereby exposing the gastric pouches. The latter were then rinsed with 50 μm filtered seawater via squirt bottle and the retrieved content was fixed immediately in buffered formalin (4% v/v; final concentration). The fixed samples were stored in the laboratory at room temperature until processed for taxonomic classification and abundances, within 4 months.
Gut content composition was defined as the relative abundance (%) of each taxon out of total prey items retrieved from the pouches (taxa abundances and comparisons between medusae were based on these relative abundances). Extraction of food items from the gut was performed in a uniform manner for all medusae and was assumed to reliably represent the diet composition of each individual.
Plankton tows
On each of the six sampling excursions, a horizontal plankton tow was performed to characterize the local ambient plankton population and compare its taxonomic composition with that of the gut contents. Plankton was sampled using a 50 cm diameter (~0.2 m2 opening), 150 μm mesh size plankton net, towed at ~1 knot, at depths of 2–10 m. Duration of each tow was ~10 min. A calibrated General Oceanics flowmeter was used to calculate the volume of water filtered.
Samples were fixed with buffered formalin (4% v/v final concentration) and stored at room temperature until they were processed for taxonomic classification, within 4 months.
Shortly before sorting, each plankton sample was concentrated onto a 100 μm mesh, rinsed with tap water to eliminate the fixative, and was then transferred to 70% EtOH.
Both gut contents and plankton samples were sorted using a 15 ml plexiglass counting chamber (Bogorov design), super-fine sorting needles and a Motic SMZ-171 stereomicroscope. Organisms were identified and classified to the lowest possible taxonomic level. Representative taxa were measured and photographed via Moticam 2 digital camera and software. Plankton from the tows were sub-sampled, using a 5 ml Stempel pipette, and a minimum of 500 plankters per tow (~3 or more sub-samples) were sorted and counted to determine their relative abundances.
Histological analysis
Male and female gonadal tissues were sampled and fixed in 4% formaldehyde/seawater solution for 24 h, after which samples were rinsed in running tap water, dehydrated in 70% ethanol and embedded in paraffin. Histological cross sections (7 μm thick) were cut with a Shandon M1R rotary microtome and were stained with Gill's haematoxylin and eosin. The vertical distance between each cross section was more than 300 μm. Five histological slides were prepared for each sample containing several slices of the gonadal tissues. The histological sections were examined under a light microscope (Olympus BX43) fitted with a digital camera (Olympus DP72).
Data analysis
In order to test whether the number of R. nomadica analysed was sufficient for describing its diet, a cumulative prey type curve was generated by plotting the cumulative number of prey types identified in the medusan guts against the cumulative number of gut contents analysed. An adequate sample size is assumed when the curve approaches an asymptote (Hurtubia, Reference Hurtubia1973; Ferry & Cailliet, Reference Ferry and Cailliet1996). PRIMER-e v6 software was used to generate the curve (Chao1).
A Spearman's rank-order correlation was run to assess: (1) the relationship between the relative abundance of various prey types >150 μm ingested by the medusae (% prey type out of total >150 μm prey items) and their relative abundance in the plankton (% prey type in the plankton samples) and (2) the relationship between size of the medusa (bell diameter) and the relative abundance of each of its major dietary components.
After ensuring normality (Shapiro–Wilk test) and equality of variances (Levene's test), one-way ANOVA was used to determine whether there are any statistically significant differences between the number of ingested prey groups on the different sampling dates.
To test the null hypothesis that there was no significant difference in prey composition among medusae of varying bell diameter, an analysis of similarity (ANOSIM) was performed (Clarke, Reference Clarke1993). Prey groups which contributed most to the dissimilarities between diet compositions were identified through similarity percentages (SIMPER) (Clarke, Reference Clarke1993). Diet compositions were visualized by means of non-parametric multi-dimensional scaling (nMDS).
Results
Gut content composition
A total of 15,192 prey items were found in 41 gut contents and sorted into 28 different prey types. Bell diameter of the sampled medusae ranged from 10–57 cm and wet weight from 0.11–9.1 kg (Table 1). Cumulative prey type curves plotted for the gut contents indicated that the overall number of gut contents sampled was sufficient for a reliable characterization of Rhopilema nomadica's diet (Figure 1). In fact, the curve starts to plateau after 5 gut contents, which was the minimum number sampled on a given date.
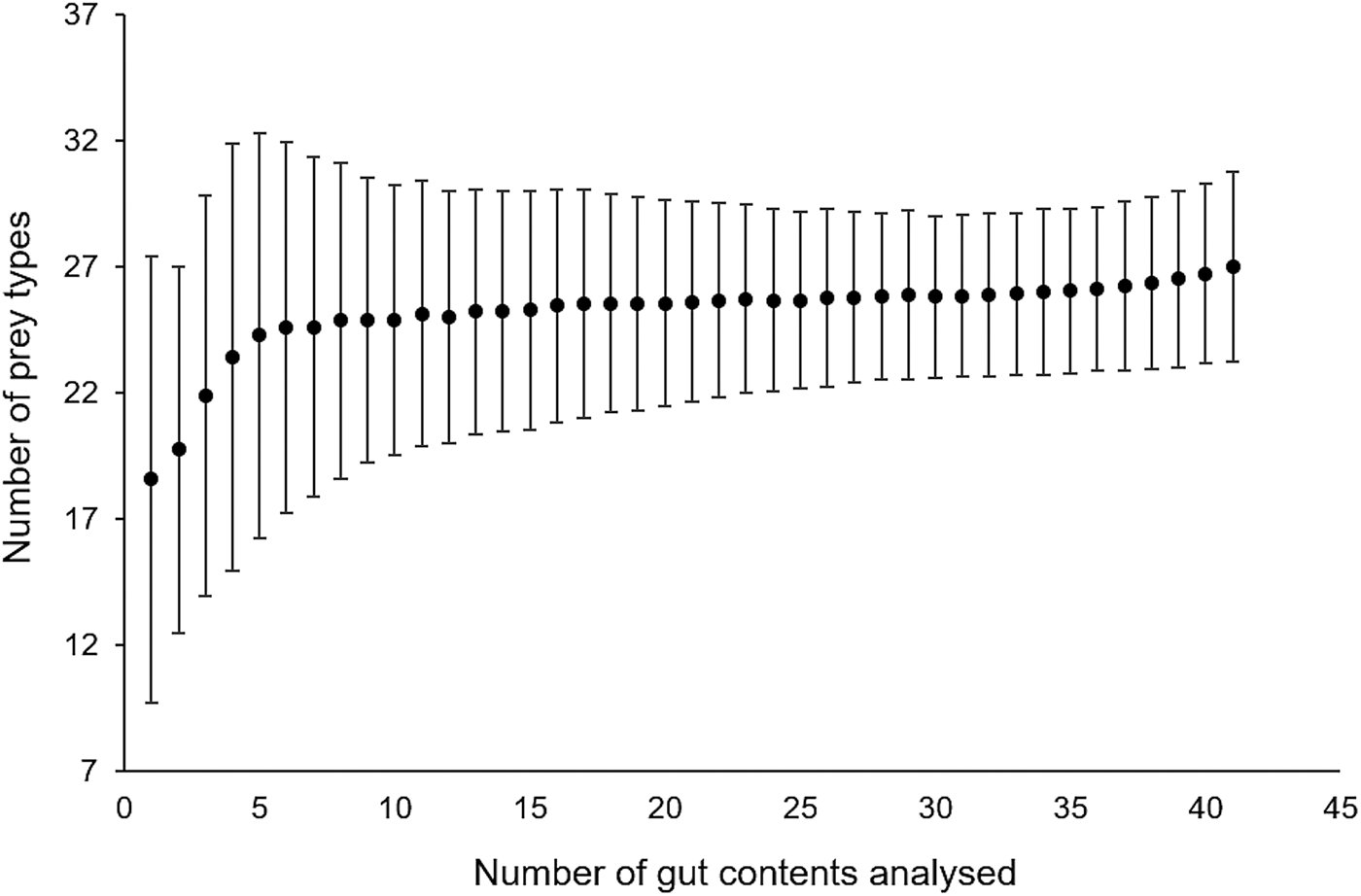
Fig. 1. Cumulative prey type curve (Chao1) to evaluate the required sampling effort (number of medusa guts analysed) to reliably estimate diet composition.
Table 1. Summary of the data collected for gut content analyses on six sampling excursions during June–July 2015

SD, standard deviation; ind., individuals.
While processing jellyfish gut contents, we found that R. nomadica feeds primarily on micro-planktonic prey <150 μm (65% of total prey ingested). In order to compare diet composition to that of plankton sampled by means of a 150 μm mesh net, prey items were sub-divided into two size groups, <150 and >150 μm, and all comparisons with plankton composition (e.g. gut content analysis, below) were performed on the latter.
On average, 12 prey types were found to comprise ≥1% of the medusa's diet (Table 2), and 10 prey types were regular dietary components found in medusae guts on all sampling dates (Supplementary Table S1).
Table 2. Relative abundance (% ± standard deviation) of the 12 prey types found in R. nomadica's gut contents which comprised ≥1% of its diet

Taxa presented in descending order of mean relative abundance of pooled contents (last column).
Of the 12 prey types, four groups contributed more than 70% of the pooled R. nomadica diet: bivalves (<150 μm), various eggs (<150 μm), calanoid and cyclopoid copepods (all >150 μm), and copepod nauplii (<150 μm), comprising 24, 18, 17 and 14% of the diet, respectively. Occasional prey, found at a mean overall relative abundance of less than 1% in the gut contents, included ascidian larvae, nematodes, hydromedusae and platyhelminthes (Supplementary Table S1).
Plankton composition
Plankton samples were sorted into 22 organism groups, of which on average 11 were found to comprise ≥1% of the plankton assemblage (Table 3). The four most abundant groups: calanoid, cyclopoid and harpacticoid copepods, diatoms and radiolarians comprised more than 80% of the plankton. Taxa found in low abundances (<1%) included various unidentified eggs, polychaetes, hydromedusae and ciliates.
Table 3. The relative abundance (% ± standard deviation) of plankton groups found within the plankton samples which comprised ≥1% of total plankton assemblage

Taxa presented in descending order of mean relative abundance of the plankton groups. <1 represents relative abundance of less than 1%. Taxa in bold represent prey found within R. nomadica's gut contents at a mean relative abundance ≥1%. Standard deviation presented for each sampling date was calculated through counting a minimum of three sub-samples of the date's sample.
Gut content analysis
The prey consumed by the medusae reflect most of the taxa found in the plankton (Supplementary Table S2), but there was no correlation between the cumulative gut content and the plankton tow yield of the major dietary items (Figure 2, Table 4). Notably radiolarians, the third most abundant taxa in the plankton, were not observed at all in the gut contents.

Fig. 2. Abundance of plankton >150 μm in ambient water and in the guts of R. nomadica. Presented are taxa which were found to comprise at least 1% of the plankton composition. Taxa appear in descending order according to their abundance in ambient water samples. Mean and standard deviation, N = 6. †Creseis sp.
Table 4. Correlation test results (Spearman's rho) between prey types >150 μm ingested by R. nomadica (comprising ≥1% of its total diet composition) and their relative abundance in plankton samples

P (two-tailed) significance <0.05.
A shift in the population, from large to smaller medusae (i.e. smaller bell diameter), was recorded during the sampling period (Figure 3A). Larger specimens dominated the population at the beginning of the summer and the relative abundance of smaller medusae increased towards the last sampling excursion. Whereas the change in medusa bell diameter had no significant impact on the number of prey groups comprising the medusa diet (Figure 3B), positive and negative correlations were found between bell diameter and the relative abundance of a few of the ‘most consumed’ taxa in the GC (Table 5). Large mesozooplankton prey, such as appendicularians and copepods, were captured and ingested more frequently by larger medusae, while smaller medusae fed more on the small prey (e.g. bivalve larvae and small diatoms). Whereas there was a decrease in mean size of the medusae, the abundance in the plankton of the four most contributing taxa to the R. nomadica diet remained relatively stable for the entire study (Figure 4).

Fig. 3. (A) the temporal shift of sampled R. nomadica's population towards smaller specimens; (B) the number of taxa found to contribute at least 1% to the diet of R. nomadica during June–July 2015. For (A), R 2 = 0.9985, y = 0.0113x2–950.13x + 2E + 07. For (B), F (1,5) = 1.34, P = 0.27, Power = 0.42 (one-way ANOVA).

Fig. 4. Time dependence of size (bell diameter) of R. nomadica and ambient concentrations of the four most contributing taxa (>150 μm) to the medusa's diet.
Table 5. Correlation test results (Spearman's rho) between size (bell diameter) of R. nomadica specimen and the relative abundance (%) of major taxa found in their gut content

P (two-tailed) significance <0.05.
It was also observed that the medusa population transitioned from one with intact (oral arms and appendages) individuals to a population that consisted of a larger number of medusae with damaged bells, reduced oral arms and even complete loss of external as well as internal feeding structures (Supplementary Figure S1). Almost all of the medusae had gonads, most of which were in advanced stages of sexual maturity (Figure 5).

Fig. 5. Histological sections of R. nomadica's gonads in advanced stages of sexual maturity. (A) female gonad; (B) male gonad. EO, Early oocyte; LO, Late oocyte; ES, Early spermatid; LS, Late spermatid.
It is noteworthy that despite the apparent physical deterioration, the medusae were swimming vigorously. Based on these observations (size and physiological state), medusae were divided for further analysis into three size groups, each group representing two sampling dates: ≥40.5 cm (N = 12; 16/06 and 17/06), 18.2–40.5 cm (N = 15; 01/07 and 05/07) and ≤18.2 cm (N = 14; 15/07 and 22/07).
A non-parametric multidimensional scaling (nMDS) and analysis of similarity (ANOSIM) of the gut content samples, where bell diameter size group is the independent factor (Figure 6, Table 6) indicates that significant dissimilarities exist between gut content compositions (P < 0.05). Pteropods, copepod nauplii and calanoid and cyclopoid copepods were found to contribute most to the observed differences.

Fig. 6. A non-parametric multidimensional scaling (nMDS) of the relative abundance of prey types in the gut content of R. nomadica, in relation to its bell diameter size group.
Table 6. ANOSIM (Analysis of Similarity) of the diet compositions in relation to the medusa's bell diameter

The most contributing taxa to the differences found via ANOSIM were identified via SIMPER (similarity percentages) analysis and are specified on the two right columns.
Discussion
The rhizostome Rhopilema nomadica has been described as one of the ‘100 worst invasive species in the Mediterranean’ (Streftaris & Zenetos, Reference Streftaris and Zenetos2006). Despite its negative impact on human activities and interests along the Israeli coast, information concerning its ecology is limited and, in this regard, the results presented here are the first to describe its diet and its possible impacts on the ecosystem.
The first notable observation that emerged from examination of R. nomadica's gut contents was the size distribution of the ingested prey. In five of the six excursions, more than 60% of the identified prey in the medusan gut were smaller than 150 μm (Table 2, Supplementary Table S1). These findings are not unusual: although scyphomedusae are known to be opportunistic predators (Larson, Reference Larson1987; Brewer, Reference Brewer1989; Ishii & Tanaka, Reference Ishii and Tanaka2001) able to consume a wide variety of zooplanktonic prey that they encounter, their feeding preferences may vary greatly. While members of the Semaeostomeae (e.g. Pelagia noctiluca, Aurelia aurita and Cyanea capillata) are able to consume prey larger than several millimetres, including large copepods and fish larvae (Purcell, Reference Purcell2003; Purcell et al., Reference Purcell, Tilves, Fuentes, Milisenda, Olariaga and Sabates2014; Cao et al., Reference Cao, Liu, Yu, Zhao, Shan, Zhuang and Dou2015), rhizostomes feed mainly on microplankton (20–200 μm), including larval zooplankton and even small taxa such as ciliates (Liu et al., Reference Liu, Wang, Li and Dong2011). This size-dependent prey selectivity is probably related to the anatomy of the feeding structures of the Rhizostomeae (Larson, Reference Larson1991; Lee et al., Reference Lee, Yoon and Lim2008). Whereas semaeostome medusae have a flexible central mouth opening which enables them to ingest even relatively large prey, rhizostome medusae lack a defined mouth. Instead, ciliated grooves located at the distal end of their branched oral arms transfer the captured prey into a set of canals towards the gastric cavities (Smith, Reference Smith1934). Stereomicroscope images show that the diameter of the openings of the ciliated grooves and the adjacent canals extending towards the gastric cavities in R. nomadica are 100–200 μm wide (Figure 7). It is likely that these ciliated openings can expand to a certain extent since larger prey were also found in the gut contents. Nevertheless, prey at the micro-size scale is probably ‘preferred’ due to the physical constraints of the feeding structures.

Fig. 7. Distal part of one of the branched oral arms of R. nomadica. Prey is captured by batteries of nematocytes (Nm) located on the club-shaped structures and transferred through the ciliated grooves (CGr) to canals leading to the gastric cavities. CGrD, ciliated groove diameter; Cl(cs), canals (cross-section).
A diet similar to the one described here for R. nomadica was also described by Liu et al. (Reference Liu, Wang, Li and Dong2011), where larvae and early developmental stages of crustaceans and molluscs comprised most of the diet of Rhopilema esculentum in South-east Asian waters. In comparison to the dominance of zooplankton in both Rhopilema spp. diets, Rhizostoma pulmo, studied at the Mar Menor coastal lagoon in Spain (Mediterranean waters), consumed mainly microphytoplankton (Perez-Ruzafa et al., Reference Perez-Ruzafa, Gilabert, Gutierrez, Fernandez, Marcos and Sabah2002). While it is only reasonable to assume that different species of rhizostomeae prefer to feed on different plankters, intraspecific differences in the diet of two populations of cannonball jellyfish, Stomolophus meleagris, were shown to occur (Larson, Reference Larson1991; Guadalupe Padilla-Serrato et al., Reference Guadalupe Padilla-Serrato, Lopez-Martinez, Acevedo-Cervantes, Alcantara-Razo and Hiram Rabago-Quiroz2013). Seeing as those two populations were found in different oceans (NE Gulf of Mexico and NW Mexico) with different plankton communities, Guadalupe Padilla-Serrato et al. (Reference Guadalupe Padilla-Serrato, Lopez-Martinez, Acevedo-Cervantes, Alcantara-Razo and Hiram Rabago-Quiroz2013) hypothesized that the observed differences in feeding may be related to the relative abundance of the available prey in the studied environments. In the absence of dietary data on other populations of R. nomadica, we are unable to speculate on the effect of prey availability on the feeding of this medusa. No correlation was found between the proportions of major prey types >150 μm ingested by the medusae and their relative abundance in the plankton (Table 4). For example, while it appeared that there was a preference for pteropod prey (they comprised 6%, on average, of the medusa gut contents in four of the six samplings), pteropods were generally found in very low abundances (<1%) in the plankton. This dietary mismatch may be real but may also be related to the limitations involved with studying a gelatinous organism's diet through its gut contents and trying to deduce its feeding preferences by comparing the contents to the plankton community in the surrounding waters. The patchy nature of plankton (Omori & Hamner, Reference Omori and Hamner1982; Hamner, Reference Hamner1988; Ritz, Reference Ritz1994) and the lack of knowledge concerning medusan digestion rates for the different prey types (Martinussen & Bamstedt, Reference Martinussen and Bamstedt2001) may bias the comparisons. Gut content composition may be different from the composition of plankton in our samples simply due to the fact that the medusa encountered prey patches different in composition than the patches we sampled. With respect to digestion times, since soft-bodied plankton (e.g. medusae, appendicularians) are digested faster than shelled ones, such as molluscs (Suchman et al., Reference Suchman, Daly, Keister, Peterson and Brodeur2008), remains of the hard-bodied organisms are likely to be found in greater proportions. As discussed in Sheppard & Harwood (Reference Sheppard and Harwood2005), biochemical and/or molecular techniques are probably superior to visual inspection when attempting to identify prey remains in gut contents, but these were not employed in this study.
What is more puzzling, however, is the apparent selectivity of prey ingested. Radiolarians were one of the dominant taxa in the plankton, yet they were entirely absent in the R. nomadica gut samples. If radiolarians were gelatinous (indeed, many are) it may be that this taxon was ingested but that its remains were not recognizable in the gut material. On the other hand, it is possible that medusae are not ‘passive feeders’ and are able to select which plankters they ingest and digest in the surrounding waters. In a laboratory culture of young R. nomadica that were offered Artemia sp., dozens of medusae (2–3 cm in diameter) fed on the artemia prey, yet one individual was observed swimming through the ‘cloud’ of prey without capturing any brine shrimp on its feeding appendages or in its gastric tracts. This behaviour repeated itself during several feeding sessions (for 48 h) until this specimen started feeding like all other individuals in the aquarium (Z. Kuplik, unpublished observations). As mentioned above, perhaps medusae can choose whether or not they capture available prey, but in order to test this accurately, additional field sampling and controlled feeding trials must be conducted. An intriguing dominant prey found within the gut contents were ‘various eggs’ of unknown organisms. Since copepods are the most abundant taxon in the plankton, the eggs may have been ingested by the medusae while consuming copepods with egg clutches as by-catch. However, whether or not the eggs were copepod eggs, their large number in the gut contents supports our finding of selectivity for prey at the micro-scale.
In general, the gut contents of larger R. nomadica contained larger (and faster) prey (i.e. adult copepods and appendicularians, Table 5). This corresponds with the findings of Costello & Colin (Reference Costello and Colin1994, Reference Costello and Colin1995) and D'Ambra et al. (Reference D'Ambra, Costello and Bentivegna2001) who showed that the velocity of the marginal flow, i.e. the flow around the bell margins created by bell pulsation, was positively correlated with the size of the medusa. Assuming that medusae can only capture prey with escape speeds slower than the marginal flow velocity, this should enable larger medusae to capture faster prey. Contrary to expectation, however, harpacticoids (rapidly moving copepods) in gut contents were negatively correlated with medusa bell diameter. This anomaly was observed in a plankton sample collected on 22 July 2015, with an unusually large number of harpacticoids in the plankton and in the medusae gut content samples when medusae were mostly small (16 cm mean bell diameter). But, since medusa body size is only one of several variables affecting prey capture and since other factors, such as predator and prey behaviour and contact rate (Graham & Kroutil, Reference Graham and Kroutil2001) were not assessed in the present study, the above assumption could not be ascertained.
Another intriguing observation was the consistent decrease in mean bell diameter of R. nomadica observed and captured during the 5 weeks of this study. Other scyphomedusae (e.g. Rhopilema esculentum, Aurelia aurita and Pelagia noctiluca) have also been observed to decrease in size over time, and a decrease in available food was the most common explanation for this phenomenon (Hamner & Jenssen, Reference Hamner and Jenssen1974; You et al., Reference You, Ma, Gao, Li, Zhang, Qiu and Wang2007). In a detailed study of Pelagia noctiluca, Lilley et al. (Reference Lilley, Ferraris, Elineau, Berline, Cuvilliers, Gilletta, Thiery, Gorsky and Lombard2014) suggested that insufficient prey availability, coupled with high metabolic rates due to temperature-dependent functions such as pulsation and respiration (Morand et al., Reference Morand, Carre and Biggs1987; Malej, Reference Malej1989) and intense reproductive efforts could all be involved in the decrease in medusa size. Since R. nomadica is considered a tropical invasive species (Galil et al., Reference Galil, Spanier and Ferguson1990), it is unlikely that the seawater temperatures recorded during this study (25.5–28.1°C) were involved in the decrease in bell diameter as a result of metabolic stress. In addition, abundances of key plankton prey did not decrease during this period, as observed in the plankton tow samples (Table 1; Figure 4). One factor that could be associated with the reduction in medusa size is the advanced reproductive state of the sampled medusae, since sexual maturity may be correlated with the degeneration of feeding structures following spawning (Fancett, Reference Fancett1986). Indeed, many of the medusae observed were shown to lack part or even all of their feeding structures (see Supplementary Figure S1), especially during the last two excursions in this study. It is possible that the reduction in size was related to increased energy investment in reproduction, a loss in feeding efficiency (reduced feeding structures) or is an indication that R. nomadica is a senescent species where sexual reproduction is followed by death (Boero et al., Reference Boero, Bouillon, Gravili, Miglietta, Parsons and Piraino2008; Uye, Reference Uye2008). This hypothesis (i.e. senescence) seems to be supported by the large number of dead medusae and medusa parts washed ashore towards the end of the blooming period. However, lack of additional data prevents us from drawing such a conclusion at this stage.
Conclusions
The findings of this study provide new information toward our understanding of the ecology and the role R. nomadica plays in the eastern Mediterranean ecosystem. Despite massive swarms of this species, it is not clear that it depletes plankton stocks indiscriminately, as might be assumed based simply on the conventional diet of medusae. The apparent preferential feeding of R. nomadica on prey smaller than 150 μm, at least at the stage of sexual maturity, should diminish its role as a significant predator of fish eggs and larvae and thus as a major competitor with large fish which rely on mesozooplanktonic rather than microplanktonic prey. However, it may compete over smaller prey with organisms such as larval fish, the survival of which depends on microscopic food due to their small mouth opening (Kohno et al., Reference Kohno, Ordonio-Aguilar, Ohno and Taki1997; Glamuzina et al., Reference Glamuzina, Skaramuca, Glavic and Kozul1998; Hagiwara et al., Reference Hagiwara, Wullur, Marcial, Hirai and Sakakura2014). In light of the notable observed changes in the medusae (i.e. gonadal maturation, loss of feeding structures and the reduction in bell size), and the fact that a large proportion of the medusae are washed to shore at the end of the annual swarming events (Edelist et al., Reference Edelist, Guy-Haim, Kuplik, Zuckerman, Nemoy and Angel2020), we suggest that R. nomadica is a sexually senescent species. The loss of feeding apparatus could imply that during the time of this study the medusa were not feeding to their full potential.
In summary, through this study we have managed to shed some light on the diet and feeding preferences of the rhizostome R. nomadica. Nevertheless, more study is required: year-round gut content sampling (e.g. inspection of intact and possibly less sexually mature medusae), feeding trials in order to obtain zooplankton species-specific digestion times, night-time sampling to examine a possibly different diet composition and plankton tows at the micro and meso size scale. Additionally, by applying techniques such as stable isotopes and fatty acids, we may identify other sources to the medusae diet that are not detectable by gut content analyses (Pitt et al., Reference Pitt, Clement, Connolly and Thibault-Botha2008). All these could provide us with valuable information for a better resolution of R. nomadica's role and trophic position in the marine food web.
Supplementary material
The supplementary material for this article can be found at https://doi.org/10.1017/S0025315420000697
Acknowledgements
First and foremost, we would like to thank Dr Dani Kerem who contributed greatly to this study. This study would not have been possible without the tremendous help of the Maritime Aquaculture Department staff, Mevoot-Yam School, Michmoret; Rafi Yavetz, Gal Belogolovsky, Arik Weinberger, Ifat Mazoz and David Halfon, who assisted in so many ways. We would also like to thank Prof. Gitai Yahel for assisting with the statistics, Dr Itzchak Brickner for his help with histology, and the School of Marine Sciences, Ruppin Academic Center, for providing marine laboratory facilities.
Financial support
The authors gratefully acknowledge support from the University of Haifa, the Helmsley Charitable Trust Mediterranean Sea Research Center, and the Maurice and Lady Hatter Fund of the Leon Recanati Institute for Maritime Studies (RIMS) at the University of Haifa.