Introduction
Performing daily activities is challenging for individuals with a transfemoral amputation. It is acknowledged that they have a higher metabolic cost and reduced physical performance during level and slope walking, stair climbing and rising from a chair compared to able-bodied individuals (Highsmith et al., Reference Highsmith, Kahle, Carey, Lura, Dubey, Csavina and Quillen2011; Lura et al., Reference Lura, Wernke, Carey, Kahle, Miro and Highsmith2015; Ledoux et al., Reference Ledoux, Member and Goldfarb2017; Esposito et al., Reference Esposito, Rábago and Wilken2018). Movement patterns also differ compared to able-bodied individuals, for example, people with a transfemoral amputation often ambulate stairs step-by-step, whereas step-over-step is common for able-bodied individuals (Ledoux et al., Reference Ledoux, Member and Goldfarb2017). Furthermore, an asymmetrical walking pattern is observed, and balance and stability are disturbed due to the lack proprioceptive information and loss of motor control (Williams et al., Reference Williams, Turner, Orendurff, Segal, Klute, Pecoraro and Czerniecki2006; Eberly et al., Reference Eberly, Mulroy, Gronley, Perry, Yule and Burnfield2014). These daily challenges and movement adaptations lead to the development of secondary complications such as lower back problems and discomfort of other joints (Wurdeman et al., Reference Wurdeman, Stevens and Campbell2018).
Common passive and quasi-passive lower limb prosthetic devices can already restore human functioning to a certain degree. However, the ultimate goal of prosthetic development is to restore at least part of daily participation so that the quality of life of individuals with a transfemoral amputation increases. Recent technological breakthroughs have evolved toward motorized prostheses (Martinez-Villalpando et al., Reference Martinez-Villalpando, Mooney, Elliott and Herr2011; Simon et al., Reference Simon, Fey, Finucane, Lipschutz and Hargrove2013, Reference Simon, Ingraham, Fey, Finucane, Lipschutz, Young and Hargrove2014; Takahashi et al., Reference Takahashi, Horne and Stanhope2015; Wu et al., Reference Wu, Haque and Shen2017). Although some devices (e.g., the Power Knee from Össur, Reykjavik, Iceland) already reached the market, they are still under-prescribed and under-utilized due to the large costs of product development and customization (Lechler et al., Reference Lechler, Frossard, Whelan, Langlois, Müller and Kristjansson2018). Moreover, current motorized prostheses mainly focus on improving walking abilities, whereas emphasis on other daily activities is also of highly importance (Ghillebert et al., Reference Ghillebert, De Bock, Flynn, Geeroms, Tassignon, Roelands, Lefeber, Vanderborght, Meeusen and De Pauw2019).
The CYBERLEGs-gamma (CLs-γ) prosthesis (Figure 1), the successor of the beta-version, has recently been designed and constructed by engineers from The CLs-γ prosthesis (Figure 1), the successor of the beta-version, has recently been designed and constructed by engineers from Vrije Universiteit Brussel (Flynn et al., Reference Flynn, Geeroms, Van Der Hoeven, Vanderborght and Lefeber2018). Conceptually the prosthesis is similar to the beta-version, but more power was added to better assist individuals with a transfemoral amputation during more demanding tasks and adaptations have been made for improving robustness that allow for longer and more frequent test sessions. These improvements enabled to perform several simulated daily activities that were not investigated with the previous prototype (Flynn et al., Reference Flynn, Geeroms, Jimenez-Fabian, Vanderborght, Vitiello and Lefeber2015; Parri et al., Reference Parri, Martini, Geeroms, Flynn, Pasquini, Crea, Lova, Lefeber, Kamnik, Munih and Vitiello2017).
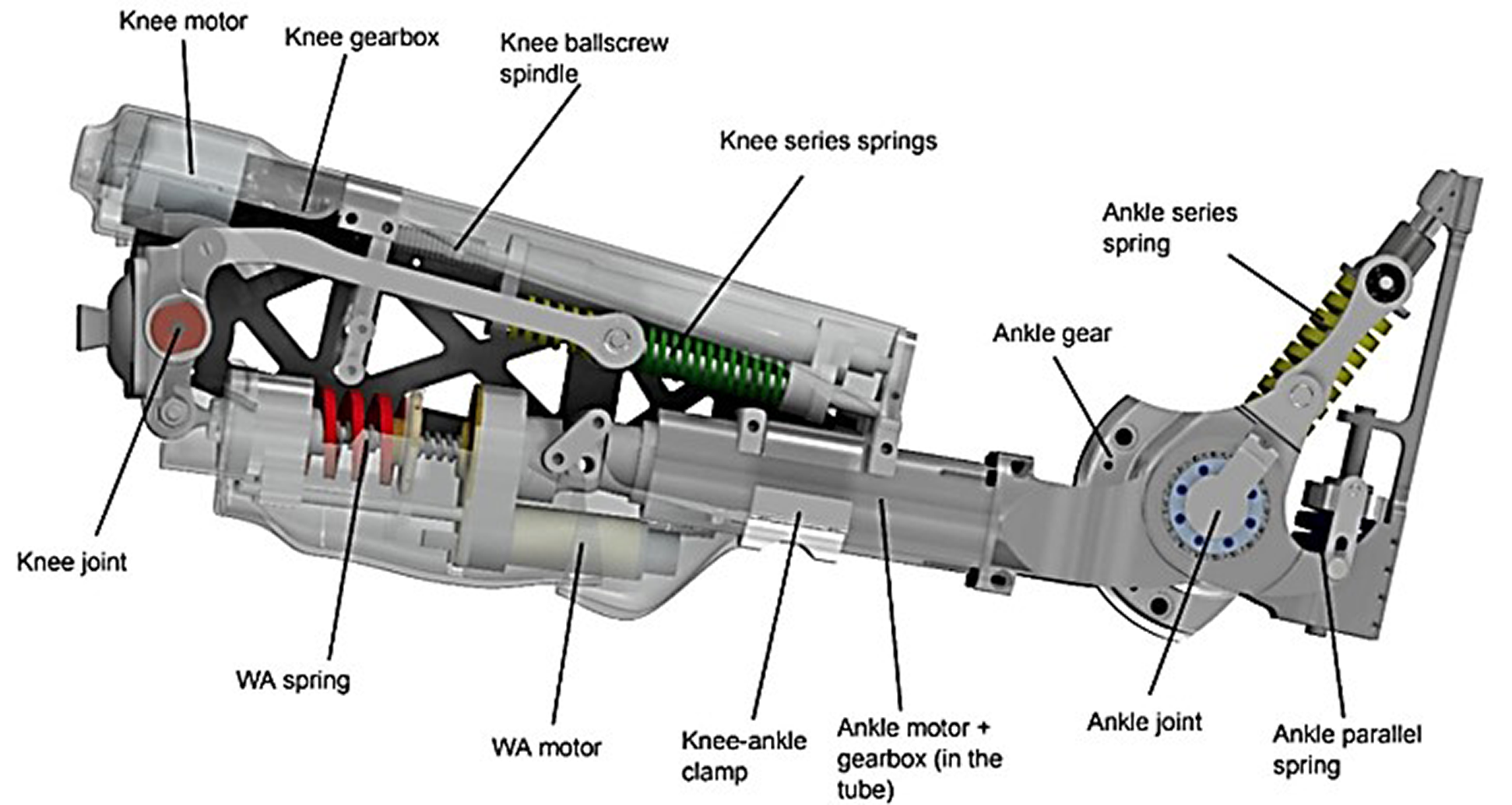
Figure 1. The CYBERLEGs-gamma prosthesis. The knee actuator consists of the motor, gearbox, and spindle, and the springs in series, acting on the knee joint through metal beams. The wearable apparatus consists of a motor moving the spring in and out of place. The ankle actuator consists of the motor, gearbox, and series and parallel springs acting on the ankle joint. The ankle and knee are clamped together, allowing a change in distance between the joints.
At the ankle joint, an actuator provides torque by compressing series elastic springs, whereas a parallel spring system acts between the shank and the foot to reduce energy consumption by storing potential and kinetic energy during dorsiflexion and releasing it during plantarflexion (Geeroms et al., Reference Geeroms, Flynn, Jimenez-Fabian, Vanderborght and Lefber2017). At the knee joint, the actuator provides resistance also through a series elastic actuator, similar to eccentric muscle power, during the weight acceptance phase and is activated right before heel strike. Afterwards, the controller provides active torque, similar to concentric muscle power, during the stance phase when the knee is extending. The motion is constrained to the sagittal plane, that is, flexion and extension. The design of the actuators in the prosthesis is based on healthy human joint pseudo-stiffnesses during preferred walking on level ground. The control of the device makes use of the CLs wearable sensory apparatus, comprising inertial measurements units placed on legs and pelvis, and force-sensitive insoles. Gait phases are automatically detected based on these sensors, using thresholds in force and angular position to transition between them (Parri et al., Reference Parri, Martini, Geeroms, Flynn, Pasquini, Crea, Lova, Lefeber, Kamnik, Munih and Vitiello2017). To avoid false detection of states and possible hazards, the high-level intention detection constrained the device discretely by selecting the correct task to be executed. The CLs-ɣ prosthesis comprises the motor drivers and control electronics in the leg structure and requires an external power source (battery pack) placed on the pelvis. Electronics of the system are custom made boards to control not only the prosthesis, but also act as interface between the force-sensitive insoles that are instrumented in the shoes of both feet and the inertial measurement units that are attached to the trunk and lower limbs (Crea et al., Reference Crea, Donati, De Rossi, Maria Oddo and Vitiello2014).
Since novel design changes have been made, and more daily activities are being supported by the device, a thorough evaluation process incorporating biomechanical, physiological, psychological, and physical performance parameters is recommended (Ferreira et al., Reference Ferreira, Noble and Biddle2007; Sutherland and Schwaber, Reference Sutherland and Schwaber2007; Ghillebert et al., Reference Ghillebert, De Bock, Flynn, Geeroms, Tassignon, Roelands, Lefeber, Vanderborght, Meeusen and De Pauw2019). Besides investigating the physical effort when using the prosthetic prototype, cognitive performance is also determined during a dual task (Pauley and Devlin, Reference Pauley and Devlin2011; Meier et al., Reference Meier, Hansen, Gard and Mcfadyen2012; Knaepen et al., Reference Knaepen, Marusic, Crea, Rodríguez Guerrero, Vitiello, Pattyn, Mairesse, Lefeber and Meeusen2015; Morgan et al., Reference Morgan, Hafner and Kelly2016; Morgan et al., Reference Morgan, Hafner, Kartin and Kelly2018; De Pauw et al., Reference De Pauw, Cherelle, Tassignon, Van Cutsem, Roelands, Marulanda, Lefeber, Vanderborght and Meeusen2019). Performing a cognitive and motoric task simultaneously is interesting since people overperform common walking trials in a laboratory setting, whereas dual task walking better mimic everyday walking and offer a perspective that laboratory measurements cannot show (Urbanek et al., Reference Urbanek, Zipunnikov, Harris, Crainiceanu, Harezlak and Glynn2018). Dual task paradigms including walking and an attentional task allow to investigate gait pattern alterations and attentional resources used during the motoric task (Krasovsky et al., Reference Krasovsky, Weiss and Kizony2017).
The objective of this study was to thoroughly evaluate the CLs-ɣ prosthesis during simulated daily activities in laboratory conditions. We hypothesized improved physical performance during stair climbing, sit to stand and walking with the CLs-ɣ compared to the current prosthesis given the limited training time with the novel prosthesis. It was also hypothesized that walking with the CLs-ɣ prosthesis would restore a more symmetrical walking pattern (step length and width, stance and swing phases, and heel pressure), and decrease physical effort (metabolic cost, heart rate and rating of perceived exertion) and cognitive load (reaction time) compared to the current prosthesis.
Materials and Methods
Recruitment
Nine participants with a transfemoral amputation (K-level > 2) were enrolled in the study (Borrenpohl et al., Reference Borrenpohl, Kaluf and Major2016). The recruitment process was performed by the physiotherapist of an orthopedic rehabilitation and research center (VIGO, Wetteren, Belgium), where experiments took place. Participants were not financially compensated but did receive transportation costs. Medicare Functional Classification level was determined by two independent physiotherapists (Jo Ghillebert and Patrick Van de Vaerd) and in case of disagreement a medical doctor was contacted. Study registration was completed at ClinicalTrials.gov under NCT03376919, and approved by the institutional medical ethics committee of UZ Brussel and Vrije Universiteit Brussel (Belgium, B.U.N. 143201732970) and the Federal Agency for Medicines and Health Products (reference number: 80M0725). All participants were provided written and verbal information about the experimental protocol, potential risks and benefits before giving informed consent to participate in the study.
Experimental Protocol
Each participant visited the laboratory six times: a familiarization session, an experimental session with their current prosthesis, three training sessions with the CLs-γ prosthesis and an experimental session with the CLs-γ prosthesis (Figure 2) (within subject design). All laboratory visits took place in the morning at the same hour and at least 24 hr between each session was planned to counteract fatigue. The total duration of the experiment was 8 months. The environmental humidity, pressure and temperature of the laboratory was 48 ± 6%, 764 ± 8 mmHg and 24.5 ± 1.6°C; respectively.
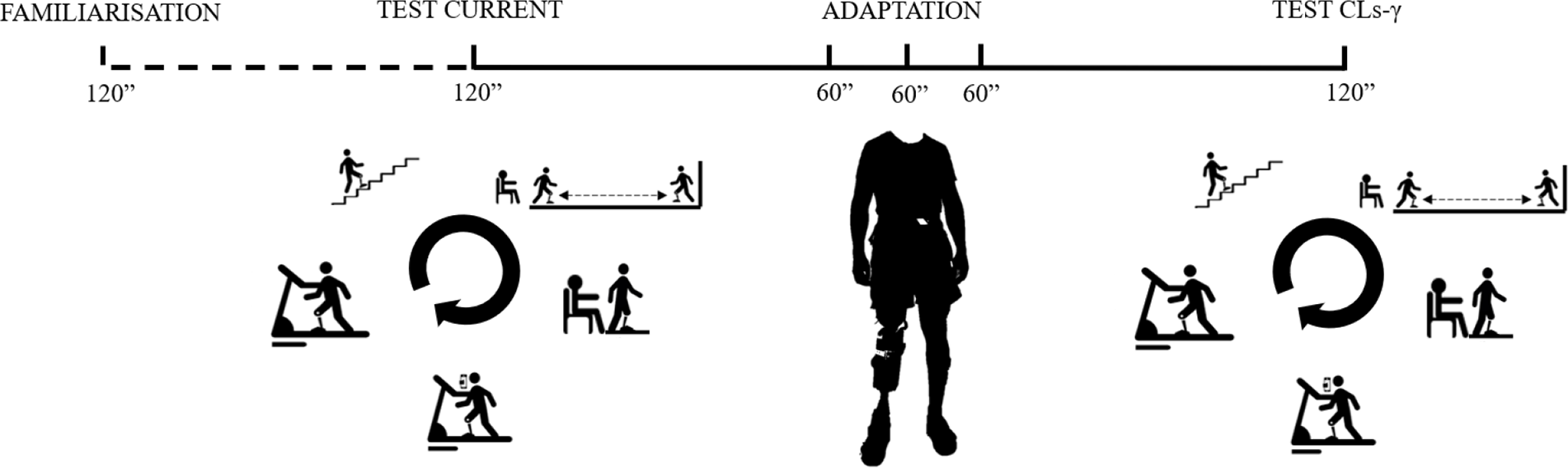
Figure 2. Experimental protocol including a familiarization trial—an experimental trial with the current prosthesis (passive or quasi-passive device)—three adaptation sessions to the novel prosthesis (i.e., the CYBERLEGs-gamma [CLs-γ] prosthesis)—an experimental trial with the CLs-γ prosthesis. The five tasks were a stair climbing test, a timed-up and go test, a sit to stand test, a 2-min dual task and 6-min treadmill walk test.
The familiarization session (120 min) aimed to accustom participants to the experimental protocol, to get used to the measurement devices and to determine the participants’ preferred walking speed. Preferred walking speed was determined on the treadmill through verbal feedback from the participant. The investigator altered the walking speed until the participant confirmed the speed was consistent with the preferred walking speed (Graham et al., Reference Graham, Ostir, Fisher and Ottenbacher2008). After the familiarization session, the preferred walking speed was used during the experimental treadmill sessions with the current and CLs-γ prosthesis.
The experimental sessions (120 min per session) with the current and CLs-γ prosthesis consisted of five consecutive tasks with 10 min of rest between each task. Participants started with the stair climbing test (Bennell et al., Reference Bennell, Dobson and Hinman2011) (SCT; intraclass correlation coefficient [ICC] ascent: 1.00, descent: 0.89 [Highsmith et al., Reference Highsmith, Kahle, Kaluf, Miro, Mengelkoch and Klenow2017]). They started in front of a staircase (four steps) and were asked to ascend and descend in a safe way as fast as possible. The use of bilateral handrails was allowed when it was difficult to maintain balance. The ascending phase was initiated with the prosthetic side, whereas the first step of the descending phase was performed with the nonprosthetic leg. The second task was a timed-up and go test (Bennell et al., Reference Bennell, Dobson and Hinman2011) (TUG; ICC: 0.88 [Resnik and Borgia, Reference Resnik and Borgia2011]). Participants stood up from their chair (44 cm total height, without arm support and a straight back), walked three meters, turned around, returned to their chair and ended in a seated position. Next, a sit to stand test (Jones et al., Reference Jones, Rikli and Beam2013) (STS; ICC: 0.84–0.92 [Jones et al., Reference Jones, Rikli and Beam2013]) was performed. Participants rose as often as possible from their chair in 30 s without support of their hands. Afterwards, a dual task was performed on a treadmill. Participants walked for 2 min on a treadmill at their preferred walking speed while performing the psychomotor vigilance task (Wilkinson and Houghton, Reference Wilkinson and Houghton1982) (ICC: 0.82 [Wilkinson and Houghton, Reference Wilkinson and Houghton1982]). Participants had to respond by pushing on a button with the index finger of their dominant hand. Earplugs were required during the task to reduce distraction related to sound. The visual stimulus, displayed as a red dot, was visualized on a dark computer screen with a random time interval between 1,000 and 10,000 ms. The interval stimulus-response onset was set at 500 ms and the distance to the screen was approximately 1 m. The experiments ended with a 6-min walk test (Crapo et al., Reference Crapo, Enright and Zeballos2002) (6MWT; ICC: 0.94–0.97 [Lin and Bose, Reference Lin and Bose2008]). Participants walked on a treadmill for 6 min at their preferred walking speed. The order of the experimental tasks was determined according to fattigue level from low to high.
Between the experimental sessions with the current and CLs-γ prosthesis, participants underwent three training sessions (60 min per session). The focus of each training session differed: (a) socket fit and walking, (b) alignment and SCT, and (c) STS and TUG. A duplicate of the participants’ current socket was made to optimize fitting and alignment of the novel prosthesis. Participants were fitted and aligned by a certified prosthetist. During each training session, participants also performed hallway walking for 10 min.
Dependent Measures
Physical performance determinants were gathered in terms of duration (s) of the SCT and TUG, number of cycles during the STS, reaction time (ms) during the psychomotor vigilance task and preferred walking speed (m/s) during the 6MWT. Following spatiotemporal and kinetics data were recorded during walking: cadence (steps/min), maximum heel pressure (N/cm2), stance and swing phases (% of gait cycle), step width (cm) and stride length (cm) were reported. Physiological outcome measures were collected in terms of heart rate (bpm) and rating of perceived exertion (score between 0 and 10) after each experimental task. Additionally, oxygen uptake (mL min−1 kg−1), ventilation (L/min) and metabolic equivalents were gathered during the 6MWT. Psychological outcome measures were determined, such as the visual analogue scale (score on 100) for fatigue and the EuroQol-5D (score on 100) to determine health status (Sung and Wu, Reference Sung and Wu2018; Van Reenen et al., Reference Van Reenen, Oppe, Boye, Herdman, Kennedy-Martin, Kenndy-Martin and Slaap2018). Other questionnaires were completed during the familiarization session, that is, the prosthetic evaluation questionnaire and the system usability scale (Brooke, Reference Brooke1996; Wurdeman et al., Reference Wurdeman, Stevens and Campbell2018).
Measurement Devices and Data Analysis
Spatiotemporal and kinetics data were collected with the Zebris software (Medical GmbH, Isny, Germany). Ground reaction force time-series were automatically reduced to zero-dimensional maxima under the forefoot, midfoot and heel. Cadence, stance and swing phases, step width, step and stride length were continuously determined. Gait variability, expressed as the coefficient of variation, was calculated for step width, and step and stride length from the standard deviation dividing by the mean (Guimaraes and Isaacs, Reference Guimaraes and Isaacs1980). Ergospirometrical data were continuously gathered during the 6MWT using a portable system (Cosmed K5, Cosmed, Rome, Italy). Preceding each test, a calibration (volume, ambient air and reference gas) was performed after a system warm-up period of 30 min. The mixed chamber setting was used, and data was continuously transferred to the Omnia program (Cosmed, Rome, Italy). The device was mounted on the back of the participant with a harness. The net metabolic cost of transport (mL m−1 kg−1) was calculated from dividing the relative oxygen uptake (mL min−1 kg−1) by the product of the duration of the test (min), the weight (kg) of the participant and the distance (m) covered during the test (Workman and Armstrong, Reference Workman and Armstrong2017). Heart rate was measured at the end of each task with an elastic belt strapped around the chest (Polar M400, H7-sensor, Kempele, Finland). The performance outcome measure of the psychomotor vigilance task was reaction times, measured with E-prime 3.0 (Psychology Software Tools, Sharpsburg, MD).
Statistical Analysis
Data are presented as mean ± standard deviation. SPSS version 25.0 (IBM, New York, NY) was used for statistical analyses. Shapiro Wilk normality tests showed that most datasets were not normally distributed. Therefore, nonparametric Wilcoxon-signed rank tests were conducted. The critical alpha for all analyses was set at 0.05. Hedges’ effect sizes were calculated from dividing the absolute standard test statistics by the square root of the number of observations (Rosenthal, Reference Rosenthal, Cooper and Hedges1994). Small, medium and large effects were considered as 0.2, 0.5, and 0.8, respectively.
Results
One participant withdrew after the first session for reasons not related to the study. Therefore, data analysis was performed on eight participants (age: 55 ± 15 years; height: 174 ± 5 cm; weight: 81 ± 11 kg; Table 1). An equal number of participants had an amputation of the right or left lower limb. Four participants’ current prosthesis was passive, the others wore a quasi-passive device (microprocessor-controlled). Years since amputation varied among participants (22 ± 14 years), but they were all familiar with their current prosthesis for a minimum duration of 3 months.
Table 1. Participants’ characteristics: demographic data, prosthetic components, and walking speed

Note: All participants were indicated as Medicare Functional Classification Level K3 ambulators and had their current prosthesis for at least 3 months.
Abbreviations: AMP, amputated; CLs-γ, CYBERLEGs-gamma; F, female; L, left; M, male; SACH, solid ankle cushion heel.
Biomechanical Outcomes
Stride length significantly increased when walking with the CLs-γ compared to the current prosthesis (17 ± 10%, p = .012; Table 2 and Figure 3a). The increased stride length was due to a greater step length of the prosthetic leg (22 ± 20%, p = .035; Table 2 and Figure 3b). No significant difference in step length of the nonprosthetic leg was reported (Figure 3b) as well as for step width and gait phases. Additionally, coefficients of variation did not differ between both prostheses for stride length, step width, and step length of the amputated and nonprosthetic leg. Maximum heel pressure of the amputated and nonprosthetic leg did not change while walking with the current compared to the CLs-γ prosthesis.
Table 2. Dependent variables are presented as mean and standard deviation with the current and CYBERLEGs-gamma prosthesis, their corresponding p-value, absolute standard test value Z and effect size

Abbreviations: AMP, prosthetic leg; CLs-γ, CYBERLEGs-gamma; CV, coefficient of variation; EQ-5D, EuroQol-5D; N-AMP, nonprosthetic leg; RPE, rating of perceived exertion; SCT, stair climbing test; STS, sit to stand test; TUG, timed-up and go test; VAS, visual analogue scale; 6MWT, 6-min walk test.
Bold entries are significant (<0.05).

Figure 3. Mean and standard deviation for stride (a) and step length (b) are presented while walking with the current compared to the CYBERLEGs-gamma (CLs-γ) prosthesis. AMP, amputated leg; N-AMP, non-amputated leg.
Physiological Outcomes
Heart rate at the end of each task did not change when wearing the current compared to the CLs-γ prosthesis. The amount of oxygen consumption, ventilation and metabolic equivalents did not vary between walking with both prostheses. However, the net metabolic cost of transport significantly increased when wearing the CLs-γ compared to the current prosthesis during the 6MWT (p = .028; Table 2 and Figure 4).

Figure 4. Representation of the net metabolic cost per meter during the 6-min treadmill walk test with the current compared to the CYBERLEGs-gamma (CLs-γ) prosthesis.
Psychological Outcomes
No differences in rating of perceived exertion and the visual analogue scale for fatigue were reported during the different tests between the current and CLs-γ prosthesis. The self-reported scores on the EuroQol-5D did not significantly change between both prostheses.
Physical Performance Outcomes
Figure 5 shows that the preferred walking speed was significantly lower with the CLs-γ compared to the current prosthesis (p = .018; Table 2). Cadence significantly decreased while walking with the CLs-γ compared to the current prosthesis (p = .012; Table 2). Furthermore, participants needed significantly more time to respond to the stimulus of the psychomotor vigilance task while walking with the CLs-γ compared to the current prosthesis (p = .012; Table 2). Participants also needed significantly more time to complete the SCT and TUG with the CLs-γ compared to the current prosthesis (p = .012 and p = .012, respectively; Table 2). Number of stands during the STS did not differ between both prostheses.

Figure 5. Individual (Williams et al., Reference Williams, Turner, Orendurff, Segal, Klute, Pecoraro and Czerniecki2006; Highsmith et al., Reference Highsmith, Kahle, Carey, Lura, Dubey, Csavina and Quillen2011; Eberly et al., Reference Eberly, Mulroy, Gronley, Perry, Yule and Burnfield2014; Simon et al., Reference Simon, Ingraham, Fey, Finucane, Lipschutz, Young and Hargrove2014; Lura et al., Reference Lura, Wernke, Carey, Kahle, Miro and Highsmith2015; Ledoux et al., Reference Ledoux, Member and Goldfarb2017; Esposito et al., Reference Esposito, Rábago and Wilken2018; Wurdeman et al., Reference Wurdeman, Stevens and Campbell2018) and group average (MEAN) walking speed (mean and standard deviation) with the current (◊) vs. the CYBERLEGs-gamma (CLs-γ) (□) prosthesis are displayed.
Discussion
This study thoroughly evaluated a novel prosthetic prototype during simulated daily activities in individuals with a transfemoral amputation in order to provide an overview on the global functioning of the device as well as more specific information for the next design iteration. The main finding was that the novel motorized prosthesis failed to enhance walking symmetry, physical effort and attentional demand during simulated daily activities compared to current devices. Weight dependent tasks (SCT, TUG, and the 6MWT) were performed worser with the CLs-γ prosthesis compared to the current devices except the increase in stride length during the 6MWT and the way of negotiating stairs. The motorized knee and ankle joint enabled participants to climb the stairs step-over-step, which is impossible with the currently used prosthetic devices where a step-by-step strategy is opted. The weight of a prosthetic device has always been a challenge and especially for motorized two-joint prostheses. Therefore, the recommendation for the next design iteration is to eliminate the weight (current weight of the ankle and knee joint is 2.3 and 3.0 kg, respectively) by selecting carbon-fiber composites and downsizing actuators.
People with a transfemoral amputation have a shorter stride length when walking with their current prosthesis compared to able-bodied individuals, because of the increased moment of inertia of the prosthetic limb during swing phase in typical prostheses (Uchytil et al., Reference Uchytil, Jandacka, Zahradnik, Farana and Janura2014). The CLs-γ prosthesis actively flexes during the swing phase, consequently the lever arm decreases between the hip joint (center of rotation) and the center of mass of the prosthesis. Considering the total energy input of the gait cycle remains the same between the current and CLs-γ prosthesis, hence causes an increased angular velocity (Fowles and Cassiday, Reference Fowles and Cassiday2000). The greater angular velocity explains the greater stride length. Although stride length significantly increased with the novel prosthesis, design adaptations are still needed to match able-bodied stride lengths (CLs-γ: 99 ± 11 cm vs. able-bodied: 157 ± 5 cm) (Mahon et al., Reference Mahon, Pruziner, Hendershot, Wolf, Darter, Foreman and Webster2017). Stride length mainly increased as a result from a larger step length of the amputated leg. Though, this exacerbated the asymmetrical walking pattern and did not contribute to a higher symmetry between the amputated and non-amputated leg. Gait variability of stride length, a measure for gait symmetry, confirmed this result as it did not significantly differ between the CLs-γ and current prosthesis, and thus an asymmetrical walking pattern remains present (Guimaraes and Isaacs, Reference Guimaraes and Isaacs1980).
From a device evaluation perspective this study shows that experimental set-ups can be designed in such a way that multidisciplinary data can be gathered to provide a broad overview of the functioning of the device and the human–prosthetic interaction. The included dual-task paradigm provided a comprehensible insight in how the novel prosthesis negatively influenced the motoric task and attentional demand during both the cognitive and motoric task which is in line with previous findings (Pauley and Devlin, Reference Pauley and Devlin2011; Meier et al., Reference Meier, Hansen, Gard and Mcfadyen2012; Knaepen et al., Reference Knaepen, Marusic, Crea, Rodríguez Guerrero, Vitiello, Pattyn, Mairesse, Lefeber and Meeusen2015; Morgan et al., Reference Morgan, Hafner and Kelly2016; Morgan et al., Reference Morgan, Hafner, Kartin and Kelly2018; De Pauw et al., Reference De Pauw, Cherelle, Tassignon, Van Cutsem, Roelands, Marulanda, Lefeber, Vanderborght and Meeusen2019). Decreased task and cognitive performance demonstrate task interference, since both walking and the cognitive task require signal processing in similar brain areas (Nijboer et al., Reference Nijboer, Borst, van Rijn and Taatgen2014). The participants experienced an adaptation, which increased the neural load of wearing the novel prosthesis that is more important than performing an automated pattern such as walking. Theories of central processing assume that attention has a fixed capacity of processing information and that interferences occur at the stimulus identification stage in the occipital lobe (Norman, Reference Norman1969). Since stimulus processing is limited, a conservative walking pattern is adopted, consisting of a reduced walking speed and wider step width (Morgan et al., Reference Morgan, Hafner and Kelly2016). This conservative walking pattern led to reduced postural control demands and minimizes cognitive resources (Morgan et al., Reference Morgan, Hafner, Kartin and Kelly2018). Furthermore, a meta-analysis showed that prosthetic training and rehabilitation can reduce brain information processing demands because of sensorimotor adaptations (Ghai et al., Reference Ghai, Ghai and Effenberg2017).
Besides design recommendations focused on mechanics, electronics might also be improved to provide necessary sensory information. Advancements have been made in the direction of human-in-the-loop optimization strategies where controller parameters could benefit from biological measures such as the metabolic cost (Handford and Srinivasan, Reference Handford and Srinivasan2016). Passive and active loops, and torque-angle and torque-time optimization could benefit movement efficiency (Handford and Srinivasan, Reference Handford and Srinivasan2018). For example, implementing a push-off control strategy in a motorized ankle prosthesis has shown to improve gait symmetry and reduce the metabolic cost with 14 ± 8% (Feng and Wang, Reference Feng and Wang2017). Therefore, improving performance of the device goes hand in hand with further developments of control algorithms next to the examination in testbeds or emulators (Caputo and Collins, Reference Caputo and Collins2014). This highlights the need to integrate more sophisticated control systems taking into consideration individual responses when using a robotic device.
Until now, it remains a challenge for two-joint synchronized devices with impedance or kinematic control-based mechanisms to improve daily functioning. Although high expectations toward improved performance are present, numerous papers reported that motorized devices fail to meet expectations, and thus still need to be further developed (Sup et al., Reference Sup, Varol, Mitchell, Withrow and Goldfarb2009; Simon et al., Reference Simon, Fey, Finucane, Lipschutz and Hargrove2013, Reference Simon, Ingraham, Fey, Finucane, Lipschutz, Young and Hargrove2014; Ingraham et al., Reference Ingraham, Fey, Simon and Hargrove2016). Thus, the thorough examination of the CLs-γ prosthesis also demonstrated premature for market implementation. Though some improvements have been noticed, especially during stair ascending and descending where participants were again able to walk the stairs step-over-step. This reciprocal stair ambulation shows the possible functional capacity of motorized lower limb prostheses. The CLs-γ prosthesis was able to restore stair climbing due to design approximating pseudo-stiffness of the non-amputated joints, which determined the stiffness and bandwidth of the artificial joints. The CLs-γ prosthesis differs from other prototypes since the finite state controller relies on pressure-sensitive insoles in the shoes and inertial measurement units attached to the trunk and lower limbs.
The choice of 3 hr of training with the CLs-γ prosthesis was based on a study that reported clinically relevant outcomes for further development of a prototype after a few hours of training with a powered ankle and knee prosthesis (Simon et al., Reference Simon, Ingraham, Fey, Finucane, Lipschutz, Young and Hargrove2014). However, this limits the results to short-term use of a novel device. To determine long-term adaptations, a training period of 3 months with a device of at least a technology readiness level 6, which refers to a model or prototype that can be demonstrated in a relevant environment, is advised (Assistant Secretary of Defense, 2011). Another limitation of this study is with regards to the study design. Future research should consider exploring the influence of years of amputation on outcome measures during an evaluation procedure. A randomized controlled study design is encouraged going forward.
To conclude, a multidisciplinary approach was adopted to evaluate a novel motorized lower limb prosthetic device. Increased physical effort and cognitive load were required during walking with the CLs-ɣ prosthesis compared to the current prosthesis. All participants were able to conduct stairs with the step-over-step instead of the step-by-step strategy.
Acknowledgments
We would like to thank Patrick Van De Vaerd and colleagues of V!GO (9230 Wetteren, Belgium) for their assistance during the experimental trials. We would also like to thank the company V!GO for providing their research laboratory.
Funding Statement
This work was supported by EUROBENCH project under Grant No. 779963 and Innoviris under The Talaris Project. This work was also supported by the European Union’s Horizon 2020 Research and Innovation Program “The CYBERnetic LowEr-Limb CoGnitive Ortho-Prosthesis Plus Project (CYBERLEGs++; ICT-2015)” under Grant No. 731931.
Competing Interests
The authors declare no competing interests exist.
Authorship Contributions
J.G.H. substantially contributed to the conception of the methods used, participant recruitment, data acquisition, interpretation, and analysis, and writing the manuscript. J.G.E. and L.F. made substantial contributions to the conception of the methods used, participant recruitment, and data acquisition, interpretation, and analysis. S.D.B., R.G., E.L., and K.D.P. made substantial contributions to conception, design, and writing the manuscript. S.C., N.V., R.M., and D.L. were involved in the conception of the methods used, data interpretation, and writing the manuscript. All authors read and approved the final manuscript.
Data Availability Statement
Data content sharing is applicable via special request for data sharing. Please contact the corresponding author.
Ethical Standards
The authors assert that all procedures contributing to this work comply with the ethical standards of the relevant national and institutional committees on human experimentation and with the Helsinki Declaration of 1975, as revised in 2008 and “The authors assert that all procedures contributing to this work comply with the ethical standards of the relevant national and institutional guides on the care and use of laboratory animals.”
Notations
- AMP
-
amputated
- CLs-ɣ
-
CYBERLEGs-gamma
- CV
-
coefficient of variation
- EQ-5D
-
EuroQol-5D
- F
-
female
- L
-
left
- M
-
male
- N-AMP
-
non-amputated leg
- R
-
right
- RPE
-
rating of perceived exertion
- SCT
-
stair climbing test
- STS
-
sit to stand test
- TFA
-
transfemoral amputation
- TUG
-
timed-up and go test
- VAS
-
visual analogue scale
- 6MWT
-
six-min treadmill walk test