INTRODUCTION
Acute gastroenteritis (AG) is considered as a cause of morbidity and mortality worldwide. Group A rotavirus (GAR), calicivirus, human astrovirus (HAstV) and enteric adenovirus (AdV) have been recognized as major aetiological agents [Reference Glass1]. However, many of the AG cases remain undiagnosed. Recently, other enteric viruses such as picobirnavirus, torovirus and Aichi virus have been also shown to cause AG [Reference Wilhelmi de Cal2]. Aichi virus of the genus Kobuvirus, family Picornaviridae was first isolated in 1989 from a Japanese patient and reported to cause oyster-associated non-bacterial gastroenteritis [Reference Yamashita3]. The complete nucleotide sequence of Aichi virus was described in 1998 [Reference Yamashita4]. The genome consists of a single-stranded positive-sense RNA molecule of 8251 nt excluding a poly (A) tail. The single open reading frame of 7302 nt encodes a polyprotein of 2433 amino acids that is cleaved into structural proteins VP0, VP3, VP1 and non-structural proteins 2A, 2B, 2C, 3A, 3B, 3C and 3D [Reference Yamashita4]. Three genotypes A, B and C of Aichi virus have been identified on the basis of phylogenetic analysis of the 3C–3D junction and VP1 regions of the virus genome [Reference Yamashita5, Reference Ambert-Balay6].
Aichi virus was detected at low (0·5–4·1%) prevalence rates with its distribution mainly in A and B genotypes in countries from Asia, South America, Africa and Europe [Reference Ambert-Balay6–Reference Sdiri-Loulizi10]. However, seroprevalence of antibodies to the Aichi virus has been estimated to be 55–76%, with an increase up to 95% with age [Reference Oh8, Reference Yamashita11, Reference Sdiri-Loulizi12]. Thus the epidemiology of AG caused by Aichi viruses is not clearly understood. This report documents for the first time prevalence and genotype distribution of Aichi virus strains detected in AG patients from India.
METHODS
Clinical specimens
In a 5-year retrospective study, a total of 1240 faecal specimens were collected from children aged ⩽8 years hospitalized for AG in Pune, Aurangabad and Nagpur, three cities in Maharashtra state, western India. These included 912 specimens from Pune (January 2004–December 2008), 100 from Aurangabad (May 2005–February 2006) and 228 from Nagpur (June 2006–August 2007).
All patients were examined for fever, number of episodes, and duration of vomiting and diarrhoea, extent of dehydration, and treatment for the assessment of severity of disease by a 20-point scale of the Vesikari scoring system [Reference Ruuska and Vesikari13].
RNA extraction and RT–PCR
Viral RNA was extracted from 30% (v/v) stool suspension using QIAamp Viral RNA kit (Qiagen Co., Germany) according to the manufacturer's instructions.
Presence of Aichi virus RNA in faecal specimens was determined by using nested RT–PCR targeting the 3C–3D junction region [Reference Yamashita5]. The nested PCR was performed using the outer F6261 and R6779 primers (F5′-ACACTCCCACCTCCCGCCAGTA-3′, R5′-GGAAGAGCTGGGTGTCAAGA-3′) to amplify a 519-bp segment and the inner FC94b and R246k primers (F5′-GACTTCCCCGGAGTCGTCGTCT-3′, R5′-GACATCCGGTTGACGTTGAC-3′) to amplify a 265-bp segment within the 3C–3D region (nucleotide positions as per the strain A846/88, accession no. AB010145). Further genotyping of the Aichi virus was performed by amplifying the VP1 region (699 bp) of the viral genome using F2954–R3636 (F5′-GGTGAATCCTTCAACGTACG-3′ and R5′-GCAAGAGAGCTGGAAGT-3′) primer set as reported earlier [Reference Ambert-Balay6]. Briefly, cDNA was synthesized using AMV RT (Promega, USA, 10 U/μl) at 42°C for 1 h with final inactivation of the enzyme at 95°C for 5 min. PCR was performed in 50 μl reaction mix containing 5 μl template, 10x Taq DNA polymerase buffer, dNTPmix (10 mm), primers (30 pmol/μl), 0·25 μl Taq DNA polymerase (5 U/μl Roche, Germany) and the volume was made up with distilled water (Milli Q water, Bangalore Genei Pvt. Ltd, India). The thermal cycling conditions were kept same for all sets of primer which consisted of an initial denaturation at 94°C for 4 min followed by 35 cycles at 94°C for 1 min, 55°C for 30 s and 72°C for 1 min with final extension at 72°C for 7 min. In the second round of PCR, 2 μl of the first PCR product was added to a total volume of 50 μl reaction mix containing inner set of primer.
The PCR products were electrophoresed on 2% agarose gels containing 0·01 mg/μl ethidium bromide and visualized under UV transillumination. All of the PCR-positive products were excised from the gel and purified by QIAquick gel extraction kit (Qiagen).
Sequencing and phylogenetic analysis
Sequencing was performed using the BigDye Terminator v. 3.1 cycle sequencing kit and ABI 3130XL Genetic Analyzer (Applied Biosystems, USA). Nucleotide identity of the sequenced amplicons was determined through BLAST analysis (www.ncbi.nlm.nih.gov/blast) followed by sequence alignment with reference strains available in the GenBank database using CLUSTAL W program. The phylogenetic tree was constructed using the neighbour-joining algorithm and Kimura two-parameter distance model and tested by applying the bootstrap test with 1000 bootstrap replications using MEGA 4 software [Reference Tamura14]. The nucleotide sequences reported in this study have been deposited in GenBank under the accession numbers HM362992–HM363005 and HM363006–HM363011 for the 3C–3D junction and VP1 regions, respectively.
Detection of other enteric viruses
All of the faecal specimens (n=1240) were investigated for the presence of GAR by rotavirus antigen detection ELISA [Reference Kelkar15], and norovirus (NoV), AdV and HAstV, by RT–PCR/PCR using RdRp gene, hexon gene and ORF1a region-specific primers, respectively.
RESULTS
Aichi virus RNA was detected in 1·3% (12/912), 1% (1/100), and 0·4% (1/228) of the faecal specimens from children with AG from Pune, Aurangabad and Nagpur, respectively, with an overall prevalence of 1·1%. Aichi virus-infected children included 64·3% (9/14) males and 35·7% (5/14) females. Of these children, co-infections with GAR and HAstV were identified in 14·3% (2/14) and 7·1% (1/14), respectively. The highest Aichi virus positivity (35·7%) was detected in children aged between 25 and 36 months. During the 5-year study period, the infection rate was found to be higher in June and July while no infection was detected during January–May. Aichi virus-positive children suffered from moderate 57·1% (8/14) and severe 42·9% (6/14) AG infections.
Phylogenetic analysis of the sequences obtained for the 3C–3D junction region of the 14 strains of Aichi virus showed 94·2–99·5% nt identity with genotype B strains [B-171/05 (EF079157), Qld/2008/204 (EU715251)] isolated in Bangladesh and Australia. Six of the 14 strains could be amplified by RT–PCR of the VP1 gene. Sequencing of the amplicons revealed 92·6–98·3% nt identity with genotype B strain B-302 (EU143276) from Bangladesh (Fig. 1 a, b).
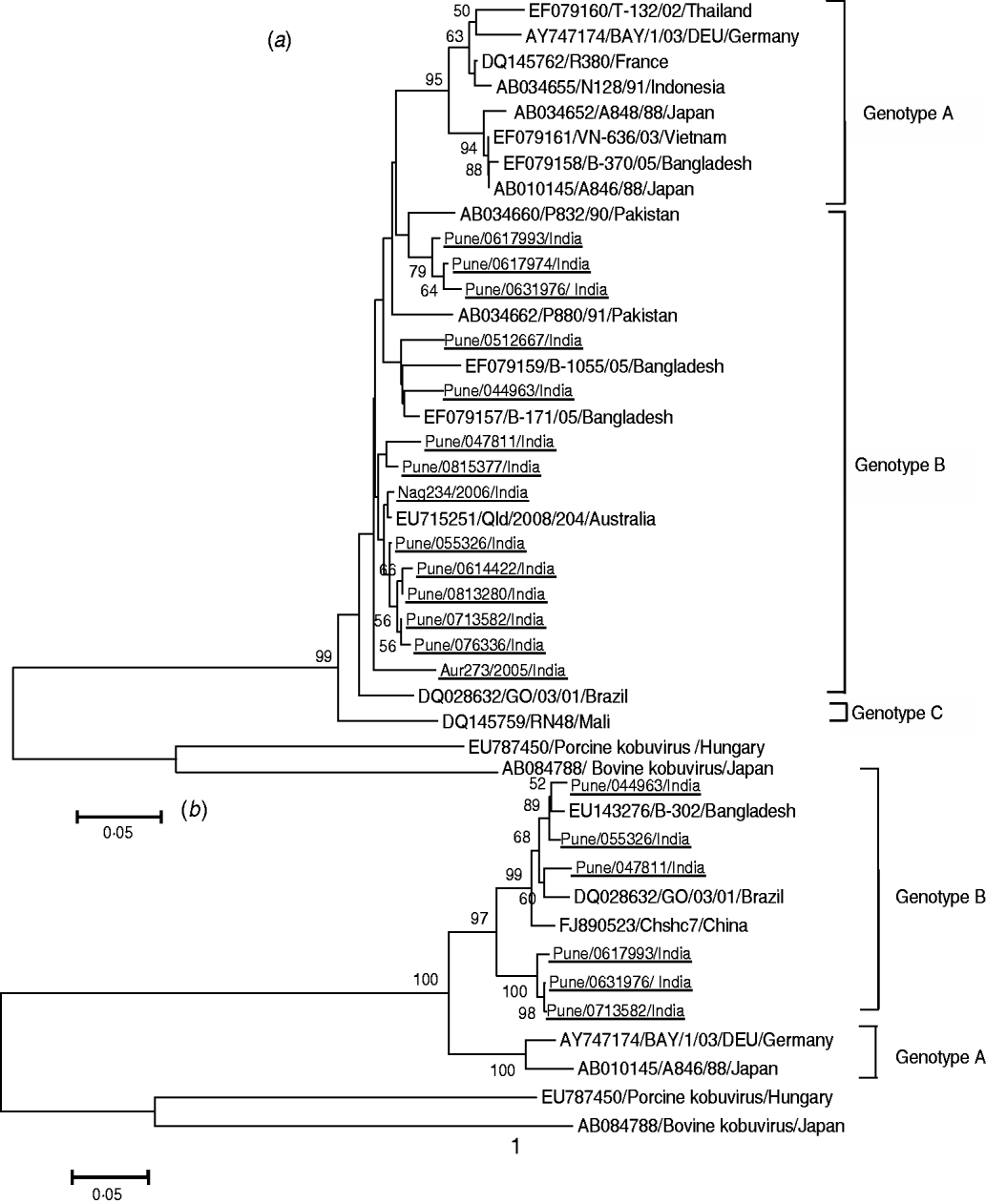
Fig. 1. Phylogenetic trees of Indian Aichi virus strains based on (a) partial nucleotide sequences of 3C–3D junction region (200 bp) and (b) VP1 region (630 bp). The study strains are underlined. All strains are identified by abbreviations – accession number or name of city/strain name/country name. Nag, Nagpur; Aur, Aurangabad.
DISCUSSION
The study describes for the first time the detection of Aichi virus genotype B strains in AG patients from India. Overall, the detection rate of Aichi virus was variable in the study regions (0·4–1·3%) and significantly low compared to other enteric viral aetiological agents GAR (36%), NoV (8·6%), AdV (6·2%) and HAstV (3·1%) of AG in the same set of specimens and/or previously reported from India [Reference Kang16–Reference Verma19]. These observations are in accord with those of earlier studies describing similar incidences of enteric viruses from countries in Asia, Europe and South America and differed from Aichi virus mono infections demonstrated in Tunisia [Reference Yamashita7–Reference Sdiri-Loulizi10, Reference Reuter20, Reference Kaikkonen21]. GAR, NoV, AdV and HAstV infections have been reported to be significantly higher in the first 2 years of life [Reference Kang16–Reference Verma19]. On the contrary, in the present study Aichi virus infections were more prevalent in children aged 2–3 years. In western India, GAR and HAstV infections prevailed in winter months, NoV infections were higher in summer months and AdV infections continued to occur through out the year. By comparison, Aichi virus infections identified in the present study peaked in the rainy season [Reference Kang16–Reference Verma19].
VP1 sequence comparisons have been described as a valuable criterion in classification of picornaviruses [Reference Oberste22]. Hence, phylogenetic analysis of the VP1 coding region of Aichi virus strains was performed. The distribution of the strains according to the VP1 sequences was similar to that obtained using analysis of the 3C–3D junction region indicating utility of both genomic regions for typing. While the Aichi virus strains from Japan and Europe were placed in genotype A and those from Africa in genotype C, all Indian strains were closer to genotype B strains from Australia and Bangladesh indicating circulation of genetically similar Aichi virus strains in these countries [Reference Ambert-Balay6–Reference Sdiri-Loulizi10, Reference Kaikkonen21].
In the present study, children (n=153) hospitalized for reasons other than AG were detected negative for Aichi virus infections (data not shown). Moreover, a considerable proportion of co-infections with other enteric viral pathogens in Aichi virus-positive children were also noted. These data emphasize the need for further studies on epidemiology, transmission pattern and pathogenesis of Aichi virus to ascertain its aetiological role as a viral pathogen in AG.
ACKNOWLEDGEMENTS
The authors are grateful to the Indian Council of Medical Research (ICMR) Delhi, India for providing financial support to the first author and to Dr A. C. Mishra, Director, National Institute of Virology, Pune for constant support during this study. We also thank Mrs Sujata S. Ranshing for her technical support.
DECLARATION OF INTEREST
None.