Introduction
Serpulids dwell on various hard substrata in all marine habitats at all depths (Kupriyanova et al., Reference Kupriyanova, Vinn, Taylor, Schopf, Kudryavtsev and Bailey-Brock2014). They are important fouling organisms and some species can also form reefs at different marine environments from the intertidal to the bathyal (ten Hove and van den Hurk, Reference ten Hove and van den Hurk1993; Bianchi and Morri, Reference Bianchi and Morri2001; Sanfilippo et al., Reference Sanfilippo, Vertino, Rosso, Beuck, Freiwald and Taviani2013; Ippolitov et al., Reference Ippolitov, Vinn, Kupriyanova and Jäger2014; Bastida-Zavala et al., Reference Bastida-Zavala, McCann, Keppel and Ruiz2017; Guido et al., Reference Guido, Jimenez, Achilleos, Rosso, Sanfilippo, Hadjioannou, Petrou, Russo and Mastandrea2017). There are 48 genera of serpulids with about 374 extant species (ten Hove and Kupriyanova, Reference ten Hove and Kupriyanova2009; Kupriyanova et al., Reference Kupriyanova, ten Hove and Rouse2023). All serpulids build calcareous tubes that are internally covered by a thin organic layer (Nishi, Reference Nishi1993), but they lack an external organic layer, such as the periostracum of molluscs and brachiopods. Serpulid tubes can be almost smooth showing only faint growth striations or variably sculptured with transverse, longitudinal ridges and peristomes (ten Hove, Reference ten Hove1973, Reference ten Hove1975; Sanfilippo and Mòllica, Reference Sanfilippo and Mòllica2000; Bastida-Zavala and ten Hove, Reference Bastida-Zavala and ten Hove2002; Sanfilippo, Reference Sanfilippo2009a, Reference Sanfilippo2009b). Growth lamellae of serpulids are thin, and are typically chevron shaped in longitudinal section (Weedon, Reference Weedon1994) or, more rarely as straight bands (Sanfilippo, Reference Sanfilippo and Cherchi1996). At the contact between the lateral tube wall and the substrate, the tube of some species contains cavities (e.g. Hedley, Reference Hedley1958). The mineral composition of serpulid tubes is either calcitic, aragonitic or a combination of both polymorphs (Vinn et al., Reference Vinn, ten Hove, Mutvei and Kirsimäe2008; Smith et al., Reference Smith, Riedi and Winter2013). Serpulid tubes can be single layered or composed of up to four layers with distinct microstructure (Vinn et al., Reference Vinn, ten Hove, Mutvei and Kirsimäe2008).
Growth lines can be defined as abrupt or repetitive changes in the character of an accreting tissue (Clark, Reference Clark1974). In molluscs, microgrowth increments are associated with alternating regions containing variable concentrations of organic materials and same would be expected for serpulid growth increments. The microgrowth patterns in molluscan shells are well known and constitute valuable tool for reconstructing habitats and they can also yield information about population dynamics (Dauphin et al., Reference Dauphin, Cuif and Williams2003). However, the distribution of growth lines in serpulid tubes has previously not been studied.
The aims of the paper are to: (1) find similarities and differences in the frequency of growth lines in Recent and fossil serpulid tubes, (2) find whether frequency of growth lines is different in warm and cold water, (3) find whether large serpulid species are growing faster than small ones and (4) find whether the frequency of growth lines depends on the tube microstructure.
Material and methods
The Recent and fossil serpulid tubes (Table 1) were cut using small electrical saw, oriented and mounted in epoxy resin for machine grinding. Five- to ten-millimetre-long longitudinal sections and single cross-sections were prepared. Sectioned tubes were polished with polishing machine and etched in a 1% solution of acetic acid for 5 min. The sample was mounted on an aluminium stub and gold sputtered prior to SEM study with a Hitachi S-4300 Field Emission Scanning Electron Microscope at 2 kV. The fossils were scanned in a back-scattered electron imaging mode. All the SEM images were digitally acquired. The SEM study was carried out at the Swedish Museum of Natural History. The growth increments were counted in calibrated digital photos of longitudinal section of the tube within 50 μm in two sections for each species. An online Pearson correlation coefficient calculator was used to find correlation between frequency of growth lines and the average tube diameter. In order to check the statistical differences in the frequency of growth lines between warm water and cold water species, a non-parametric Mann–Whitney test was used (a result is significant if P < 0.05). Same test was also used to find statistical differences in the frequency of growth lines between different tube microstructures. Vinn et al. (Reference Vinn, ten Hove, Mutvei and Kirsimäe2008) terms for serpulid microstructures were used.
Table 1. Growth lines in serpulid tubes

Results
There are 7–37 growth lines per 50 μm in the tube wall of Recent serpulids (Table 1). In the Mesozoic serpulids, there are 15–25 growth lines per 50 μm. In the Cenozoic serpulids, there are 5–20 growth lines per 50 μm (Table 1). All the known Paleozoic serpulids have not been examined in this work because their tube walls have internal structure almost completely obliterated by diagenesis (Sanfilippo et al., Reference Sanfilippo, Rosso, Reitano and Insacco2017, Reference Sanfilippo, Rosso, Reitano, Viola and Insacco2018). The Pearson correlation coefficient calculator found that there is a moderate negative correlation between frequency of growth lines and the average tube diameter (R = −0.6877; P-value = 0.006641; the result is significant at P < 0.05), so that the larger tubes tend to have fewer growth lines per 50 μm. There are 10–36 growth lines per 50 μm in cold water serpulids and 9.5–35 growth lines in warm-water serpulids. Mann–Whitney U test calculator did not find significant difference in the frequency of growth lines between warm water (N = 11) and cold water (N = 6) serpulids (U-value = 32.5, critical value of U at P < 0.05 is 13 and therefore, the result is not significant at P < 0.05; z-score = 0, P-value = 1 and the result is not significant at P < 0.05). There are 6–36 growth lines per 50 μm in prismatic structures (Figures 1A, B, 2), 5–22.5 in lamello-fibrillar (LF) structures (Figure 1C), 10–35 in irregularly oriented prismatic (IOP) structures (Figure 1D), 13 in a spherulitic irregularly oriented prismatic (SIOP) structure and seven in spherulitic lamello-fibirallar (SLF) structure. Mann–Whitney U test calculator found that growth lines are significantly more frequent in 11 species with prismatic structures than in eight species with LF structures. The U-value is 16. The critical value of U at P < 0.05 is 19. Therefore, the result is significant at P < 0.05. The z-score is 2.27074. The P-value is 0.0232. The result is significant at P < 0.05.

Figure 1. (A) Placostegus tridentatus (Bergensfjord, Norway), longitudinal section through a prismatic structure. (B) Pentaditrupa subtorquata (Cretaceous, Germany), longitudinal section through a prismatic structure. (C) Crucigera websteri (shelf of Surinam), longitudinal section through LF structure. (D) Serpula crenata (Indonesia), longitudinal section through IOP structure. Arrows pointing to growth lines.
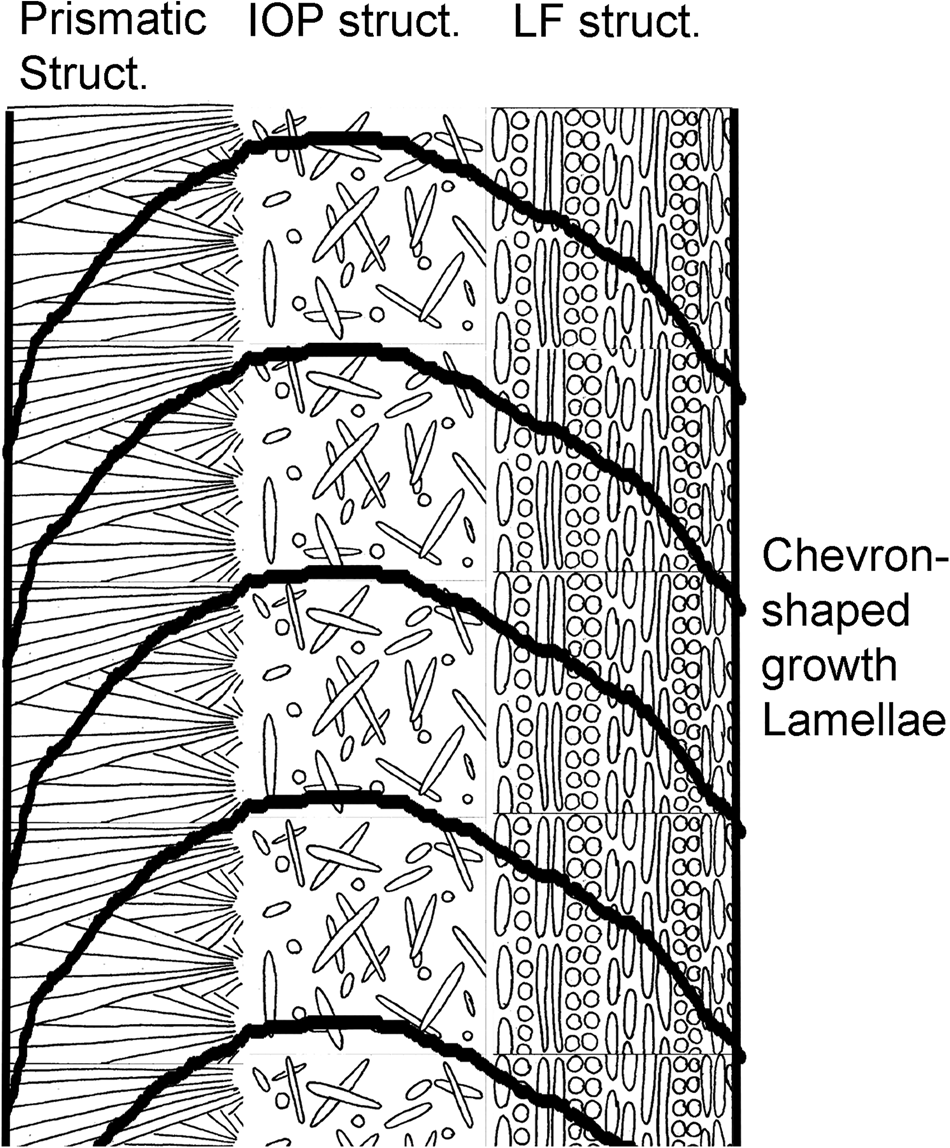
Figure 2. Schematic drawing of growth lines in a serpulid tube wall with multiple microstructures.
Discussion
Temperature
Ambient temperature is the most important variable controlling metabolic rate, energy expenditure, majority of physiological processes and thus growth and development among ectotherms such as molluscs (Atkinson, Reference Atkinson1993; van der Have and de Jong, Reference Van der Have and de Jong1996; Broell et al., Reference Broell, McCain and Taggart2017; Saulsbury et al., Reference Saulsbury, Moss, Ivany, Kowalewski, Lindberg, Gillooly, Heim, McClain, Payne, Roopnarine, Schöne, Goodwin and Finnegan2019). The effect of temperature on growth rate can depend on the time of exposure to the high temperatures in molluscs (Nielsen, Reference Nielsen1988). The instant response to temperate exposure is an increase in shell-length growth with temperature in Mytilus edulis. However, during the acclimation period, shell-length growth changes, and for acclimated mussels length-growth rate decreases with increasing temperature so that the maximum shell-length growth is recorded at the lowest experimental temperatures (8–12°C) (Nielsen, Reference Nielsen1988). Study of shell growth in bivalve mollusc Scapharca broughtonii has shown that that rearing temperature significantly affects the shell growth increments (Nishida et al., Reference Nishida, Suzuki, Isono, Hayashi, Watanabe, Yamamoto, Irie, Nojiri, Mori, Sato, Sato and Sasaki2015). There is a negative correlation between temperature and the relative thickness of the composite prismatic structure in S. broughtonii specimens. The relationship curve between temperature and the growth increment of the composite prismatic structure was hump-shaped in S. broughtonii, with a maximum at 17.8°C (Nishida et al., Reference Nishida, Suzuki, Isono, Hayashi, Watanabe, Yamamoto, Irie, Nojiri, Mori, Sato, Sato and Sasaki2015). The growth increments of studied serpulids do not show correlation with the water temperature and presumably cold water and warm water serpulid species are growing on average with similar speed. This could mean that there are no evolutionarily advantageous general growth rates for cold or warm climate serpulids. The lack of correlation between the width of the growth increments and the temperature, as observable in Table 1, suggests that frequency of growth lines is a specific character. In bivalve molluscs, growth coefficients exhibit moderate phylogenetic signal, and taxonomic membership is a stronger predictor of growth coefficient than any environmental predictor, but there is still strong environmental control on growth rates within taxa (Saulsbury et al., Reference Saulsbury, Moss, Ivany, Kowalewski, Lindberg, Gillooly, Heim, McClain, Payne, Roopnarine, Schöne, Goodwin and Finnegan2019). The situation in serpulids could be similar. However, studied collection did not enable to explore the possible influence of temperature on the growth increments within any particular serpulid species. Thus, possibility that temperature affected growth rates of studied serpulid species cannot be ruled out. In Otago Harbour, tube growth in Galeolaria hystrix and Spirobranchus cariniferus is slower in winter compared to summer and slows with serpulid age (Riedi, Reference Riedi2012). The future studies should try to compare the growth increments of each single species both from cold and warm localities (i.e. for instance comparing populations of a species from different sites in its distributional range) to highlight whether the temperature actually has an influence on the growth rate and therefore on the width of the lamellae in most serpulids.
Size of serpulids
Nishi and Nishihira (Reference Nishi and Nishihira1996) used growth increments of host coral to estimate life span of large endobiotic serpulid Spirobranchus. They found that Spirobranchus lived within corals usually more than 10 years and sometimes more than 40 years. There are only a little data available on the growth speed in serpulids. Sanfilippo et al. (Reference Sanfilippo, Vertino, Rosso, Beuck, Freiwald and Taviani2013) provide measures of the total length of S. vermicularis individuals encrusting panels from deep waters in the NW Mediterranean that allowed to estimate a maximum tube growth rate of 25 mm year−1 at least for the first life stages, when individuals usually grow faster. The largest S. vermicularis specimens, whose tubes are up to 20 cm long, could be about 8 years old (Sanfilippo et al., Reference Sanfilippo, Vertino, Rosso, Beuck, Freiwald and Taviani2013). In Scottish sea lochs, yearly growth increments of S. vermicularis are on average 32.0 mm long (Hughes et al., Reference Hughes, Poloczanska and Dodd2008). In Otago Harbour both serpulids are believed to live as long as about 10–12 years, during which G. hystrix produces a tube of ~21 cm and S. cariniferus a tube of ~11 cm in length (Riedi, Reference Riedi2012). Mean annual tube growth for larger G. hystrix is 4.1 cm (range 2.0–6.7 cm) and 1.7 cm (range 0.4–3.4 cm) for smaller S. cariniferus (Riedi, Reference Riedi2012). Mean annual calcification rates are also greater in larger species, 1.5 g year−1 for G. hystrix and 0.3 g year−1 for S. cariniferus individuals in Otago Harbour (Riedi, Reference Riedi2012). Our data show that large serpulid species usually have significantly larger growth increments than smaller species and presumably also grew faster than smaller species. Thus, serpulids that attained large adult sizes usually also followed a strategy of fast growth. Theoretically, a slow growth strategy that forages infrequently also accumulates low but constant predation risk contrasts to a fast growth strategy that forages frequently and experiences a high early predation risk in return for lower future predation risk and enhanced fecundity (Urban, Reference Urban2007). Slow strategies generally are advantageous in communities dominated by gape-unconstrained predators whereas fast strategies are advantageous in gape-limited predator communities (Urban, Reference Urban2007). Thus, it is possible that fast growing large serpulids may have an evolutionary advantage over small species in gape-limited predator communities. Di Geronimo et al. (Reference Di Geronimo, Di Geronimo, La Perna, Rosso, Sanfilippo and Hart2000) found in a study of cold Pleistocene invertebrate fauna that larger-sized serpulids occur as a response of low temperatures. Thus, if the cold climate favours large size in serpulids, it is possible that a large sample of serpulids from warm and cold climates could reveal a difference in average thicknesses of growth lines.
Prismatic vs LF structure
Serpulid tubes with LF structure are relatively porous in studied sections under SEM and due to that they likely have a lower skeletal density than tubes with prismatic structure (Vinn and Kupriyanova, Reference Vinn and Kupriyanova2011). The latter appear to be much denser and less porous than tubes with LF structure in studied sections under SEM. Thus, it is not surprising that denser skeletal structures such as prismatic structures have lower growth rates (i.e. mean 18.6 growth lines per 50 μm) than more porous skeletal structures such as LF structure (i.e. mean 10.1 growth lines per 50 μm). It is possible that serpulids do not have to calcify faster to produce thicker growth increments with lower density. Future studies should show whether this is a part of a larger pattern or valid only in case of LF and prismatic structure.
Fossil vs Recent serpulids
The frequency of growth increments in the tube wall of few Mesozoic serpulids (i.e. 15–25 growth lines per 50 μm) is in the range of modern species (i.e. 7–37 growth lines per 50 μm). Similarly, values of fossil serpulids from Cenozoic (5–20 growth lines per 50 μm) do not differ from those of modern species. This indicates that growth lines preserve in fossil material and can be counted in fossil serpulids with high confidence. These results also suggest that there has not been any drastic change in the process of producing growth increments in serpulids from the Jurassic to Recent. If the serpulid growth lines constitute a good proxy to the speed of growth then our results also suggest that the growth speed of serpulids has not drastically changed from the Jurassic to Recent. The unchanged growth speed also suggests that ecology and biomineralization of the Mesozoic and Cenozoic serpulids was not much different from that of Recent species.
Data availability
SEM images are available on request.
Acknowledgements
I am grateful to Harry A. ten Hove for providing me with the tube samples of Recent serpulids. I am grateful to two anonymous reviewers for the constructive comments on the manuscript.
Author contributions
The sole author of this paper is Olev Vinn.
Financial support
This research was funded by a Sepkoski Grant from Paleontological Society.
Competing interest
None.
Ethical standards
I have followed best practices of marine biological research.