Introduction
Studies of fear learning, using Pavlovian conditioning (Pavlov, Reference Pavlov1927), have greatly advanced our understanding of the psychobiological mechanisms of posttraumatic stress disorder (PTSD), which is characterized by symptoms such as re-experiencing, avoidance, hyperarousal, and alterations in mood and cognition (American Psychiatric Association, Reference Association2013). In Pavlovian fear conditioning, an originally neutral cue or context is paired with a biologically relevant stimulus [unconditioned stimulus (US)], to become a conditioned stimulus (CS, cue or context). This CS can then elicit a conditioned response that is similar to the response to the unconditioned response, without the US being present. This association between the US and the CS is learned during fear acquisition and can be overwritten during fear extinction. Deficient context learning has been at the center of recent psychobiological models of PTSD (Liberzon & Abelson, Reference Liberzon and Abelson2016; Maren, Phan, & Liberzon, Reference Maren, Phan and Liberzon2013; Shalev, Liberzon, & Marmar, Reference Shalev, Liberzon and Marmar2017) and has been associated with typical PTSD symptoms (Shalev et al., Reference Shalev, Liberzon and Marmar2017). Spatial contexts have most often been examined in contextual learning and it has been proposed that patients with PTSD may not be able to sufficiently discriminate safe from dangerous contexts and thus maintain a fear response even in safe contexts (Acheson, Gresack, & Risbrough, Reference Acheson, Gresack and Risbrough2012; Flor & Wessa, Reference Flor and Wessa2010). Deficient context learning has been associated with lower functional activity in the hippocampus and ventromedial prefrontal cortex (vmPFC; Acheson et al., Reference Acheson, Gresack and Risbrough2012; Garfinkel et al., Reference Garfinkel, Abelson, King, Sripada, Wang, Gaines and Liberzon2014; Pitman et al., Reference Pitman, Rasmusson, Koenen, Shin, Orr, Gilbertson and Liberzon2012). In addition, enhanced learning of trauma-related cues has been observed in patients with PTSD, which was associated with higher functional activity in the amygdala (Garfinkel et al., Reference Garfinkel, Abelson, King, Sripada, Wang, Gaines and Liberzon2014; Pitman et al., Reference Pitman, Rasmusson, Koenen, Shin, Orr, Gilbertson and Liberzon2012). A variety of study protocols were developed to investigate context conditioning (Lonsdorf et al., Reference Lonsdorf, Menz, Andreatta, Fullana, Golkar, Haaker and Merz2017) using virtual reality in human subjects (Glenn, Risbrough, Simmons, Acheson, & Stout, Reference Glenn, Risbrough, Simmons, Acheson and Stout2017; Kroes, Dunsmoor, Mackey, McClay, & Phelps, Reference Kroes, Dunsmoor, Mackey, McClay and Phelps2017). Two factors that are conducive to an environment being perceived as a context are its longer representation time and its higher complexity compared to single cues (Lonsdorf et al., Reference Lonsdorf, Menz, Andreatta, Fullana, Golkar, Haaker and Merz2017). The larger the time frame, in which a US can occur, the larger the unpredictability and the higher the levels of anxiety (Indovina, Robbins, Núñez-Elizalde, Dunn, & Bishop, Reference Indovina, Robbins, Núñez-Elizalde, Dunn and Bishop2011; Schmitz & Grillon, Reference Schmitz and Grillon2012). With the US being presented within a longer presentation phase of a given context it cannot be directly associated with an object within the context, which is why contexts are usually considered to be unpredictable of the US. The hippocampus and vmPFC have both been proposed to play a key role in the organization of previously learned information to make predictions and for flexible behavioral responses (Behrens et al., Reference Behrens, Muller, Whittington, Mark, Baram, Stachenfeld and Kurth-Nelson2018; Stachenfeld, Botvinick, & Gershman, Reference Stachenfeld, Botvinick and Gershman2017). In a more unpredictable environment, prediction about possible future behaviors has to be formed and tested, which requires the activity in the vmPFC and hippocampus. In previous studies, patients with PTSD showed decreased activity in both of these regions in comparison to healthy trauma- or non-trauma-exposed individuals during fear acquisition and for the vmPFC also during fear extinction (Milad et al., Reference Milad, Wright, Orr, Pitman, Quirk and Rauch2007; Rougemont-Bücking et al., Reference Rougemont-Bücking, Linnman, Zeffiro, Zeidan, Lebron-Milad, Rodriguez-Romaguera and Milad2011). Previous context conditioning studies have shown that patients with PTSD show difficulties in contextual fear acquisition, but improve when cues are added to predict if a context is dangerous or safe (Steiger, Nees, Wicking, Lang, & Flor, Reference Steiger, Nees, Wicking, Lang and Flor2015). Furthermore, patients with PTSD show reduced capacity to use context information to regulate fear responses (Garfinkel et al., Reference Garfinkel, Abelson, King, Sripada, Wang, Gaines and Liberzon2014) during fear extinction (Rougemont-Bücking et al., Reference Rougemont-Bücking, Linnman, Zeffiro, Zeidan, Lebron-Milad, Rodriguez-Romaguera and Milad2011), memory of the extinction (extinction recall; Milad et al., Reference Milad, Pitman, Ellis, Gold, Shin, Lasko and Rauch2009), and when the already extinguished fear is returning (fear renewal; Wicking et al., Reference Wicking, Steiger, Nees, Diener, Grimm, Ruttorf and Flor2016). Here, contexts were defined as steady images of an office (Garfinkel et al., Reference Garfinkel, Abelson, King, Sripada, Wang, Gaines and Liberzon2014; Rougemont-Bücking et al., Reference Rougemont-Bücking, Linnman, Zeffiro, Zeidan, Lebron-Milad, Rodriguez-Romaguera and Milad2011) or virtual reality scenes of different rooms (Wicking et al., Reference Wicking, Steiger, Nees, Diener, Grimm, Ruttorf and Flor2016), in which contexts could be distinguished by retrieving a single object from the environments, making it a predictable context. In configural learning (Acheson et al., Reference Acheson, Gresack and Risbrough2012; Rudy, Huff, & Matus-Amat, Reference Rudy, Huff and Matus-Amat2004; Rudy & O'Reilly, Reference Rudy and O'Reilly1999), multiple objects that are associated to each other form a conjunctive representation of a context. Acheson et al. (Reference Acheson, Gresack and Risbrough2012) hypothesized that lower hippocampal activity leads to an inability of configural-based learning in patients with PTSD. Instead, cue-based associations will be formed, in which a single cue is assumed to predict the occurrence of the US, independent of its predictability or the complexity of the surrounding information (Acheson et al., Reference Acheson, Gresack and Risbrough2012; Lonsdorf et al., Reference Lonsdorf, Menz, Andreatta, Fullana, Golkar, Haaker and Merz2017). Each individual cue is then potentially able to elicit a fear response across contexts, with the amygdala being more active (Phillips & Ledoux, Reference Phillips and Ledoux1992), independent of the context being safe or dangerous. Persons with PTSD show an increased responsiveness to aversive cues with an associated higher amygdala response in comparison to healthy trauma- or non-trauma-exposed individuals (Mahan & Ressler, Reference Mahan and Ressler2012).
The aim of our study was to compare an unpredictable context based on configural learning with a predictable context, in which a single cue predicted the occurrence of a US within a context in patients with PTSD and trauma-naïve and trauma-experienced controls. To this end we developed a new Virtual Reality-based, combined cue-context conditioning paradigm. This new approach defined contexts via a configural learning approach, in which the configuration of furniture in a room defined a particular context. Participants were presented with unpredictable and predictable contexts as contextual conditioned stimuli during fear acquisition and extinction. We hypothesized that patients with PTSD in comparison to non-trauma-exposed (HC) and healthy trauma-exposed (TC) subjects would (a) show lower Blood Oxygenation Level Dependent activity in the hippocampi and vmPFCs, (b) show lower skin conductance response (SCR), and (c) report higher arousal, valence, and contingency ratings during acquisition of the unpredictable context. In the predictable context, we expected higher Blood Oxygenation Level Dependent activity in the amygdalae in patients with PTSD in comparison to TC and HC subjects. During contextual fear extinction, we hypothesized higher Blood Oxygenation Level Dependent activity in the amygdalae and lower activity in the vmPFCs for patients with PTSD in comparison to both control groups in both contexts.
Methods and materials
Participants
Twenty patients suffering from PTSD, 21 age- and sex-matched TC, and 22 HC subjects participated in this study (Table 1; for details on recruitment and inclusion criteria see online Supplementary Methods). The study was carried out in accordance with the Code of Ethics of the World Medical Association (World Medical Association, 2013) and was approved by the Ethical Review Board of the Medical Faculty Mannheim, Heidelberg University. All participants gave written informed consent.
Table 1. Demographic and clinical characteristics of the study sample

ADS, Allgemeine Depressionsskala (German version of the Center for Epidemiological Studies Depression Scale (CESD)); CAPS, Clinician-Administered PTSD Scale; CFT, Culture Fair Intelligence Test; CI, Confidence Interval; Cont., contrast between groups (TC, HC, PTSD); CTQ, Childhood Trauma Questionnaire; df, degrees of freedom; F, results of F-statistics; HC, group of healthy control subjects, who never experienced trauma in their lives; KAI, Kurztest für allgemeine Basisgrößen der Informationsverarbeitung (Short Test for General Factors of Information Processing); M, mean; n, number of participants; p, probability of obtaining test result; PTSD, patients with posttraumatic stress disorder; SD, standard deviation; STAI-T, State-Trait Anxiety Inventory – Trait Anxiety; T, results of T-statistics; TC, group of trauma control subjects, who at least experienced one traumatic event but did not fulfill the criteria for PTSD; Tukey HSD, Tukey's honest significant difference test; χ2, results of χ2 test.
a Psychopharmacological medication: pregabalin; quetiapine; tetrahydrocannabinol.
b Non-psychopharmacological medication: contraceptive pill; levothyroxine; mesalazine; prednisolone.
Procedure and study design
The study consisted of two assessments on two consecutive days, each lasting for approximately 5 h. On the first day, participants completed questionnaires and clinical assessments on PTSD and participated in the Structured Clinical Interviews (SCID I + II; Fydrich, Renneberg, Schmitz, & Wittchen, Reference Fydrich, Renneberg, Schmitz and Wittchen1997; Wittchen, Wunderlich, Gruschwitz, & Zaudig, Reference Wittchen, Wunderlich, Gruschwitz and Zaudig1997). During the first experimental phase, participants completed a training and habituation phase outside the magnetic resonance imaging (MRI) scanner, while sitting in front of a computer screen with a head mounted display (HMD). Participants then determined the intensity of the painful stimulus that served as US (online Supplementary Methods) before completing the context and cue acquisition phases inside the MRI scanner. On the second day, participants took part in the context and cue extinction phases inside the MRI scanner. This was followed by a final testing phase including cognitive and neuropsychological assessments.
Stimuli and experimental procedure
During the experimental phase, participants were passively navigated through virtual contexts (living rooms) on a parabola-shaped trajectory with a constant slow-paced walking speed of 0.45 km/h and an egocentric viewpoint (Fig. 1). A total of four different contexts and two different cues (CS+, CS–, colored triangles) were presented during acquisition and extinction (Fig. 1 and see online Supplementary Methods) in a differential conditioning paradigm, where the CS+ signaled the US and the CS– its absence. The US was a painful stimulus. In one context the cue did not predict the US [unpredictable (unpred)], in the other context a cue reliably predicted the US [predictable (pred)]. There were two additional contexts with different configuration of the furniture in which no painful stimulus occurred (safe). The perspective rotated slightly from right to left and right again, so that each of the four walls of a room was entirely visible at least once. The virtual contexts consisted of several objects (bookshelves, chest of drawers, floor lamp, potted plant, racks, seating corner, television, Fig. 1) and were built using an online software toolbox Open-Source Graphics Rendering Engine (OGRE; http://ogre3d.org) and the support of a software company (Glodeck Software GmbH) using Visual Studio Professional (2010, Redmond, WA, USA). The furniture in each individual room was visible the entire time, while a participant was exploring the context. Only the camera perspective shifted as depicted in Fig. 1. The arrangement of the objects differed for each context but the objects were identical, thus forcing configural processing for context differentiation. Two colored CS± squares were presented on the walls of each context in a counterbalanced fashion. We a priori chose particular time windows (gray squares in Fig. 1) in each context for the extraction of fMRI and SCR data.

Fig. 1. During context acquisition, participants were passively walked through four different contexts. In the first context, the appearance of an unconditioned stimulus (US) could not be predicted by a single cue but only by the configuration of the furniture in the room as a whole (unpredictable). In the second context, the occurrence of an US could be predicted by a single cue (predictable). In between, two contexts were presented, in which no US was present (safe). The virtual room had a size of 4 × 4 m and individuals were walked on a predefined path through each room for 60 s until they entered the next room. On this path, the camera angle changed, so that participants could see each wall of the room completely at least once (see images of rooms and virtual room outlines 1 and 2). Each room entry was considered a trial. The presentation of the stimuli changed from trial to trial according to the context participants were in. Predefined context triggers (ctx) were set for which the functional brain activity and the skin conductance were read out. These were invisible to participants. The participants were then presented with colored triangles, one signaling the US (CS+), and one signaling the absence of the US (CS–). The procedure for all four contexts was repeated during the extinction phase without the presentation of the US. cs, conditioned stimulus; ctx, time window for context trigger; pred, predictable; ITI, inter-trial-interval; us, unconditioned stimulus.
Statistical analysis
All statistical analyses were performed in R-Statistics (Team, Reference Team2013). Data were assessed for outliers, normal distribution, homoscedasticity, and multicollinearity. All assumptions were met, if not mentioned otherwise below. Demographic and clinical data as well as self-reports and SCRs were analyzed with analyses of variance (ANOVAs) or independent t tests in case of two sample comparisons (e.g. trauma characteristics). The χ2 tests were performed to assess statistical differences in frequency distributions (e.g. gender). For the region-of-interest (ROI) analyses, we performed ANOVAs including the factors group (PTSD, TC, HC) × context (unpred, pred) × hemisphere (left, right) separately for the acquisition (ACQ) and extinction (EXT) phase as well as brain region (hippocampus, amygdala, vmPFC). Based on our a priori hypotheses, we only compared the functional activity within the above-mentioned ROIs and in the contexts, in which a painful stimulus occurred (unpred, pred). We further applied Tukey's honestly significant difference test as post-hoc single-step comparison procedure. The post-hoc t tests were Bonferroni corrected for the number of comparisons possible in each case. The number of levels of each factor (3 groups × 2 contexts: 6) defined the correction applied. There were few cases of missing data, motion artefacts, or outliers (see Results).
The online Supplementary Methods include information about participants, stimuli and experimental procedure, SCRs, clinical and neuropsychological assessments, self-reports, MRI data acquisition and analysis, manipulation check, and statistical analysis.
Results
Sample characteristics
The experimental groups did not significantly differ in any of the demographic variables except for education [χ2(2, 62) = 9.67, p = 0.008]. Patients with PTSD had a significantly lower level of education than the TC and HC group (p = 0.015; Table 1). All detailed information on demographic data, trauma severity, PTSD assessment, and comorbidities can be found in Table 1 and the online Supplementary Results. Patients with PTSD scored significantly higher on neuroticism [F (2, 56) = 12.87, p < 0.001] and lower on agreeableness [F (2, 56) = 4.98, p = 0.010] than HCs or TCs. There were no other significant differences between the experimental group in personality traits or neuropsychological assessments. We describe the results on personality traits and neuropsychological assessment in more detail in online Supplementary Table S1 and in the online Supplementary Results. There was no significant difference between the experimental groups on any of the debriefing questions concerning the difficulty of the study (online Supplementary Table S2 and Supplementary Results).
Functional magnetic resonance imaging
During acquisition, there was a significant interaction of group × context [Fgroup×context (2, 47) = 3.42, p = 0.04] in the hippocampus with significantly higher β values in HCs than patients with PTSD in the unpredictable context (p adj. = 0.035). In addition, HCs had significantly higher β values in the hippocampus during the unpredictable in comparison to the predictable context (p adj. = 0.0001). For the interaction group × context [Fgroup×context (2, 47) = 3.42, p = 0.04] in the amygdala a post-hoc t test revealed a significantly higher β value in the predictable context in HCs than in patients with PTSD (p adj. = 0.006) and HCs in comparison to TCs (p adj. = 0.02). Furthermore, HCs had significantly higher β values in the unpredictable in comparison to the predictable context (p adj. = 0.003). There was no other significant main effect or interaction during acquisition (Fig. 2; Table 2).

Fig. 2. Extracted β values for region-of-interest (ROI) analyses on the hippocampi, amygdalae, and vmPFC during acquisition (ACQ; top row) and extinction (EXT; bottom row) for each group of the three experimental groups. The β values were extracted for each context separately (unpredictable, predictable) selecting specific time windows in each context (gray squares in Fig. 1). We calculated 3 (groups: HC, TC, PTSD) × 2 (context: unpredictable, predictable) × 2 (hemisphere: left, right) analyses of variance. During acquisition, there was a significant group × context interaction. Post-hoc t tests revealed significantly higher hippocampal activity in the unpredictable context for subjects in the HC group in comparison to patients with PTSD. Furthermore, healthy participants showed higher activity in the unpredictable in comparison to the predictable context. During acquisition, we found a marginal significant group × context interaction in the amygdala. The significant post-hoc t test results are depicted for completeness, but we only interpret them with caution in the result section. During extinction, there was a significant group × context interaction. Subjects in the TC group showed significantly higher vmPFC activity in the predictable context than subjects in the HC group or patients with PTSD. Furthermore, participants in the TC group showed significantly higher activity in the predictable in comparison to the unpredictable context. ACQ, acquisition; EXT, extinction; HC, healthy control subjects without trauma experience; PTSD, patients with PTSD; ROI, region of interest; TC, healthy control subjects with trauma experience; vmPFC, ventromedial prefrontal cortex.
Table 2. Extracted β values for region-of-interest (ROI) analyses on the hippocampi, amygdalae, and vmPFC for context unpredictable (unpred) and context predictable (pred) during acquisition and extinction and for each group (p < 0.05).
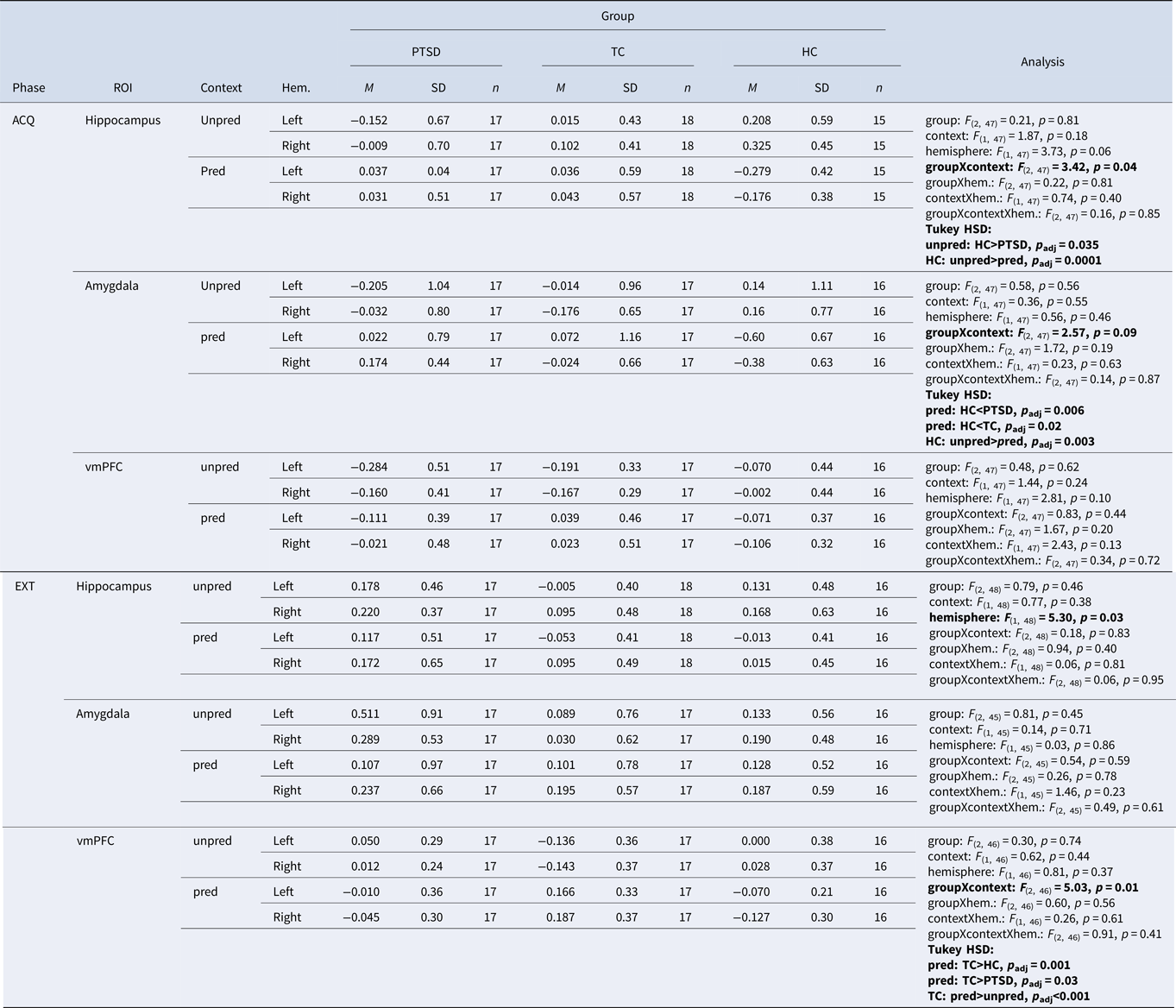
ACQ, acquisition; EXT, extinction; HC, healthy control subjects without trauma experience; hem, hemisphere; M, mean; pred, predictable; n, number of participants; PTSD, patients with PTSD; TC, healthy control subjects with trauma experience; unpred, unpredictable; p adj, Bonferroni corrected by possible number of post-hoc t tests; ROI, region of interest; SD, standard deviation; vmPFC, ventromedial prefrontal cortex. Values marked in bold show alpha < .05, bonferroni adjustment applied.
During extinction, we found a significant interaction of group × context [Fgroup×context (2, 46) = 5.03, p = 0.01] in the vmPFC. A post-hoc t test revealed a significantly higher β value in TCs than patients with PTSD (p adj. = 0.03) and TCs in comparison to HCs (p adj. = 0.001), both in the predictable context. Furthermore, TCs showed higher β values in the vmPFC in the predictable than in the unpredictable context (p adj < 0.001). There was no other significant main effect or interaction during extinction (Fig. 2; Table 2).
Skin conductance
During acquisition, there was a significant main effect for context in the unpredictable [Fcontext (1, 36) = 14.55, p < 0.001] in comparison to the safe and the predictable [Fcontext (1, 34) = 66.07, p < 0.001] in comparison to the safe contexts. There was also a main effect of group in the predictable condition [Fgroup (2, 34) = 5.45, p < 0.009] with higher SCRs for participants in the HC group than patients with PTSD or TCs. We did not find a significant main effect of group during the unpredictable context condition, nor any significant interaction of group × context (Fig. 3; online Supplementary Table S4a). Patients with PTSD in comparison to HC and TC subjects showed a similar SCR in the unpredictable context. However, we found a significantly lower SCR in the predictable context for patients with PTSD in comparison to the two healthy control groups during the predictable context.

Fig. 3. SCRs across each of the four conditions (unpredictable, predictable, 2×safe), two phases (acquisition, extinction), and each of the three experimental groups. (a) Skin conductance response is depicted during fear acquisition separately for the different contexts. (b) Skin conductance response is depicted during fear extinction separately for the different contexts. HC, healthy control subjects without trauma experience; PTSD, patients with PTSD; TC, healthy control subjects with trauma experience; μS, microSiemens.
Differences in SCRs between CS+ and CS–
During contextual fear acquisition, we found a significant main effect of group for the mean difference of CS+ and CS– in the unpredictable [Fgroup (2, 36) = 3.93, p = 0.029] in comparison to the safe and in the predictable [Fcontext (1, 34) = 62.59, p < 0.001] in comparison to the safe contexts. Patients with PTSD and TC subjects showed a significantly lower CS± differentiation than the HC subjects in the unpredictable contexts. In the predictable contexts, the groups did not significantly differ in their difference scores but showed overall higher scores in the predictable context than in the safe context. There was no other significant main effect or interaction of group × context. During context extinction, we found a significant main effect of context for the mean difference of CS+/CS– [Fcontext (1, 35) = 5.47, p = 0.025] with lower SCRs in the unpred in comparison to the safe context. There was no other significant main effect of interaction of group × context (online Supplementary Fig. S3 and Table S4b).
Self-report
Ratings across contexts
We found significant main effects of phase (HAB, ACQ, EXT), separately across all four contexts (unpred, pred, 2× safe) for the arousal and contingency ratings with the highest scores during acquisition and the lowest scores during extinction. For the valence ratings, we found a significant main effect of phase in the unpredictable context, with higher ratings in the acquisition than in the habituation or extinction phase and a significant group × phase interaction (online Supplementary Fig. S2a and Table S3b–d). Patients with PTSD in comparison to HC and TC subjects reported similar arousal, valence, and contingency ratings during the unpredictable context. There was no significant difference in the ratings between the groups in the predictable context.
Differences in ratings between CS+ and CS–
There were significant main effects of phase during acquisition for ratings of arousal [Fphase (1, 56) = 39.13, p < 0.001], valence [Fphase (1, 56) = 21.54, p < 0.001], and contingency [Fphase (1, 56) = 42.89, p < 0.001], showing that all three groups successfully learned the differential cue conditioning in the acquisition phase. There were no other significant main effects of phase or group nor any significant interaction of group × phase (online Supplementary Fig. S2b and Table S3e).
Discussion
The present study investigated differences in functional activity in the hippocampus, amygdala, and vmPFC in unpredictable and predictable contextual fear learning in patients with PTSD in comparison to healthy trauma or non-trauma exposed control subjects. During contextual fear acquisition, patients with PTSD showed significantly lower functional brain activity in the hippocampi in the unpredictable context and significantly higher activity in the amygdalae and SCR in the predictable context than HC subjects. In addition, HC subjects displayed a main effect of context, with higher activity in the unpredictable in comparison to the predictable context for the hippocampus and amygdala. During contextual fear extinction, patients with PTSD showed significantly lower activity in the vmPFC in the predictable context than TC subjects. Here, TC subjects had significantly higher activity patterns in the vmPFC in the unpredictable in comparison to the predictable context. There were no significant differences between the groups in the behavioral ratings for the contexts. Learning about the predictability of an aversive stimulus within a given context and overwriting what one has learned during extinction are key mechanisms of associative learning. In this novel Virtual Reality-based combined context-cue conditioning paradigm, we show that both context and cue in context conditioning are altered in patients with PTSD.
During contextual fear acquisition, we showed that patients with PTSD display lower functional brain activity in the hippocampi in the unpredictable and higher functional brain activity in the amygdalae in the predictable context. This fits well with findings that the hippocampi are involved in contextual fear acquisition of complex environments (Maren et al., Reference Maren, Phan and Liberzon2013) and that difficulties in contextual fear acquisition in patients with PTSD might be associated with difficulties in configural learning, which was required in our study (Acheson et al., Reference Acheson, Gresack and Risbrough2012). The amygdala in contrast has been associated to cued processing (Maren et al., Reference Maren, Phan and Liberzon2013; Rudy, Reference Rudy2009) and is well established as a region of interest in PTSD within a larger brain network processing salience and threat (Shalev et al., Reference Shalev, Liberzon and Marmar2017). Previous studies have shown that behavioral and psychophysiological responses as well as functional brain activity during contextual fear learning often show a contrasting picture (Baeuchl, Meyer, Hoppstädter, Diener, & Flor, Reference Baeuchl, Meyer, Hoppstädter, Diener and Flor2015; Steiger et al., Reference Steiger, Nees, Wicking, Lang and Flor2015; Wicking et al., Reference Wicking, Steiger, Nees, Diener, Grimm, Ruttorf and Flor2016). In line with these findings, we did not observe any differences in behavioral valence, arousal, or contingency ratings between the experimental groups, neither during acquisition nor extinction. We showed significantly lower SCRs in patients with PTSD in comparison to both healthy control groups as a response to the predictable context. This is in line with findings in cue-only conditioning studies reporting higher amygdala activity in patients with PTSD suggesting increased threat detection with lower peripheral psychophysiological reactions. In line with this, we did not find a significant correlation between amygdala activity and SCRs. This might be interpreted in a way that higher amygdalae activity in patients suggests that some information about the threat is learned and increases the predictability of the cue, which might minimize the peri-physiological response. Our study highlights that it is essential to distinguish the predictive power of the occurrence of an aversive stimulus in a given context and whether a threat can be predicted by a single cue or only by a more complex configuration of objects (e.g. furniture). Furthermore, operationalizing context learning via a configural learning approach facilitates to overserve cue and context-related responses in the same environment.
During contextual fear extinction, we showed higher functional brain activity in the vmPFC in TC subjects in comparison to patients with PTSD and HC subjects. The vmPFC is commonly found to be engaged during fear extinction (Lang et al., Reference Lang, Kroll, Lipinski, Wessa, Ridder, Christmann and Flor2009; Maren et al., Reference Maren, Phan and Liberzon2013), and has been repeatedly associated with the inhibition of fear responses. Differences in the activity of the vmPFC between both TC v. patients with PTSD as well as TC v. HC and its association to higher emotion regulation in TCs are in line with psychobiological models of PTSD (Shalev et al., Reference Shalev, Liberzon and Marmar2017) and a recent neuroimaging meta-analysis (Stark et al., Reference Stark, Parsons, Van Hartevelt, Charquero-Ballester, McManners, Ehlers and Kringelbach2015). The vmPFC is vital for emotion regulation during fear extinction, particularly for cued-based learning (like in a predictable context), and thereby to downregulate the salience network (Lanius, Frewen, Tursich, Jetly, & McKinnon, Reference Lanius, Frewen, Tursich, Jetly and McKinnon2015) and associated physiological responses. More generally, difficulties in contextual fear extinction and extinction recall in PTSD have been associated with lower functional activity in the vmPFC (Glenn et al., Reference Glenn, Risbrough, Simmons, Acheson and Stout2017; Wicking et al., Reference Wicking, Steiger, Nees, Diener, Grimm, Ruttorf and Flor2016), which is also related to structural white and gray matter reduction in the vmPFC (Siehl, King, Burgess, Flor, & Nees, Reference Siehl, King, Burgess, Flor and Nees2018; Siehl et al., Reference Siehl, Wicking, Pohlack, Winkelmann, Zidda, Steiger-White and Nees2020). While some studies do report hippocampal involvement during contextual fear extinction (Milad et al., Reference Milad, Wright, Orr, Pitman, Quirk and Rauch2007) or contextual retrieval (Maren et al., Reference Maren, Phan and Liberzon2013), the findings in the literature do not yield a consistent picture. We suggest that one common factor between contextual fear acquisition and extinction might be the difference in functional brain activation between unpredictable and predictable contexts in healthy control subjects but not in patients with PTSD. Our findings are in line with recent models examining PTSD in a predictive-coding framework (Seriès, Reference Seriès2019). Forming accurate predictions about the safety of one's environment is necessary for the ability to plan actions and interpret bodily sensations. If uncertainty about the danger of safety of the surrounding environment is high, due to difficulties in forming a stable mental representation of the context through configural learning, the prediction may favor safety. High levels of anxiety might be the consequence for patients with PTSD, preparing for a potential danger and paying a metabolic price for a contextual prediction that fails to be updated. Future studies will have to further assess the role of building (via configural learning) a mental representation of a context in contrast to elemental contextual learning as two important factors for predictability in contextual fear learning.
Limitations
Two main limitations apply to our study. Whereas the complexity of the design allows for the simultaneous examination of context- and cue-related triggers and their interaction, the design limits the choice of where to select the context triggers in each environment. To minimize overlapping, or additive effects in SCR or Blood Oxygenation Level Dependent activity, the triggers had to be far enough apart from each other. This, however, extended each trial to 50 s, which in turn limited our total number of trials per given condition to eight. With this rather low number of trials, each missing data point became a potential dropout. The interdependence of the triggers in the unpredictable context was also a problem. Here, almost each trial represented a unique composition of positions for the CSs, US, and context triggers. A fear response in a given context is most likely not limited to a single predictive cue or multiple contextual features but might also generalize to objects being non-predictive to the occurrence of the aversive stimulus. This is, however, a problem in many studies and not limited to our study (Lonsdorf et al., Reference Lonsdorf, Menz, Andreatta, Fullana, Golkar, Haaker and Merz2017) and the presented results have to be interpreted within the boundaries of the study design. A second limitation concerns a rather high number of potential non-responders in the SCR assessment. The SCR was measured on the foot, instead of the hand of participants, because participants received the painful stimulus on the left hand and responded with the response pad on the right hand. The signal on the foot might not have been strong enough. A potential solution could be to measure SCR on the shoulder instead (van Dooren, de Vries, & Janssen, Reference van Dooren, de Vries and Janssen2012). Finally, participants were wearing HMDs outside the scanner and MRI-suitable goggles inside the scanner. While unfortunately, there are no commercially available MRI-suitable HMDs yet to our knowledge, we argue that the use of MR goggles changed the experience in our study, which differed from ‘just’ watching a video due to increased immersiveness during both habituation in Virtual Reality and acquisition and extinction with MRI goggles, in comparison to using a mirror or screen. A mirror or screen is more static and a background or context is visible of the MRI measurement room, which is not the case for MRI-suitable goggles. Since context plays a key role in our study, we argue that this context perception is increased. However, this needs to be empirically tested.
Conclusions
In this cross-sectional study, we show that patients with PTSD when compared to TC and HC subjects display lower hippocampal activation in unpredictable and higher amygdala activity in predictable contextual fear acquisition as well as lower activity in the vmPFC during extinction of predictable contexts. Our results support the model that patients with PTSD show deficiencies in configural learning, while being more sensitive to cue-based learning and highlight the importance of predictability of fear in contextual learning. Trauma-focused exposure-based treatments might benefit from increasing predictability during the integration of contextual features within traumatic memories during exposure.
Supplementary material
The supplementary material for this article can be found at https://doi.org/10.1017/S0033291722003695.
Acknowledgements
We thank Birgül Sarun and Claudia Stief for their help in data acquisition and Michaela Ruttorf for advice regarding data analysis. This work was supported by two grants of the Deutsche Forschungsgemeinschaft (SFB636/C1 to Herta Flor; SFB1158/B03 to Herta Flor and Frauke Nees) and co-funded by the European Union under Grant No. 101057429.
Financial support
The authors report no financial relationships with commercial interests.
Conflict of interest
All authors declare that the research was conducted in the absence of any commercial or financial relationships that could be construed as a potential conflict of interest. The datasets and scripts for analyses are made available online. Readers seeking further information are welcome to contact the corresponding author.