Introduction
Mount Cervandone, in the Devero Valley, and the Swiss Wannigletscher (also named Cherbadung) are the type localities of rare earth element (REE) arsenates and arsenites including asbecasite (Graeser, Reference Graeser1966), cafarsite (Graeser, Reference Graeser1966), cervandonite-(Ce) (Armbruster et al., Reference Armbruster, Bühler, Graeser, Stalder and Amthauer1988), fetiasite (Graeser et al., Reference Graeser, Schwander, Demartin, Gramaccioli, Pilati and Reusser1994), gasparite-(Ce) (Graeser and Schwander, Reference Graeser and Schwander1987) and paraniite-(Y) (Demartin et al., Reference Demartin, Gramaccioli and Pilati1994). Mount Cervandone is also the type locality of deveroite-(Ce), a natural REE-oxalate (Guastoni et al., Reference Guastoni, Nestola, Gentile, Zorzi, Alvaro, Lanza, Peruzzo, Schiazza and Casati2013). Marchettiite (Fig. 1) is the eighth type mineral and the seventh organic mineral found in the alpine fissures of Mount Cervandone and Wannigletscher after deveroite-(Ce) (Guastoni et al., Reference Guastoni, Nestola, Gentile, Zorzi, Alvaro, Lanza, Peruzzo, Schiazza and Casati2013), humboldtine (Guastoni et al., Reference Guastoni, Gentile, Nestola, Soldani and Zorzi2015), lindbergite (Albertini and Meisser, Reference Albertini and Meisser2012), moolooite (Guastoni, Reference Guastoni2009), tinnunculite (Guastoni et al., Reference Guastoni, Nestola and Zorzi2020) and whewellite (Guastoni et al., Reference Guastoni, Gentile, Nestola, Soldani and Zorzi2015).

Fig. 1. Optical microphotograph of marchettiite holotype (Museum of Mineralogy of the Department of Geosciences at the University of Padova, catalogue number MMP M171892), the long side of the picture is 6 mm (photo: Stefano Castelli).
In this study, we describe a new mineral named marchettiite, ideally C5H7N5O3, found at Mount Cervandone, Devero valley, Western-Central Alps, Italy. Marchettiite is an organic compound collected in 1995 in an alpine cleft by Gianfranco Marchetti, a local mineral collector. The mineral is stable in natural atmospheric conditions and at room temperature.
Marchettiite has no natural analogue, powder micro-X-ray diffraction and electron microprobe analysis highlighted that is the natural analogue of the synthetic ammonium hydrogen urate compound (Friedel et al., Reference Friedel, Bergmann, Kleeberg and Schubert2006; Sekkoum et al., Reference Sekkoum, Cheriti, Taleb and Belboukhari2016); also known as the anhydrous ammonium salt of uric acid. The simple molecular formula C5H7N5O3 can be written alternatively as (NH4)C5H3N4O3 (Tettenhorst and Gerkin, Reference Tettenhorst and Gerkin1999).
The new mineral and the name (symbol Mht) have been approved by the Commission on New Minerals, Nomenclature and Classification (CNMNC) of the International Mineralogical Association (IMA2017-066, Guastoni et al., Reference Guastoni, Nestola, Gentile, Zorzi, Andò, Lorenzetti and Mattioli2017). The holotype material is deposited in the mineralogical collection of the Museum of Mineralogy of the Department of Geosciences at the University of Padova (Italy), under the catalogue number MMP M171892. The name is for Gianfranco Marchetti (1943–2013), discoverer of the mineral and amateur mineralogist.
Occurrence
Mount Cervandone is composed of fine-grained to very fine-grained two-mica leucocratic gneisses belonging to the lower-Penninic, Mount Leone-Arbola’ nappe (Dal Piaz, Reference Piaz G.1975; Steck, Reference Steck2008). The Mount Leone nappe emplacement took place in a regime of ductile deformation and contains 3T-polytype phengite relicts overprinted by the regional amphibolite-facies metamorphism (Hammerschmidt and Frank, Reference Hammerschmidt and Frank1991). In this area, REE bearing-minerals include several arsenites, arsenates, phosphates, oxides and deveroite-(Ce), a natural REE-oxalate (Guastoni et al., Reference Guastoni, Pezzotta and Vignola2006; Guastoni et al., Reference Guastoni, Cámara and Nestola2010; Guastoni et al., Reference Guastoni, Nestola, Gentile, Zorzi, Alvaro, Lanza, Peruzzo, Schiazza and Casati2013). REE-bearing minerals, with the exception of the organic compound deveroite-(Ce), are related to the presence of swarms of sub-horizontal dykes with pegmatitic textures, which crop out for hundreds of metres along the slopes of Mount Cervandone and Punta Marani. These dykes were involved with regional amphibolite-facies metamorphism and underwent ductile deformation developing folds or filling gaps related to foliation boudinage of the main schistosity. These dykes are composed of coarse grained vitreous colourless or smoky quartz, K-feldspar and silvery-greenish micas. Pegmatite dykes also contain accessory minerals including centimetric up to decimetric black tourmaline prisms and curved mica flakes embedded in vitreous ‘smoky’ quartz which exhibit boudinage-like textures. Occasionally, centimetric pale sky-blue beryl prismatic crystals were also found. Pegmatitic dykes are locally cut by Alpine-type fissures which contain a rich assemblage of typical Mount Cervandone minerals as reported above.
The formation of marchettiite requires the presence of water as the fluid medium. This natural anhydrous ammonium hydrogen urate is not related to any amphibolite-facies metamorphism or pegmatite dykes but was formed by circulation of meteoric waters at low temperatures enriched with soluble altered biological material (Hazen et al., Reference Hazen, Downs, Kah, Sverjensky, Hazen, Jones and Baross2013).
Experimental methods
Electron microprobe analysis
Semi-quantitative electron microprobe analyses (EMPA) were performed on the same crystal fragment used for the micro-X-ray diffraction analysis at the Dipartimento di Scienze Geologiche e Geotecnologie of the University of Milano-Bicocca, Italy. The chemical composition was determined using a SEM Tescan VEGA TS 5136 XM equipped with energy-dispersive analysis (EDS) by means of an EDAX GENESIS 4000 XMS electron microprobe. Major and minor elements were determined at 20 kV accelerating voltage and 190 ρA (in a Faraday cup), spot size = 250 nm, 100 s as counting time and working distance = 23 mm. For EDS semi-quantitative analyses the following lines were used: CKα, NKα. C and N were measured (in wt.%) on a crystal fragment that had been polished and Au coated using an Edwards Sputter Coater S150B, under an Argon atmosphere at 6.5 mbar, operating at 40 mA/1 kV, for 10 s in order to obtain an estimated 2.5 nm of Au coating.
The mean analytical results are given (Table 1). The empirical formula, based on 3O atoms per formula unit (apfu) obtained from semi-quantitative EMPA (with H2O and C2O3 calculated by stoichiometry, due to the very small quantity of material available) is: C4.99H6.97N4.91O3.00. The ideal formula is C5H7N5O3, or alternatively written as (NH4)C5H3N4O3, which requires: C 32.44, H 3.81, N 37.83, O 25.92, total 100.00 wt.%.
Table 1. Semi-quantitative chemical data (by wt.%) for marchettiite (n = 15).

Notes: *H, *O, calculated by stoichiometry (Friedel et al., Reference Friedel, Bergmann, Kleeberg and Schubert2006)
Optical properties
The refractive indices were computed from the crystal structure using the periodic coupled–perturbed Kohn Sham method as implemented in CRYSTAL17 (Dovesi et al., Reference Dovesi, Erba, Orlando, Zicovich-Wilson, Civalleri, Maschio, Rerat, Casassa, Baima, Salustro and Kirtman2018; Ferrero et al., Reference Ferrero, Rérat, Orlando and Dovesi2008) using the B3LYP/pob-TZVP_rev2 (Vilela Oliveira et al., Reference Vilela Oliveira, Laun, Peintinger and Bredow2019) level of theory with the shrinking factor set to 8.
X-ray diffraction
Powder micro X-ray diffraction (PXRD) data were recorded on a Rigaku-Oxford Diffraction Supernova diffractometer equipped with a micro X-ray source (beam diameter = 0.12 mm and λ = MoKα) and a 200K Pilatus detector (Dectris). An aggregate of ~0.100 mm was glued on a borosilicate fibre. The sample-to-detector distance was 68 mm. The experimental PXRD profile was extracted with Crysalis Pro (Rigaku Oxford Diffraction), and further analysed with the programs Highscore Plus (Panalytical) and GSAS 2 (Toby and Von Dreele, Reference Toby and Von Dreele2013). Although the small crystal sizes did not allow us to refine structural data, it was possible to obtain unit-cell parameters, which are in good agreement (Table 2) with those reported for synthetic anhydrous ammonium acid urate (Friedel et al., Reference Friedel, Bergmann, Kleeberg and Schubert2006) and to perform a qualitative validation of the structural model.
Table 2. Unit-cell parameters from X-ray diffraction data for marchettiite in comparison with the synthetic analogue.

Table 2. nafeasite. Only calculated lines with intensities > 1.5 are listed.
The limited data quality did not allow for ab initio structure solution. Nevertheless, starting from the structural model proposed by Friedel et al. (Reference Friedel, Bergmann, Kleeberg and Schubert2006), we have performed a rigid-body Rietveld refinement which converged to a meaningful structure. Our refined structure differs from the published one for the absence of any excess NH3 in our structural model, in agreement with the chemical analysis. A second difference is in the intramolecular geometry, as we have maintained the planarity expected for the flat and rigid monodeprotonated urate anion. Though the fitting of the experimental pattern is not ideal, and the model cannot be reliably refined further without overparameterising, the fair agreement between observed and calculated pattern (Fig. 2) is further evidence supporting the identification of marchettiite as the natural analogue of ammonium hydrogen urate. Observed d values and intensities are given in Table 3, atomic coordinates in Table 4 and bond distances in Table 5. The crystallographic information file has been deposited with the Principal Editor of Mineralogical Magazine and is available as Supplementary material (see below). The crystal structure of marchettiite is showed in Fig. 3.

Fig. 2. Rigid-body Rietveld refinement of marchettiite against PXRD data (λ = 0.71073 Å; Rp = 0.032; Rwp = 0.045; RBragg = 0.130; RF2 = 0.148).

Fig. 3. The structure of marchettiite (colour code: C grey, N blue, O red and H white). (a) hydrogen urate anion and ammonium cation with atom labelling scheme. (b) The approximative distances between hydrogen bond donors and acceptors in the structure are indicated in green. (c) The hydrogen bonds (in blue) form a 2D network. (d) The hydrogen-bonded planes (distinguished with different colours) stack in the third dimension via π⋅⋅⋅π interactions (in magenta) among the anions. The structure is plotted using Vesta 3 software (Momma and Izumi, Reference Momma and Izumi2011).
Table 3. Powder X-ray diffraction data (d in Å) for marchettiite.*

*The strongest lines are given in bold
Table 4. Fractional atomic coordinates and isotropic displacement parameters (Å2) for marchettiite. Hydrogen urate and ammonium cations were treated as rigid bodies, hence standard uncertainties are reported only for the reference points and not for the individual atomic coordinates. For hydrogen urate, the geometrical centre of the 6-membered ring, indicated as point ‘A’, was used as the reference point. For ammonium, N10 was used as reference point. A global isotropic displacement parameter was refined for each rigid body.

Table 5. Interatomic distances (Å) for marchettiite. The values reflect the unrefined intramolecular distances of the rigid bodies.

Micro-Raman spectroscopy
The micro-Raman spectrum of marchettiite was collected at the Department of Chemical Sciences of the University of Padova using a Raman DXR (ThermoScientific) with a laser wavelength of 532 cm–1 (power 8 mW). The spectrum was collected in the range of 200–3500 cm–1 with a spectral resolution of 1.9 cm–1 and a spatial resolution of 1 μm. The Raman spectrum is shown in Fig. 4a (which also reports the Raman band values) and compared with the synthetic commercial anhydrous ammonium hydrogen urate (Santos et al., Reference Santos, Carcía-Ramos, Bellanato, Carmona, Cifuentes-Delatte, Vahlensieck and Gasser1984) in Fig. 4b.

Fig. 4. Raman spectrum of marchettiite (a) and comparison with the synthetic analogue by Santos et al. (Reference Santos, Carcía-Ramos, Bellanato, Carmona, Cifuentes-Delatte, Vahlensieck and Gasser1984) in (b).
Infrared spectroscopy
The Fourier-transform infrared (FTIR) spectrum was acquired at the Department of Ingegneria Industriale of the University of Padova by FTIR-ATR technique using a Nicolet iS50 FTIR (Thermo Fisher Scientific) equipped with diamond crystal. The spectrum was collected in the range of 4000–650 cm–1 with a resolution of 4 cm–1. The FTIR spectrum with peak values of marchettiite is given in Fig. 5; literature data are summarised in Table 6 (Benramdane et al., Reference Benramdane, Bouatia, Idrissi and Draoui2008 and Sekkoum et al., Reference Sekkoum, Cheriti, Taleb and Belboukhari2016). From the FTIR results, it is evident that our sample of marchettiite is not pure material but is probably intermixed with whewellite.

Fig. 5. Infrared spectrum of marchettiite.
Table 6. FTIR data for marchettiite in comparison with urate hydrogen ammonium from two sources. Bold notes the bands common to all three samples.
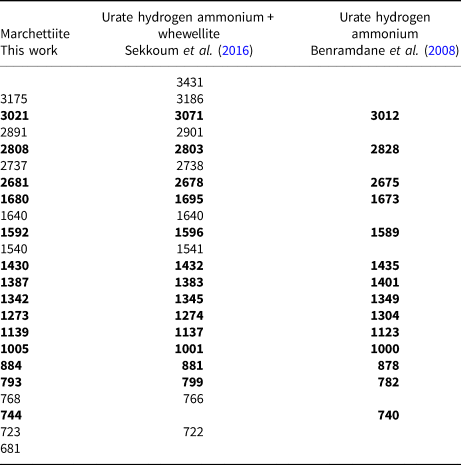
Results and discussion
Physical and optical properties
Marchettiite occurs as aggregates of opaque pale pink to white, elongated, platy, prismatic crystals (the actual morphology can be determined only by SEM). Indeed, marchettiite shows no monocrystals (as demonstrated by X-ray diffraction) and is always found in microcrystalline aggregates in a cleft of fine grained orthogneiss. Marchettiite shows no inclusions of, or evident intergrowths with, other minerals. The maximum size of the marchettiite aggregates is 3 mm (Figs 1 and 6). Marchettiite is associated with albite, K-feldspar, muscovite, quartz and whewellite. It has a white streak, a dull and opaque lustre, is not fluorescent, and neither pleochroism nor twinning were observed. Due to the extremely limited thickness of the crystal hardness is only estimated as 2–2½ on the Moh scale. Attempts of micro-indentation on a very thin crystal only provided unreliable data. The tenacity is brittle and the crystals have a good cleavage, parallel to {001}. Due to the small grain size, it was impossible to measure the density. The calculated density using the empirical formula and our unit-cell data is equal to 1.69 g/cm3, in good agreement with the synthetic analogue, which is 1.772 g/cm3 (Tettenhorst and Gerkin, Reference Tettenhorst and Gerkin1999).

Fig. 6. Back-scatter electron image of marchettiite (~850 μm wide). Holotype specimen, catalogue number MMP M171892.
Optical properties were determined by using the periodic – DFT method (details are reported in the Experimental methods below). Marchettiite is biaxial (–), with α = 1.372, β = 1.681, γ = 1.768 and 2V = 47.243°. This relatively new approach for minerals was adopted as we realised that, at least at the scale of the FTIR analyses (see sections below), it seemed evident that marchettiite was intermixed with a probable whewellite impurity and, at the same time, considering the polycrystalline nature of marchettiite it was actually impossible to obtain any reliable optical properties experimentally.
The refractive indices of marchettiite are in satisfying agreement with those of tinnunculite (C5H4N4O3⋅2H2O), of: α = 1.503(3), β = 1.712(3), γ = 1.740(1), biaxial (–) and 2Vobs = 40(10)° (Pekov et al., Reference Pekov, Chukanov, Yapaskurt, Belakovskiy, Lykova, Zubkova, Shcherbakova, Britvin and Chervonnyi2017) and its synthetic counterpart, uric acid dihydrate with: α = 1.508(3), β = 1.691(3), γ = 1.728(3), biaxial (–) and 2Vobs = 40.4°(6) (Ringertz, Reference Ringertz1965).
Marchettiite has no natural non-biological analogue, as mentioned above, however there is a synthetic analogue (Friedel et al., Reference Friedel, Bergmann, Kleeberg and Schubert2006; Sekkoum et al., Reference Sekkoum, Cheriti, Taleb and Belboukhari2016). Other related natural organic species approved by the IMA–CNMNC include: acetamide, CH3CONH2 (Srebrodol'skiy, Reference Srebrodol'skiy1975); guanine, C5H3(NH2)N4O (Bridge, Reference Bridge1974); kladnoite, C6H4(CO)2NH (Jehlička et al., Reference Jehlička, Žáček, Edwards, Shcherbakova and Moroz2007); tinnunculite, C5H4N4O3⋅2H2O (Pekov et al., Reference Pekov, Chukanov, Yapaskurt, Belakovskiy, Lykova, Zubkova, Shcherbakova, Britvin and Chervonnyi2017); urea, CO(NH2)2 (Bridge, Reference Bridge1973) and uricite, C5H4N4O3 (Bridge, Reference Bridge1974).
X-ray diffraction and marchettiite crystal structure
As noted above, the mineral marchettiite is the natural equivalent of the compound ammonium hydrogen urate, also known as the ammonium salt of uric acid (Sekkoum et al., Reference Sekkoum, Cheriti, Taleb and Belboukhari2016).
The space group of marchettiite, in analogy with its synthetic counterpart, is P $\bar{1}$ and the unit-cell parameters refined by PXRD are: a = 3.6533(2) Å, b = 10.2046(7) Å, c = 10.5837(7) Å, α = 113.809(5)°, β = 91.313(8)°, γ = 92.44(1)°, V = 360.312 Å3 and Z = 2. The list of d spacings obtained by PXRD for marchettiite from the Le Bail refinement are reported in Table 3. The synthetic counterpart of marchettiite by Friedel et al. (Reference Friedel, Bergmann, Kleeberg and Schubert2006) has the unit-cell parameters: a = 3.650 Å, b = 10.215 Å, c = 10.597 Å, α = 113.9°, β = 91.1°, γ = 92.3° and V = 360.8 Å3. The natural and synthetic compounds show an excellent agreement in all single-cell parameters and volume and this definitively supports the assumption that they have a nearly identical composition.
The crystal structure is composed by the flat and rigid hydrogen urate anions and ammonium cations (Fig. 4a), and features two ionic pairs per unit cell, related by the crystallographic inversion centre. Though the limited precision of the model does not allow a detailed discussion of the interactions, we can infer that hydrogen urate anions can interact side-by-side via pairs of hydrogen bonds on each side, thus forming H-bonded ribbons. Ammonium cations occupy interstitial sites between neighbouring ribbons and are within favourable distances (≤ 3.0 Å) from the oxygen acceptor atoms (O2, O6 and O8) of four different anions (Fig. 4b). Due to ammonium's tetrahedral geometry, it cannot interact with all four acceptors simultaneously and it is probably disordered around its average position (we could not experimentally locate the positions of hydrogen atoms). All in all, the possible hydrogen bonds give rise to a waved 2D network (Fig. 4c). The crystal packing is completed by a parallel stacking of hydrogen urate moieties, into columns extending along the crystallographic a axis (Fig. 4d). The average stacking distance of 3.340(2) Å is indicative of a π⋅⋅⋅π interaction among the anions.
Because of the abundance of H-bond donors and acceptors available (3 donors and 4 acceptors on acid urate; 4 donors on ammonium) and the subtle asymmetry of the acid urate anion, it is possible that disorder and stacking faults occur in the structure. This could explain the ‘low quality’ and low resolution, observed in the PXRD patterns, not only for our natural marchettiite sample, but also for the synthetic ammonium hydrogen urate (Friedel et al., Reference Friedel, Bergmann, Kleeberg and Schubert2006).
FTIR and Raman spectroscopy
A satisfying match between marchettiite and the synthetic analogue is given by the FTIR data (Benramdane et al., Reference Benramdane, Bouatia, Idrissi and Draoui2008 and Sekkoum et al., Reference Sekkoum, Cheriti, Taleb and Belboukhari2016) (Table 6). However, by comparison with the literature data, it appears evident that marchettiite, at least for the sample analysed by FTIR, is not a pure material but is intermixed with whewellite. Indeed, the bands at ~3175, 2891, 1540 and 768 cm–1 are present for our marchettiite spectrum and the spectrum by Sekkoum et al. (Reference Sekkoum, Cheriti, Taleb and Belboukhari2016) (see their table 3), which also contains whewellite.
The infrared characteristic broad bands of marchettiite in the range 3200–2600 cm–1 correspond to N–H stretching of heterocyclic and ammonium groups. Other strong and sharp bands are: carbonyl (C=O) of urea groups at 1634–1640 cm–1; conjugated carbonyl band at 1590–1596 cm–1; valence stretching of =C–O at 1541 cm–1; three bands around 1428–1432, 1388–1383 and 1341–1345 cm–1 corresponding to N–H deformation; stretching vibration of O=C–H at 1274 cm–1; other vibrations between 1139 and 1000 cm–1 are attributed to the C–N stretching mode (Sekkoum et al., Reference Sekkoum, Cheriti, Taleb and Belboukhari2016).
In terms of Raman spectroscopy, we can state that the marchettiite spectrum and that of its synthetic analogue (Santos et al., Reference Santos, Carcía-Ramos, Bellanato, Carmona, Cifuentes-Delatte, Vahlensieck and Gasser1984) are nearly identical as shown in Fig. 5b. On the basis of Daudon et al. (Reference Daudon, Protat, Reveillaud and Jaeschke-Boyer1983), the peak at 1418 cm–1 corresponds to C ═ N stretching, the peak at 1215 and 1058 cm–1 are related to C–O stretching and O–H bending, whereas the peak at 1001 cm–1 is probably due to the ring vibration. The two peaks at 880 and 792 cm–1 correspond to the N–H out of plane bending and ring vibration, respectively, whereas the peaks at 633 and 585 cm–1 are due to the ring breathing and ring vibration, respectively; additionally, the peak at 490 cm–1 is a ring vibration.
Environment of formation of marchettiite
Considering that the analogue of marchettiite is known to be present in urinary stones, it is reasonable to think that the circulation of meteoric waters at temperatures between 20–30°C may have contributed to the formation of this new mineral at Mount Cervandone. Such an assertion is backed up by the fact that seven organic minerals have now been found at Mount Cervandone, and all of them were collected in a cleft associated with classic Alpine fissure minerals such as quartz, albite and muscovite. Deveroite-(Ce) is among these organic compounds: it is the natural hydrated REE-bearing analogue of the La, Ce and Nd synthetic oxalate compound (Ollendorf and Weigel, Reference Ollendorff and Weigel1969; Gammons and Wood, Reference Gammons and Wood2000) probably formed by circulation of meteoric waters at low temperatures, between 20 and 30°C, that may occur on the rock surfaces during summer at 2500 m of altitude in the Italian Alps. Tinnunculite is another anhydrous uric acid (Pekov et al., Reference Pekov, Chukanov, Yapaskurt, Belakovskiy, Lykova, Zubkova, Shcherbakova, Britvin and Chervonnyi2017) also described recently at Mount Cervandone (Guastoni et al., Reference Guastoni, Nestola and Zorzi2020); it is undoubtedly a natural organic mineral formed under the action of atmospheric agents in a geological environment with bird excrement mineralised on the surface of cliffs and rock fragments.
Nevertheless, the IMA–CNMNC considers that if a mineral derives from animal or organic waste it may be approved as a new mineral if the processes responsible for the crystallisation of the new compound excludes any human influence (Nickel and Grice, Reference Nickel and Grice1998). Marchettiite was formed from the transformation of animal excrement of birds or marmots at temperatures between 20 and 30°C – temperatures that may occur from the circulation of surface waters on the rock surfaces during the summer season on Mount Cervandone.
Further studies will clarify whether more organic samples are present in this area and what the potential is for such a deposit to exist. Natural organic phases have been previously overlooked because Mount Cervandone is renowned internationally as a mineral deposit for inorganic mineral phases formed during Alpine geological processes.
Acknowledgements
The authors thank Stefano Castelli who photographed the holotype of marchettiite. This work was supported by the “Progetto di Ateneo 2014, University of Padova, grant n. CPDA140255” to Prof. Luciano Secco.
Supplementary material
To view supplementary material for this article, please visit https://doi.org/10.1180/mgm.2022.97
Competing interests
The authors declare none.