α-Glucans such as starch, glycogen and dextrin, consisting predominantly of α-1,4 linkages of glucose residues and partial α-1,6 linkages, are carbohydrates that provide an important source of energy for humans. α-Glucans are readily catabolised in the small intestine, especially the duodenum and the jejunum, by an elaborate system for carbohydrate digestion(Reference Gray1). After this process is completed, digested α-glucans are immediately absorbed(Reference Gray1). However, the digestion rate of α-glucan is affected by certain factors, including the type of glucose linkage and the degree of polymerisation. Starch is categorised into three types by its rate of digestion: (1) rapidly digestible starch, (2) slowly digestible starch and (3) digestion-resistant starch(Reference Englyst, Kingman and Cummings2,Reference Englyst, Englyst and Hudson3) . Previous work showed that administration of rapidly digestible α-glucan to an experimental model led to an immediate increase in glucose and insulin levels in the blood and hence, α-glucan rarely reached the distal small intestine, the ileum. Furthermore, in other studies, it was found that diets with a high glycaemic load in the form of rapidly digestible α-glucans increased the risk of type 2 diabetes and CHD(Reference Liu, Willett and Stampfer4–Reference Salmeron, Ascherio and Rimm6). By contrast, administration of diets that contain large amounts of slowly digestible α-glucan seems to prevent development of diabetes via suppression of insulin resistance(Reference Blaak, Antoine and Benton7). Hence, regulation of the digestion rate of α-glucans in the small intestine is crucial for human health, as the slow absorption rate of glucose, a desirable effect, would depend on the digestion rate of α-glucans.
α-Glucosidic linkages can be almost completely degraded by α-amylase in saliva and the pancreatic juice and mucosal α-glucosidases such as maltase and isomaltase(Reference Lee, Rose and Lin8). However, degradation by these enzymes of α-1,3 and α-1,6 glucosidic linkages is slower than that of α-1,4 glucosidic linkages(Reference Lee, Rose and Lin8,Reference Sim, Willemsma and Mohan9) . Therefore, digestion of α-1,3 and α-1,6 linkage-rich α-glucans could potentially be extended to the ileum because the transit time through the small intestine would not be long enough to completely digest these α-glucans. In other words, slowly digestible α-glucans and digestion-resistant α-glucans, to name a few, would be able to leave the small intestine partially undigested and thus, being able to reach the large intestine. Past work provided insight into the chemical nature of their α-glucosidic and β-glucosidic linkages(Reference Hamaguchi, Hirai and Aizawa10–Reference Lahtinen, Knoblock and Drakoularakou12) that can be used to find or synthesise slowly digestible and/or resistant α-glucans. Such polysaccharides could then become important sources of energy for humans without exerting adverse health effects such as obesity and diabetes.
Isomaltodextrin (IMD), an α-glucan averaging a molecular weight of 5000 and a degree of polymerisation (DP) of 30, is produced from starch using enzymes α-glucosyltransferase and α-amylase derived from Paenibacillus alginolyticus PP710. IMD is a highly branched dextrin with an α-glucosidic bonding structure consisting of 3, 49, 19, 7 and 5 % of α-1,3; α-1,6; α-1,4; α-1,3,6 and α-1,4,6 linkages, respectively, as well as a 17 % non-reducing end(Reference Tsusaki, Watanabe and Nishimoto13). Previously, we found that rats fed IMD had a high concentration of SCFA in caecum(Reference Nishimura, Tanabe and Yamamoto14), demonstrating that a high proportion of α-1,3 and α-1,6 linkages was the cause for the partial resistance of IMD glucose to digestion and absorption in the small intestine of rats. Nonetheless, at the time, we were unable to determine the digestibility of IMD and the absorption rate of its glucose in the small intestine.
Secretion of glucagon-like peptide-1 (GLP-1), an incretin hormone, could be increased by delivery of glucose to the ileum because it has been shown that GLP-1 is produced and secreted upon stimulation of nutrients such as glucose, amino acids, peptides and lipids readily made available by L cells densely present in the ileum(Reference Tolhurst, Reimann and Gribble15,Reference Spreckley and Murphy16) . GLP-1 can greatly influence glucose homeostasis by exerting physiological effects, for example, increased biosynthesis and secretion of insulin in the pancreas, increased insulin sensitivity in muscles and decreased gastric emptying(Reference Baggio and Drucker17). In this context, regulation of GLP-1 secretion can be considered a process highly contributing to improved human health. For example, stimulating an increase in GLP-1 secretion by delivering glucose of slowly digestible α-glucans to the ileum could help improve glucose tolerance in the recipient thus minimising the risk of development of chronic diseases.
In the present study, to elucidate the effect of IMD with a high proportion of α-1,3 and α-1,6 linkages on the gut physiology, we measured the digestibility of IMD and absorption of its glucose in the small intestine of rats. In addition, we investigated the effect of slow delivery of IMD glucose to the small intestine, on GLP-1 secretion.
Materials and methods
Samples
IMD (commercial name: Fibrixa; digestibility 15 % as per the AOAC2001.03 method), high-amylose maize starch (HAMS, Class 7, 70 % high amylose content, 41 % resistant starch content and 52 % digestibility in rat small intestine(Reference Nishimura, Tanabe and Yamamoto18)), isomalto-oligosaccharides (IMO; digestibility 35 % as per AOAC 2001.03 method; 4 % monosaccharides, 47 % disaccharide, 27 % trisaccharide and 22 % tetrasaccharide; commercial name: Isomalto-900) and fructo-oligosaccharides (FOS; commercial name: Meioligo-P; 44 % 1-kestose, 46 % nystose and 10 % 1 F-β-fructofuranosylnystose) were supplied by Hayashibara Co. Ltd, Nihon Shokuhin Kako Co. Ltd, Showa Sangyo Co. Ltd and Meiji Food Materia Co. Ltd, respectively.
Animals and diets
Male Sprague–Dawley rats were purchased from Japan SLC. Seventy-four rats of 5 weeks of age (mean body weight 130–150 g) were used for the experiments. In addition, eight rats of 8 weeks of age (mean body weight 240–260 g) were used as donors of brush-border membrane vesicles (BBMV) for an in vitro digestion. Rats were housed in individual cages with screen bottoms made of stainless steel and kept in a room maintained at 23 ± 2°C, with 50–70 % humidity, and under 12-h light (07.00–19.00 hours) and 12-h dark conditions. For all experiments, rats were first acclimatised to the experimental settings for 5–9 d and given a basal, 25 % casein diet previously reported(Reference Nishimura, Tanabe and Sasaki19) (online Supplementary Table S1) and water ad libitum.
The present study was approved by the Shizuoka University Animal Use Committee (approval numbers: 29A-15 and 2018A-9). Animals were kept and cared for as per the Guidelines for the Care and Use of Laboratory Animals, Shizuoka University.
Digestibility of isomaltodextrin (Expt 1)
In vivo digestibility of isomaltooligosaccharide and isomaltodextrin
To determine its availability for digestion and absorption, we estimated the in vivo digestibility of IMD in the small intestine of ileorectostomised rats. IMO, which reported to be slowly digestible α-glucans(Reference Kaneko, Yokoyama and Suzuki20), were used as contrasting treatment, due to their average DP of only 2·7 resulting from a low molecular weight, and presence of α-1,4 and 1,6 linkages. Rats were deprived of food, but not water, for 24 h prior to the surgical procedure. After the acclimatisation period ended, rats were ileorectostomised during anaesthetic-induced unconsciousness (inhalation of 2 % isoflurane), as described in our previous report(Reference Nishimura, Nishikawa and Kiriyama21). The surgical procedure was carried out as per the method of Lambert(Reference Nishimura, Nishikawa and Kiriyama21,Reference Lambert22) with some modifications, as follows. As described by Morita et al. (Reference Morita, Ito and Brown23), to shorten the recovery period, the caecum and the colon were not dissected; instead, the ileocaecal valve was ligated. Next, the colonic terminal was anatomised via a stoma in the abdominal wall to allow caecal and colonic contents to be excreted as natural as possible. To prevent post-surgical wound infection, rats were administered an intraperitoneal injection of sulfamethoxazole (about 5 mg; Shinomin, Shionogi). Although they had access to water, rats were not permitted to eat for the first 24-h post-surgery. Following the post-surgical recovery period for 10 d and based on body weight, ileorectostomised rats were divided into basal (control) diet (n 3), 3 % IMO diet (n 5) and 3 % IMD diet (n 5) groups. IMO and IMD supplementation was achieved by replacement of an equal weight of maize starch in the basal diet. Diets were given to rats for 10 d. It was noted that the growth rate of operated rats matched that of pre-operated rats. For determination of undigested, excreted saccharides with DP ≥ 2–3, during the last 3 d of the experimental period, faecal samples were collected, lyophilised, weighed and stored at –80°C until further analysis.
Measurement of isomaltooligosaccharide and isomaltodextrin in diets and faeces
The content of IMO and IMD in diets and faeces was determined by the method of Kondo et al. (Reference Kondo, Handa and Genda24). Briefly, undigested IMO and IMD in the diets and faeces were repeatedly extracted with distilled water and afterwards, defatted with diethyl ether. The IMO and IMD contents in the water phase were measured using an HPLC apparatus equipped with an ULTRON PS-80N column (10 μm, 8·0 × 300 mm, Shimadzu GLC) and a refractive index detector, as the yield of saccharides with DP ≥ 2 (IMO) and DP ≥ 3 (IMD). Spike and recovery testing in duplicate using faecal samples from rats given only the basal diet showed that extraction efficiencies of IMO and IMD were 95·8 and 97·1 %, respectively. These efficiency percentages were used to determine the precise amount of the respective saccharide in faeces.
Calculations
Digestibility of IMO and IMD from mouth to terminal ileum was calculated using data obtained from ileorectostomised rats using the following formulas:
(a) IMO digestibilitymouth-terminal ileum = (intake of saccharides of DP ≥ 2 – faecal saccharides of DP ≥ 2)/intake of saccharides of DP ≥ 2 × 100
(b) IMD digestibilitymouth-terminal ileum = (intake of saccharides of DP ≥ 3 – faecal saccharides of DP ≥ 3)/intake of saccharides of DP ≥ 3 × 100
Digestibility of IMO and IMD was determined by correcting with the data obtained from the control group. For example, saccharides with DP ≥ 2 and 3 were considered indigestible fractions in IMO and IMD, respectively.
In vitro digestion of isomalto-oligosaccharides and isomaltodextrin using brush-border membrane vesicles from rat small intestine
To collect the mucosa of the small intestine, eight rats were given the basal diet for 10 d and then killed by decapitation under general anaesthesia (inhalation of 2 % isoflurane). BBMV were prepared from the mucosa by the method of Kessler et al. (Reference Kessler, Acuto and Storelli25), in which maltase activity was measured by the method of Dahlqvist(Reference Dahlqvist26), and frozen at –80°C until further use. In vitro digestibility of IMO and IMD was determined by our previously reported method(Reference Kondo, Handa and Genda24), which included the AOAC 2001.03 method(Reference Ohkuma, Matsuda and Katta27) and an in vitro digestion method in which porcine pancreatic α-amylase (300 units/0·1 g of saccharides) and BBMV (1000–3000 units/0·1 g of saccharides as maltase activity) from rat small intestine were used(Reference Kondo, Handa and Genda24). In both methods, the resistance to in vitro digestion of IMO and IMD was defined as the yield of sugars after a digestion with a DP ≥ 2 and 3, respectively.
Absorption of glucose released from isomaltodextrin (Expt 2)
In vitro digestion of maize starch, isomaltodextrin and high-amylose maize starch using brush-border membrane vesicles from rat small intestine
To determine the digestion rate of IMD, we examined the time course of in vitro digestion of IMD, comparing maize starch (rapidly digestible) and HAMS (slowly digestible). In vitro digestion method in which porcine pancreatic α-amylase (300 units/0·1 g of saccharides) and BBMV (1000 units/0·1 g of saccharides as maltase activity) from rat small intestine were used. An aliquot of digestion sample was collected at 0, 20, 120, 480 and 960 min for glucose analysis. Glucose concentration in digestion samples was measured with a glucose oxidase method using a commercial kit (Glucose CII-Test Wako, FUJIFILM Wako Pure Chemical). In vitro digestibility of maize starch, IMD and HAMS was determined from the amount of glucose released.
Change in the plasma glucose concentration after oral ingestion of isomaltodextrin
To determine whether IMD is also slowly absorbed in the small intestine after slow digestion, changes in the plasma glucose concentration of rats after oral ingestion were estimated. After an acclimatisation period, twenty-one rats were given the basal diet for 6 d, after which they were deprived of food for 24 h. On day 7 and based on body weight, rats were divided into four groups and given any of saline (n 3; control), glucose solution (n 6), IMD solution (n 6) and HAMS suspension (n 6). Glucose was used as a rapidly absorbed saccharide because it was confirmed that the changes in plasma glucose concentration in rats given glucose and maize starch were similar in our preliminary study (unpublished results). Oral administration was made after blood collection (50 μl; taken as zero time) from the tail vein. A total volume of 4 ml/kg per animal was intra-gastrically administered, with the purpose of achieving a final load of 1 g of saccharide/kg of body weight. Next, blood samples (50 μl) were collected from the tail vein into heparinised microtubes at 15, 30, 45, 60, 90 and 120 min after treatment administration. Blood plasma was separated by centrifugation (1500 g for 20 min at 4°C). The plasma glucose concentration was then measured using a commercial kit (Glucose CII-Test Wako, FUJIFILM Wako Pure Chemical). Control rats were used to identify possible changes in the plasma glucose concentration (e.g. increase in blood glucocorticoids) caused by the experimental procedures (e.g. treatment administration, blood sampling, etc.).
Effect of isomaltodextrin on glucagon-like peptide-1 secretion from the small intestine (Expt 3)
To determine whether IMD, as a slowly glucose-releasing material, stimulates secretion of GLP-1 in the small intestine, we examined the effect of IMD on the portal GLP-1 (active form) concentration in caecectomised rats that were administered antibiotics, comparing with that of FOS, consisting of mainly fructose. Similar to Expt 1, thirty-two rats were deprived of food for 24 h before surgery, although they had access to water ad libitum. After the acclimatisation period, rats underwent experimental and surgical procedures similar to those in Expt 1, except that this time they were caecectomised, as described in our previous report(Reference Nishimura, Nishikawa and Kiriyama21). Again, as in Expt 1, rats were not allowed to ingest food for the first 24 h post-surgery. After recovering from surgery and based on body weight, caecectomised rats were divided into three groups and given any of the basal (n 8, control), 5 % IMD (n 8) and 5 % FOS (n 8) diets for 10 d. IMD and FOS supplementation was achieved by replacement of an equal weight of maize starch in the basal diet. Separately, sham-operated rats (n 8) were given only the basal diet. To stop fermentation in the remaining part of the large intestine, all rats were given water containing 0·2 % (wt/vol) neomycin trisulphate. It was also observed that the growth rate of operated rats matched that of sham-operated rats.
At the end of the experimental period and to laparatomise them, all rats were deprived of food for 3·5 h and anaesthetised by inhalation with 2 % isoflurane. Immediately, 1 ml of blood was collected from the portal vein into microtubes containing heparin (10 units/ml; Ajinomoto, Inc.), aprotinin (500 kallikrein inhibitor units/ml; FUJIFILM Wako Pure Chemical) and DPP-IV inhibitor (50 μmol/ml, Millipore) as per the method of Hira et al. (Reference Hira, Ikee and Kishimoto28). We confirmed that the stomach and small intestine in all rats were full of digesta. Plasma was separated by centrifugation and frozen at –80°C until GLP-1 analysis. After collection of blood, the colon and the caecum and colon were removed from the operated and sham-operated rats, respectively.
Plasma analysis
Plasma GLP-1 concentration was determined using commercial kits (Multi Species GLP-1 Total ELISA kit and Glucagon Like Peptide-1 (Active) ELISA kit, Merck Millipore). Caecal organic acids (acetate, propionate, n-butyrate and succinate) were measured using an HPLC system (LC-10A, Shimadzu) equipped with a Shim-pack SCR-102H column (8 mm internal diameter × 30 cm; Shimadzu) and an electro-conductivity detector, as previously reported(Reference Hoshi, Sakata and Mikuni29).
Statistical analysis
To determine an adequate sample size to identify significant differences in digestibility of saccharides (Expt 1), plasma glucose concentration (Expt 2) and GLP-1 concentration (Expt 3), a power analysis was carried out using the Student’s t test (Expt 1) and one-way ANOVA (Expts 2 and 3) in G*Power statistical package version 3.1.9.3. Sample size was then calculated considering an α probability of 0·05 with a power of 0·80, and the effect size was estimated using the results from a preliminary study at these premises (unpublished results). From the power analysis, it was determined that the required sample size was five (Expt 1), six to seven (Expt 2) and eight (Expt 3) rats per group. Data were analysed for homogeneity of variances with Bartlett’s test. Data with unequal variances were log-transformed, and for those with equal variances, one-way ANOVA was used, followed by Student’s t test (Expt 1) and the Tukey–Kramer post hoc test (Expts 2 and 3), to compare individual group means. If sample variances were still unequal after log-transformation, Welch’s t test (Expt 1) and the Steel–Dwass test (Expts 2 and 3) were then used. Apart from the power analysis, all statistical analyses were carried out using SAS JMP software (version 13.2.1). Values obtained from the experiments are expressed as mean values with their standard errors, and the statistical significance was defined as P < 0·05.
Results
Digestibility of isomaltodextrin (Expt 1)
Food intake and body weight gain did not differ between experimental groups (data not shown). Whilst IMO was almost completely digested in the small intestine of rats, the digestibility of IMD in vivo was approximately 80 % (Table 1). However, the in vitro digestibility of IMO and IMD was lower than that in vivo (online Supplementary Fig. S1). To test whether the digestibility of IMD but not IMO in vitro could be further increased, we increased the units of BBMV in the reaction system to 2000 and 3000. Interestingly, even after increasing the BBMV in the reaction system, the digestibility of IMO and IMD remained almost constant.
Table 1. Food intake and body weight gain of ileorectostomised rats and their digestibility of isomaltooligosaccharides (IMO) and isomaltodextrin (IMD)†
(Mean values with their standard errors)

RG, digestion-resistant α-glucan.
* Means are significantly different (P < 0·05) from those of group IMO.
† Ileorectostomised rats were given for the first 10 d only a basal diet (control) or the basal diet supplemented with 30 g/kg of either IMO or IMD. RG intake was calculated using the data from the estimated food intake and the measured values of digestion-resistant α-glucan in diets.
Changes in the plasma glucose concentration after oral ingestion of isomaltodextrin (Expt 2)
In in vitro digestion, timewise and based on the maltase activity of 1000 units of BBMV, the fractions of maize starch, IMD and HAMS digested during the first 2 h were 64, 43 and 29 %, respectively (online Supplementary Fig. S2 and Table S2).
As in Expt 1, food intake and body weight gain did not differ between the experimental groups (data not shown). The concentration of glucose in plasma increased after administration of every saccharide. At 15 min post-administration and compared with that in the glucose group, the concentration of glucose in plasma was significantly lower (P < 0·05) in the HAMS group but tended to be lower in the IMD group (P = 0·0652). Peak levels of glucose were observed at 30 min post-administration of glucose. By contrast, the concentration of plasma glucose after administration of IMD and HAMS peaked between 30 and 45 min (Fig. 1).
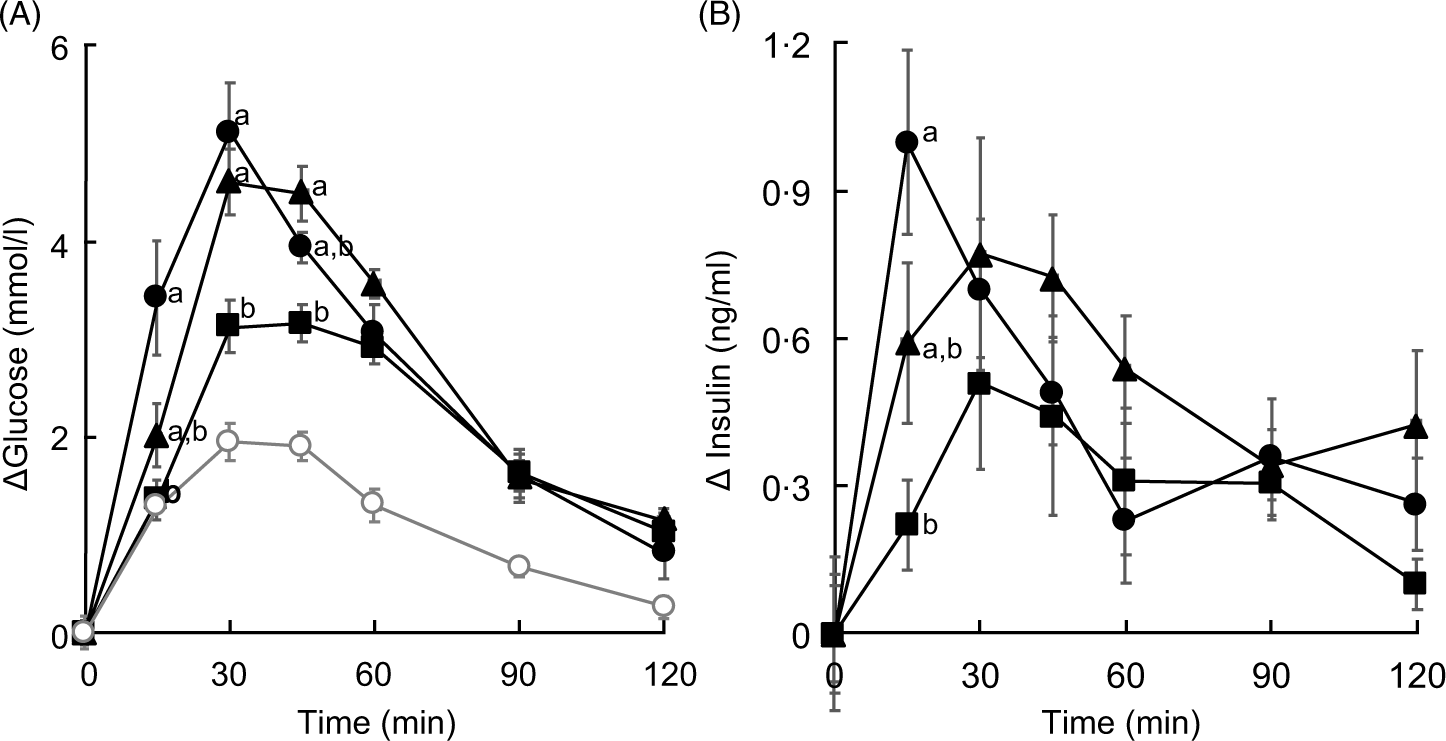
Fig. 1. Changes in the concentrations of plasma glucose and insulin in isomaltodextrin (IMD)-administered rats. (A) Plasma glucose and (B) plasma insulin. Treatment groups were administered 1000 mg of saccharide/kg of body weight after fasting for 24 h. Values are means with their standard errors represented by vertical bars (n 6, except saline group, n 3). Repeated-measures, two-factor ANOVA was used to analyse the concentrations of Δplasma glucose and insulin in all experimental groups except for the saline group, across time (Δplasma glucose: saccharide, P = 0·0050; time, P < 0·0001; interaction, P < 0·0001; Δplasma insulin: saccharide, P = 0·1972; time, P < 0·0001; interaction, P = 0·0085), followed by the Tukey–Kramer post hoc test. a,b Mean values with unlike letters at specific time points are statistically significantly different (P < 0·05). (A) , Group administered glucose;
, group administered IMD;
, group administered high-amylose maize starch (HAMS);
, group administered saline. (B)
, Group administered glucose;
, group administered IMD;
, group administered HAMS.
The area of the plasma glucose concentration under the curve for 0–30 min (AUC0–30 min) was significantly lower in the HAMS group than that in the glucose group. Although the AUC of the IMD group was found to be 70 % that of the glucose group, it was not statistically significant. Following a similar trend, the AUC for 0–120 min (AUC0–120 min) was significantly lower (P < 0·05) in the HAMS group but not the IMD group than that in the glucose group (Table 2). The AUC30–120 min showed no significant differences between the experimental groups (data not shown). Lastly, when compared with that of the glucose group, the AUC0–30 min:AUC0–120 min ratio was significantly lower (P < 0·05) in the HAMS group and tended to be lower (P = 0·0909) in the IMD group.
Table 2. Effect of isomaltodextrin (IMD) on glucose absorption*
(Mean values with their standard errors)

HAMS, high-amylose maize starch.
a,b Mean values within a row with unlike superscript letters were significantly different (P < 0·05).
* Data were analysed with one-way ANOVA and the Tukey–Kramer post hoc test.
† Percentages of AUC from the respective group to the control group.
Effect of isomaltodextrin on glucagon-like peptide-1 secretion from the small intestine (Expt 3)
As in Expts 1 and 2, no differences in food intake and body weight gain were detected between the experimental groups. In the caecum of sham-operated rats given the basal diet, although n-butyrate was undetected, the concentrations of acetate and propionate were 6·67 (se 0·66) and 3·34 (se 0·29) μmol/g, respectively. Nonetheless, in antibiotic-administered, caecectomised rats, SCFA were barely detected in the colon (Table 3), unlike in sham-operated rats.
Table 3. Food intake, body weight gain and colonic organic acid concentration of caecectomised rats administered 0·2 % neomycin sulphate and either isomaltodextrin (IMD) or fructo-oligosaccharides (FOS)†
(Mean values with their standard errors; median values and ranges (Min–Max))
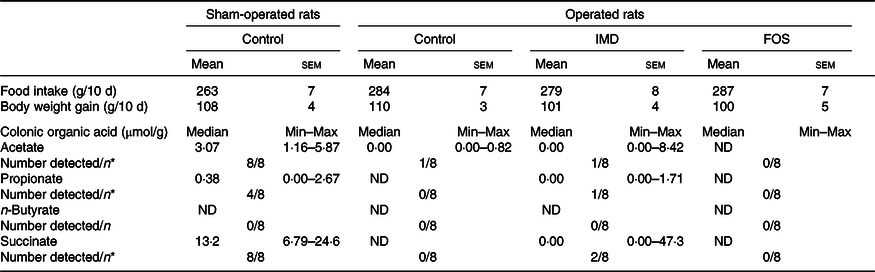
ND, not detected.
a,b Mean values within a row with unlike superscript letters were significantly different (P < 0·05).
* Number of rats in which the respective organic acid was detected; the resulting data were analysed with Fisher’s exact test. Acetate, P < 0·0001; propionate, P = 0·034; succinate, P < 0·0001.
† Sham-operated rats underwent a surgical procedure similar to caecectomy except that the caecum was not removed; operated rats were caecectomised. The control group was given a basal diet. The FOS group was given the basal diet supplemented with 5 % FOS. The IMD group was given the basal diet supplemented with 5 % IMD.
The total concentration of GLP-1 in portal plasma did not significantly differ between experimental groups. In contrast, the concentration of the active form of GLP-1 was significantly lower in the caecectomised rats given the basal diet than in sham-operated rats given the same diet. Finally, when compared with that of control, caecectomised rats and unlike FOS, IMD significantly improved the portal concentration of the active form of GLP-1 (Fig. 2).
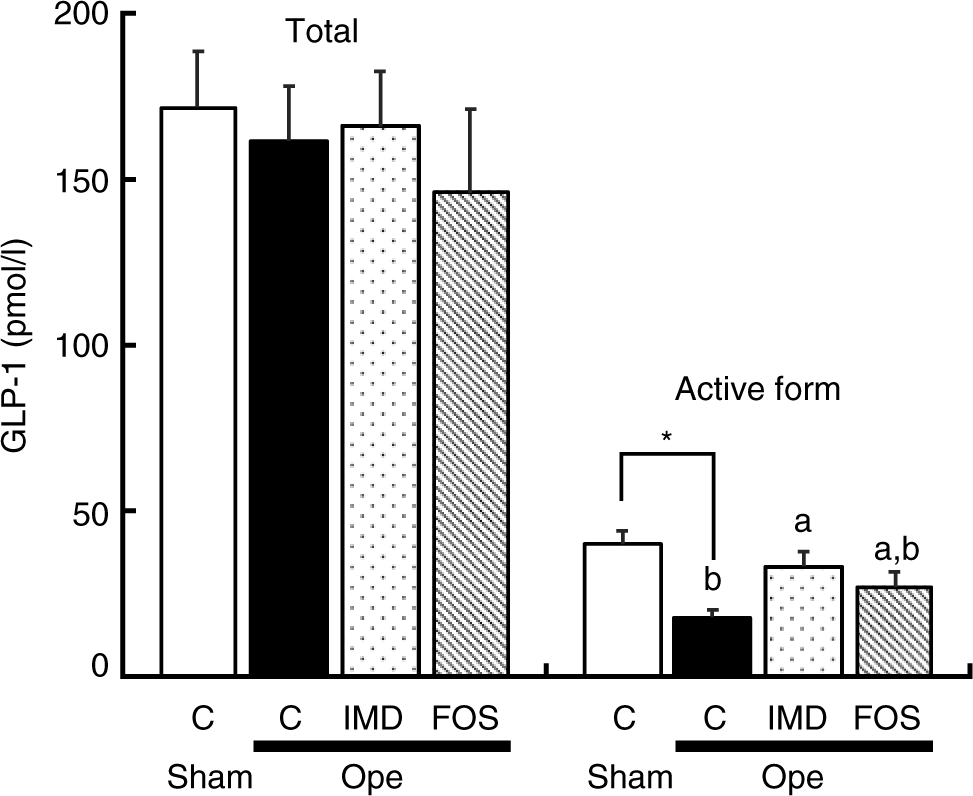
Fig. 2. Effect of isomaltodextrin (IMD) on glucagon-like peptide-1 (GLP-1) concentration in portal plasma of caecectomised rats. C, group given the basal diet; FOS, group given the basal diet supplemented with 5 % fructo-oligosaccharide; IMD, group given the basal diet supplemented with 5 % IMD; Ope, caecectomised rats; Sham, sham-operated rats. All rats were given drinking water supplemented with 0·2 % neomycin sulphate. Values are means with their standard errors represented by vertical bars (n 8). a,b Unlike letters indicate that mean values of rat groups are significantly different, except for sham-operated rats (P < 0·05). * Mean values are significantly different between Sham and Ope groups (P < 0·05). The data were analysed with one-way ANOVA and Student’s t test. The data from the three rat groups undergoing caecectomy were analysed with one-way ANOVA and the Tukey–Kramer post hoc test.
Discussion
IMD, which is produced from starch using enzymes α-glucosyl transferase and α-amylase derived from Paenibacillus alginolyticus PP710, is a highly branched dextrin with α-glucosidic linkages (3 % α-1,3 linkages, 19 % α-1,4 linkages, 49 % α-1,6 linkages, 7 % α-1,3,6 linkages and 5 % α-1,4,6 linkages; average DP 30)(Reference Tsusaki, Watanabe and Nishimoto13). In the present study, the digestibility of IMD in the small intestine was estimated to be 70–80 % both in vivo and in vitro. Contrastingly, IMO, consisting of 81·5 % of saccharides, with a DP of 2–5 and containing α-1,4 and α-1,6 but not α-1,3 linkages, was 96–99 % digestible, which indicated that α-1,3 linkages and DP levels were likely factors critically determining the digestibility of α-glucan in the small intestine of rats. Now, considering the analysis of the concentration of plasma glucose after treatment administration to rats, our results showed that the AUC0–30 min for IMD and HAMS were 72 and 49 %, respectively, that for the glucose group (Table 2), suggesting that the absorption of glucose released from IMD and HAMS would delay due to their slow digestibility. Although AUC0–30 min and the percentage of digested fraction after 2 h may not be comparable, it was very interesting to observe that these percentage differences were very similar to those resulting from the analysis of the digested fraction of starch, IMD and HAMS after 2 h (IMD, 67 % and HAMS, 45 % that of starch (63·9 %)) (online Supplementary Table S2). These data seem to support that, since IMD is partially resistant to digestion and hence broken down at a slower rate, digestion of IMD may extend to the distal small intestine. However, in the present study, we did not observe direct evidence of increased glucose released from IMD to the ileum because the assay for ileal glucose was not carried out. Further investigation needs to be performed to elucidate whether IMD delivers glucose to the ileum.
In the present study, differences in digestibility were observed when IMD was assessed in vitro and in vivo, being the digestibility of IMO and IMD lower in vitro than in vivo, which was in total agreement with the work on resistant maltodextrin by Kondo et al. (Reference Kondo, Handa and Genda24). The differences in digestibility may be explained by the fact that the orocaecal transit time of IMD in ileorectostomised rats was longer (about 9 h) than that reported in entire rats (about 7 h)(Reference Kondo, Handa and Genda24), which likely lengthened the time required to digest IMD. Moreover, we also observed the moderately increased concentration of plasma glucose in rats given saline. Blood was carefully collected not to load to rats in the present study because blood sampling from rats enhances the secretion of glucocorticoids, which increase blood glucose concentration by inducing hepatic gluconeogenesis. Although the increase in glucocorticoid concentration in the blood is less when blood is collected from the tail vein than another blood sampling, moderately increased secretion of the hormones is observed in rodents after 30 min of blood sampling(Reference Kim, Foong and Cooper30). Therefore, the increased glucose concentration in plasma in rats given saline would attribute to the experimental procedures such as blood sampling.
GLP-1 is produced by L cells densely found in the ileum and the large intestine, and its secretion is further stimulated if nutrients such as glucose (released from food) in the ileum and SCFA (via fermentation) in the large intestine are fully available. In the present study, the concentration of portal active GLP-1 in antibiotic-administered, caecectomised rats significantly increased by feeding IMD, but not FOS, predominantly consisting of fructose moiety. As the caecum is the main fermentation organ in rats and mice, as expected, SCFA were not detected in antibiotic-administered, caecectomised rats even after being given IMD and FOS diets. In light of this, it is likely that an elevated concentration of portal active GLP-1 was induced by glucose released from IMD in the small intestine, perhaps the ileum. Indeed, Hira et al. (Reference Hira, Ikee and Kishimoto28) previously reported that resistant maltodextrin, an α-glucan partially consisting of β-glycosidic linkages, stimulated GLP-1 secretion. These workers concluded that direct (by resistant maltodextrin itself) and indirect (by SCFA produced by colonic bacteria) effects on the L cells in the ileum and large intestine, respectively, caused an elevated production and secretion of GLP-1 after administration of resistant maltodextrin. As it has been assumed that resistant maltodextrin is barely broken down in the gut(Reference Gordon and Okuma31), the effect of glucose released from the glucan on GLP-1 secretion in the ileum may seem unusual. However, previous work has reported that glucans constituted by many α-linkages were partially digested in the small intestine(Reference Kondo, Handa and Genda24). These workers also reported that the digestibility of resistant maltodextrin was approximately 30 %(Reference Kondo, Handa and Genda24). Therefore, it can be reasonably speculated that the reported increase in GLP-1 secretion due to administration of resistant maltodextrin could also have been dependent on glucose delivery, as it was the case for IMD in the present study. However, it remains unclear whether an undigested fraction of slowly digestible α-glucan such as IMD and resistant maltodextrin would stimulate GLP-1 secretion. Also, in the present study, we did not examine the time course of portal GLP-1 concentration by a single oral gavage of IMD. Further study is required to elucidate the effect of the undigested fraction of α-glucan on GLP-1 secretion and the increase in GLP-1 secretion from l-cells in the ileum by IMD glucose.
In addition, some limitations of the study need to be considered. GLP-1 secretion could be altered by the duration of fasting and the amount of nutrient intake. Although there was no difference in food intake for 24 h before 3·5 h fasting between groups given the control and IMD diet in the present study (data not shown), the difference in the timing rats consumed the diet between both groups cannot be excluded. Also, the administration of IMD for 10 d may also increase basal GLP-1 secretion. These limitations should be acknowledged to interpret the present study.
Many α-glucans have been artificially developed and marketed as a nutraceutical material with low-energy and high dietary fibre contents. However, as shown in the present study and elsewhere(Reference Kondo, Handa and Genda24), these commercially available α-glucans can be digested to some extent, at least in the small intestine of rats. Hence, in the present study, the true content of dietary fibre in these α-glucans has been shown to be lower. For example, although the content of dietary fibre in IMD is approximately 80 %(Reference Tsusaki, Watanabe and Yamamoto32) as per the AOAC 2001.03 method(Reference Ohkuma, Matsuda and Katta27), in the present work the true content of dietary fibre in IMD after digestion was found to be 20 % in vivo (ileorectostomised rats) and 30 % in vitro (α-amylase and BBMV). Since digestion-resistant α-glucans were found to be slowly digested, more α-glucan may potentially be available for digestion in the small intestine, perhaps the ileum and, as a result, more glucose may be potentially released. More readily available glucose would stimulate GLP-1 secretion from L cells in the ileum. This may well be a new property of α-glucans, which were first categorised as slow-release or ‘lente’ carbohydrates by Jenkins et al. (Reference Jenkins, Wolever and Taylor33). Furthermore, as far as humans are concerned, the delivery of slowly digestible α-glucans such as IMD to the ileum could potentially play an important role in the improvement of health.
It must be stressed that the use of ileorectostomised and caecectomised rats in the present work to estimate the digestibility of IMD and to examine its effect on GLP-1 secretion in the ileum permitted us to obtain detailed information and understanding of the effect of nutrients on those specific parts of the gut. However, caution should be taken if these surgical procedures are to be conducted again, as they affect certain physiological processes in rats, for example, altered transit time caused by diarrhoea occurrence, lack of caecum, and stronger than normal intestinal adhesion. Although it was confirmed that no pathological adhesion took place after the surgical procedures, occurrence of diarrhoea and absence of caecum were unavoidable events.
In conclusion, IMD, a highly branched and partially resistant to digestion (70–80 % digestibility) α-glucan, was shown to be slowly digested and absorbed in the small intestine of rats. These results may show that, unlike that of maize starch, digestion of IMD extends to the distal small intestine. In addition, we showed that glucose of IMD released in the small intestine, speculatively the ileum, stimulated GLP-1 secretion. Delivery of glucose to the small intestine could potentially exert significant, yet unknown physiological effects on glucose homoeostasis of recipients. Although both humans and rats are omnivores, the gastrointestinal tract has some anatomical and physiological differences between rats and humans. The differences could affect the digestibility and absorbability of nutrients. Although the time for digesta to traverse the small intestine of rats and humans is almost the same, the surface area to the length of the small intestine is smaller in rats than that in the human(Reference DeSesso and Jacobson34). Therefore, IMD may be more rapidly digested in and absorbed from the small intestine in humans than in rats. Whether the present findings can be extrapolated to humans requires further investigation.
Acknowledgements
The authors thank editors at BioScience Proofreaders (Tokyo, Japan) for their assistance in the manuscript editing.
N. N. designed the research; Y. K., T. K., S. H. and T. M. conducted the research; N. N., Y. K. and T. K. analysed the data; N. N. wrote the manuscript and was the primary responsible for the final content. All authors were involved in designing the study, reviewing and interpreting the results, and drafting the manuscript. In addition, all authors read and approved the final document.
The authors declare that there are no conflicts of interest.
Supplementary material
For supplementary materials referred to in this article, please visit https://doi.org/10.1017/S0007114519003210