Diabetes mellitus (DM) is a metabolic disease characterised by chronic hyperglycaemia and is one of the most common chronic diseases in almost all countries, caused either by defects in insulin secretion and insulin action or by defects in both(Reference DeFronzo, Bonadonna and Ferrannini1). The increase in the number of type 2 DM (T2DM) patients can be attributed to a change in their routines such as reduced physical activity or exercise. The incidence of T2DM has been increasing globally for decades and has consumed, and will continue to do so, a vast amount of medical resources; the expenditure is predicted to increase up to 300 million US dollars worldwide by 2025(Reference Gadsby2). The complications of T2DM lead to both a large personal burden and socio-economic cost. CHD and diabetic cardiomyopathy (DC), in particular, are the major causes of morbidity and mortality associated with diabetes. According to previous reports, diabetic patients have a 5-fold increased risk of developing CHD(Reference Haffner, Greenberg and Weston3, Reference Manson, Colditz and Stampfer4). Several mechanisms such as dyslipidaemia, glycosylation product accumulation and insulin resistance have been indicated to contribute to endothelial dysfunction in T2DM(Reference Storey, Perry and Petrie5).
As high blood glucose levels are one of the leading causes of cardiomyopathy, an efficient blood glucose control strategy is crucial for effective cardioprotection. Currently, insulin injection is a routinely practised clinical prescription, but insulin shock is a serious side effect that has to be considered before it is prescribed(Reference Oduru and Ahmad6).
The members of lactobacilli and bifidobacteria are extensively being used for the development of novel biotherapeutic probiotic formulations for the management of several diseases. The effects of probiotics on various gut-related diseases have been investigated extensively in several in vitro and in vivo experiments and in human clinical trials(Reference Kaur, Chopra and Saini7–Reference Laitinen, Poussa and Isolauri9). Probiotic treatment destroys β-cells in the islets of Langerhans in type 1 DM mouse models and decreases insulin levels and improves insulin-binding potential in T2DM mouse models(Reference Matsuzaki, Yamazaki and Hashimoto10, Reference Matsuzaki, Nagata and Kado11). However, the prospects of probiotic application in the management of lifestyle diseases, particularly diabetes, obesity and CVD, have not been explored extensively(Reference Panwar, Rashmi and Batish12). Lactobacillus rhamnosus GG has been reported to be a probiotic bacterium that improves insulin sensitivity in mice by promoting the transcription of GLUT4 and augmenting the activation of AMP kinase in skeletal muscles and adipose tissues(Reference Kim, Park and Kim13).
In streptozotocin (STZ)-induced DM animal models, left ventricular (LV) systolic dysfunction and diastolic dysfunction have been reported to develop after 12 weeks of induction(Reference Akula, Kota and Gopisetty14). However, treatment with a probiotic bacterium, Lactobacillus reuteri GMNL-263, has been reported to reduce glycated Hb levels and blood glucose levels in STZ-induced diabetes rats(Reference Lu, Yin and Chang15). Dahi, a fermented milk product containing Lactobacillus acidophilus NCDC14 and L. casei NCDC19, has been reported to decrease blood glucose levels in fructose-induced diabetes rats and to suppress STZ-induced oxidative damage in pancreatic tissues of DM rats(Reference Yadav, Jain and Sinha16). Similarly, probiotic pre-treatment with a mixture of L. acidophilus, Bifidobacterium lactis and L. rhamnosus has been reported to reduce blood glucose levels and further improve the bioavailability of gliclazide, a sulphonylurea drug used to treat T2DM in alloxan-induced diabetes rats(Reference Panwar, Rashmi and Batish12, Reference Al-Salami, Butt and Tucker17). The probiotic administration of Lactobacillus plantarum DSM 15 313 and L. reuteri GMNL-263 has been reported to lower blood glucose and glycosylated Hb levels, respectively, in high-fat diet-fed mice and STZ-induced diabetes rats(Reference Lu, Yin and Chang15, Reference Andersson, Branning and Ahrne18).
The Fas ligand- or TNF-α-dependent (type I) apoptotic pathway is one of the major pathways triggering cardiac apoptosis(Reference Huang, Yang and Lin19). Upon binding of the Fas ligand, the Fas receptor, a transmembrane receptor of the TNF receptor superfamily, recruits the Fas-associated protein with death domain (FADD) to bind to the death domain of Fas along with pro-caspase 8 to form the death-inducing signalling complex and trigger apoptosis(Reference Huang, Yang and Lin19). In our previous work in DM rats, the Fas/Fas ligand-induced apoptotic pathway, which involves the cleavage and activation of caspase 8, was found to induce apoptosis in cardiomyocytes and cause serious cardiac damage(Reference Huang, Yang and Lin19–Reference Lee, Kuo and Ho21). Herein, we report on an effective probiotic bacterial strain, L. reuteri GMN-32, and its protective effects on diabetes-related cardiomyopathy in STZ-induced diabetes rats.
Materials and methods
Preparation of bacterial suspensions
L. reuteri GMN-32 was provided by GenMont Biotech, Inc., and two different doses, 107 colony-forming units (cfu)/ml and 109 cfu/ml, of L. reuteri GMN-32 were prepared in PBS for oral administration to rats.
Animal model
All Sprague–Dawley rats were purchased from BioLASCO Taiwan Company Limited and separated into four groups (n 6 each). Group I consisted of control rats, group II consisted of STZ-induced DM rats, and groups III and IV consisted of STZ-induced DM rats treated with 107 cfu/ml per d and 109 cfu/ml per d of L. reuteri GMN-32, respectively. An ambient temperature was maintained at 22–24°C, and the rats were kept under an artificial 12 h light–12 h dark cycle. The light period began at 07.00 hours. The rats were provided with standard laboratory chow (Lab Diet 5001; PMI Nutrition International) and water ad libitum. All the rats were allowed to adapt to the environment for 3 weeks, and echocardiography was carried out on the test rats 2 d before STZ injection. After allowing the rats to fast for 24 h, DM was induced by administering a single intraperitoneal injection of STZ (50 mg/kg body weight) dissolved in 10 mm-sodium citrate, pH 7·0. The blood glucose levels of the rats were determined after 3 d of induction, and after an additional 3 d, probiotics were administered to the rats for the next 30 d. The blood glucose levels of the STZ-injected rats were monitored every day. After 30 d of probiotic oral administration, cardiac function was examined using echocardiography, and blood glucose levels were also determined. The rats were then killed, and their hearts were dissected and stored at − 80°C until analysis. All protocols were reviewed and approved by the Institutional Review Board and the Animal Care and Use Committee of the China Medical University, Taichung, Republic of China, and the study was conducted in accordance with the Principles of Laboratory Animal Care(22).
Haematoxylin and eosin staining
The hearts of the test rats were excised, soaked in formalin, dehydrated in graded alcohol (100, 95 and 75 %) and embedded in paraffin wax. The paraffin-embedded tissue blocks were cut into 0·2 μm-thick sections and deparaffinised by immersion in xylene. The slices were stained with haematoxylin and eosin and rinsed with water. Each slide was dehydrated using graded alcohol and rinsed twice in xylene. Photomicrographs were obtained using a Zeiss Axiophot microscope (Carl Zeiss Microscopy).
4,6-Diamidino-2-phenylindole staining and terminal deoxynucleotidyl transferase 2'-deoxyuridine 5'-triphosphate (dUTP)-mediated nick-end labelling assay
For the terminal deoxynucleotidyl transferase 2′-deoxyuridine 5′-triphosphate (dUTP)-mediated nick-end labelling (TUNEL) assay, tissue sections were incubated with proteinase K, washed with PBS, incubated with a permeabilisation solution followed by incubation in a blocking buffer, and washed twice with PBS. The sections were then incubated for 60 min at 37°C in terminal deoxynucleotidyl transferase and fluorescein isothiocyanate-dUTP provided in the apoptosis detection kit (Roche Applied Science). Under florescence (excitation wavelength 460 nm and detection 515–565 nm), TUNEL-positive nuclei (fragmented DNA) are illuminated in bright green. The sections were also stained with 0·1 μg/ml of 4,6-diamidino-2-phenylindole for 5 min, and the nuclei were detected by UV light microscopy at 454 nm. Photomicrographs were obtained using a Zeiss Axiophot microscope.
Tissue protein extraction
The cardiac tissue extracts of the rats were obtained by homogenising the LV samples in a lysis buffer (100 mg/ml). The homogenates were placed on ice and then centrifuged at 12 000 g for 40 min. The supernatants were collected and stored at − 80°C for use in further experiments.
Western blot analysis
The protein concentrations of the cardiac tissue extracts were determined using Lowry's protein assay method. Protein samples were separated by 12 % SDS–PAGE with a constant supply of 75 V. The proteins were then transferred onto polyvinylidene difluoride (GE Healthcare Life Sciences) membranes using 50 V current for 3 h. The membranes were incubated in 3 % bovine serum albumin in Tris-buffered saline (TBS) buffer followed by incubation with primary antibodies to specific proteins (Santa Cruz Biotechnology). Horseradish peroxidase-labelled secondary antibodies were used for detection, and pictures were taken with Fujifilm LAS-3000 (GE Healthcare Life Sciences).
Echocardiography
M-mode echocardiographic examinations were conducted using a 6–15 MHz linear transducer (15–6 L) via a parasternal long-axis approach. LV M-mode measurements at the level of the papillary muscles included LV internal end-diastolic dimensions, LV internal end-systolic dimensions, interventricular septum, posterior wall thicknesses, ejection fraction (EF) and fractional shortening (FS). EF (%) was calculated using the following equation:

where EDV is the end-diastolic volume and ESV is the end-systolic volume.
FS (%) was calculated using the following equation:

Statistical analysis
The results are reported as means and standard deviations of three independent experiments. Statistical analysis was performed using one-way ANOVA. For paired samples, Student's t test was used.
Results
Physiological features
The average body weight and blood glucose levels of the control group rats during the 30 d experiment were 346·1 (sd 50·5) g and 1068 (sd 74) mg/l, respectively. The average body weight of the STZ-induced DM rats was 269·33 (sd 46·1) g (22·18 % lower than that of the control group rats), and the average blood glucose level was 4472 (sd 334) mg/l (4·1-fold higher than that of the control group rats). The average body weight of rats treated with low-dose L. reuteri GMN-32 (107 cfu/d) was 266·9 (sd 15) g, which was comparable with that of the DM rats, but their blood glucose levels were 3617 (sd 247) mg/l (19·1 % lower than those of the DM rats). However, the average body weight of rats treated with high-dose L. reuteri GMN-32 (109 cfu/d) was 300·83 (sd 5·4) g (11·7 % higher than that of the DM rats), and the average blood glucose level was 3040 (sd 434) mg/l (32 % lower than that of the DM rats). From these results, it can be inferred that probiotic treatment can prevent the detrimental effects on the physiological features of DM rats.
Cardiac echocardiography
After 30 d of probiotic administration, heart EF (Teich) and FS were determined using cardiac echocardiography. The average EF (Teich) of the control group rats was 78·7 (sd 5·7) % and the average FS was 42·9 (sd 5·5) % (Table 1). The average EF (Teich) of the STZ-induced DM rats was 69·38 (sd 4) % (9·4 % lower than that of the control group rats), and the average FS was 34·7 (sd 3) % (8·2 % lower than that of the control group rats). The average EF (Teich) of the L. reuteri GMN-32 (107 cfu/d)-treated rats was 73·3 (sd 8·9) % (3·9 % higher than that of the DM rats), and the average FS was 38·3 (sd 3) % (3·62 % higher than that of the DM rats). The average EF (Teich) of the L. reuteri GMN-32 (109 cfu/d)-treated rats was 73·3 (sd 8·9) % (1·83 % higher than that of the DM rats), and the average FS was 36·5 (sd 2·9) % (1·8 % higher than that of the DM rats).
Table 1 Echocardiographic assessment of the effects of low (10−7 colony-forming units (cfu)/d) and high (109 cfu/d) doses of Lactobacillus reuteri GMN-32 treatment on the cardiovascular structure and function of the streptozotocin-induced diabetes mellitus rats (Mean values and standard deviations)
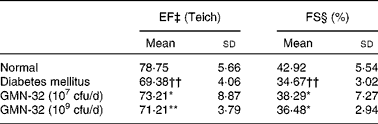
EF, ejection fraction; FS, fractional shortening; EDV, end-diastolic volume; ESV, end-systolic volume.
Mean values were significantly different from those of the control group: *P< 0·05, **P< 0·01.
†† Mean values were significantly different from those of the diabetes mellitus group (P< 0·01).
‡ EF (%) was calculated using (EDV − ESV)/EDV × 100.
§ FS (%) was calculated using (left ventricular internal end-diastolic dimensions − left ventricular internal end-systolic dimensions)/left ventricular internal end-diastolic dimensions × 100.
Cardiac characteristics
The prominent cardiac characteristics of the DM rats deteriorated significantly when compared with those of the control rats, but in the L. reuteri GMN-32-administered rat groups, the cardiac characteristics were found to remain relatively stable. The whole-heart weight (g) and LV weight (g) compared with the respective tibia lengths (mm) in the L. reuteri GMN-32-administered DM rat groups were higher than those in the DM rats. The whole-heart weight:tibia length ( × 103, g/mm) of the control rats, DM rats and low-dose and high-dose L. reuteri GMN-32-administered DM rats were 25·8, 21·8, 22·5 and 23·5, respectively, and the LV weight:tibia length values were 18·4, 15·2, 15·9 and 16·6, respectively (Table 2).
Table 2 Effect of Lactobacillus reuteri GMN-32 treatment on the cardiac characteristics of the streptozotocin-induced diabetes mellitus (DM) rats‡ (Mean values and standard deviations)
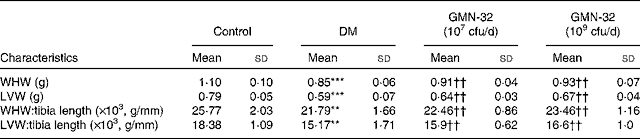
cfu, colony-forming units; WHW, whole-heart weight; LVW, left ventricular weight.
Mean values were significantly different from those of the control group: **P< 0·01, ***P< 0·001.
Mean values were significantly different from those of the DM group: ††P< 0·01.
‡ The effects of DM on the hearts of rats were significantly reduced when treated with low (107 cfu/d) and high (109 cfu/d) doses of L. reuteri GMN-32.
Heart biopsy
Haematoxylin and eosin staining of the cardiac tissue sections of the STZ-induced DM rats showed that the arrangement of the cardiomyocytes was disordered (Fig. 1). The arrangement of the cardiomyocytes of the L. reuteri GMN-32 (107 cfu/d)-treated rats was more ordered than that of the DM rats and was similar to that of the control group rats.
Fig. 1 Haematoxylin and eosin staining of cardiac tissue sections. Histopathological analysis of cardiac tissue sections of the left ventricles of the control rats, streptozotocin (STZ)-induced diabetes mellitus rats without treatment (sham), STZ-induced diabetes mellitus rats treated with a low dose of Lactobacillus reuteri GMN-32 (107 colony-forming units (cfu)/d) and STZ-induced diabetes mellitus rats treated with a high dose of L. reuteri GMN-32 (109 cfu/d). Haematoxylin stains basophilic structures such as the nucleus in blue and eosin stains eosinophilic structures in bright pink. The portions were magnified to 400 × . (A colour version of this figure can be found online at journals.cambridge.org/bjn).
Protein analysis
The expression levels of crucial heart proteins were examined by Western blot analysis of the cardiac tissue extracts. As indicated by the results, the expression levels of the Fas protein and the downstream protein FADD in the hearts of the STZ-induced DM rats were much higher than those in the hearts of the control group rats (Fig. 2(a)). The expression levels of Fas/FADD were also correlated with a corresponding increase in the levels of active cleaved caspase 8 in the hearts of the DM rats. The expression levels of Fas, FADD and caspase 8 in the hearts of the L. reuteri GMN-32 (107 cfu/d)-treated rats were found to be lower than those in the hearts of the DM rats. In the hearts of rats treated with a higher dose of L. reuteri GMN-32 (109 cfu/d), the expression levels of Fas, FADD and caspase 8 were further reduced, and those of Fas and caspase 8 were very similar to the levels in the hearts of the control group rats. Further analysis of the expression ratios showed that L. reuteri GMN-32 treatment controlled the expression of Fas, FADD and caspase 8 proteins in the hearts of the rats in a dose-dependent manner (Fig. 2(b)–(d)). The ratio of pro-caspase 8:cleaved caspase 8 increased from 1·9 (sd 0·05) in the control rats to 2·4 (sd 0·4) in the DM rats, but the ratios for the hearts of the low-dose and high-dose L. reuteri GMN-32-treated rat groups were 2·1 (sd 0·4) and 1·5 (sd 0·3), respectively, indicating a dose-dependent control of the activation of caspase 8 in the treatment groups.
Fig. 2 Protein expression analysis by Western blotting. The levels of the Fas/Fas-associated protein with death domain (FADD)/caspase 8 proteins increased in the left ventricles of the streptozotocin-induced diabetes mellitus rats, whereas treatment with low (107 colony-forming units (cfu)/d) and high doses (109 cfu/d) of Lactobacillus reuteri GMN-32 reduced the expression of these proteins. (a) Western blots of Fas/FADD/caspase 8 proteins. (b–d) Respective densitometry of the Western blots shown in (a). Quantifiable representation of the expression levels of (b) Fas, (c) FADD and (d) caspase 8 was done by normalising their expression with that of the α-tubulin as the internal control. Data represent the results of six animal models; the samples from two rats were pooled together and therefore three independent experiments were conducted. Values are means, with standard deviations represented by vertical bars. ** Mean values were significantly different from those of the control group (P< 0·01). † Mean values were significantly different from those of the GMN-32 (107 cfu/d)-treated diabetes group (P< 0·05). ‡‡ Mean values were significantly different from those of the GMN-32 (109 cfu/d)-treated diabetes group (P< 0·01).
Nucleic acid staining
The nuclei of cardiomyocytes were stained in blue with 4,6-diamidino-2-phenylindole, and specific DNA fragments resulting from caspase 8-mediated apoptosis were stained in green in the TUNEL assay. The 4,6-diamidino-2-phenylindole/TUNEL dual stain showed a higher (7·6-fold higher than that in the control group) number of apoptotic cell nuclei in the cardiac tissue sections of the STZ-induced DM rats (Fig. 3(a)). The cardiac tissue sections of rats treated with L. reuteri GMN-32 for 30 d had fewer apoptotic cell nuclei than those of rats that were not treated. The results showed that the treatment with the probiotic bacteria L. reuteri GMN-32 significantly reduced cardiac apoptosis that was found to occur in the STZ-induced DM rats.
Fig. 3 4,6-Diamidino-2-phenylindole (DAPI) and terminal deoxynucleotidyl transferase dUTP-mediated nick-end labelling (TUNEL) staining to detect apoptosis. (a) DAPI- and TUNEL-stained cardiac tissue sections of the control rats, streptozotocin (STZ)-induced diabetes mellitus rats and rats treated with low (107 colony-forming units (cfu)/d) and high (109 cfu/d) doses of Lactobacillus reuteri GMN-32. The nuclei were stained in blue after DAPI staining, and DNA fragments produced due to apoptosis were stained in green after the TUNEL assay. Fluorescent microscopy studies revealed cleaved nuclear DNA in the cardiac tissue sections of the STZ-induced diabetes mellitus rats. Treatments with low (107 cfu/d) and high (109 cfu/d) doses of L. reuteri GMN-32 reduced apoptosis to a level comparable with that observed in the control rats. (b) Corresponding quantifiable representation of the levels of apoptosis as detected by the TUNEL assay. (A colour version of this figure can be found online at journals.cambridge.org/bjn).
Discussion
Recent studies have shown that probiotics exhibit various biological activities, including anti-inflammatory, anticancer and antioxidant potential activities(Reference de Vrese and Schrezenmeir23). Lactic acid bacteria, in particular, are known to reduce blood glucose levels and decrease the complications of diabetes. Lactobacilli have also been reported to provide long-term cardioprotection against ischaemia and diabetes-induced apoptosis(Reference Huang, Yang and Lin19, Reference Oxman, Shapira and Klein24).
The STZ-induced diabetes animal model exhibits many of the pathophysiological deficits observed in diabetic humans, such as hypoinsulinaemia, hyperglycaemia, cardiac hypertrophy, cardiomyopathy, cardiovascular dysfunction and heart failure(Reference Shiomi, Tsutsui and Ikeuchi25–Reference Cheng, Ho and Yang27).
In the present study, blood glucose levels in 4-week-old STZ-induced DM rats increased to 4470 mg/l (four times higher than those in the normal group rats), and the rats lost 22 % of their body weight after induction. Body-weight loss is a common phenomenon in DM patients caused by imbalances in carbohydrate metabolism(Reference Franz28). In a healthy rat, the percentage value of EF is greater than 70 and the FS value is greater than 40, and in a healthy human, the percentage value of EF is greater than 55 and the FS value is greater than 30(Reference Gudmundsson, Rydberg and Winter29–Reference Soetikno, Sari and Sukumaran32). If the EF or FS value is below the normal range, conditions of heart dysfunction arise. A decrease in the values of EF (%) to 9·4 and in those of FS (%) to 8·2 reveals the occurrence of heart dysfunction in STZ-induced DM rats. In the present study, heart dysfunction was also accompanied by a deterioration of cardiac characteristics such as the whole-heart weight and the LV weight, demonstrating the occurrence of DC in the DM rats.
However, treatment with a high dose of L. reuteri GMN-32 (109 cfu/d) controlled the blood glucose levels at 3040 mg/l and maintained the body weight at 300·8 g in the STZ-induced DM rats. Furthermore, EF and FS values were above 70 and 36 %, respectively (Table 1). Whole-heart weight and LV weight measurements of the L. reuteri GMN-32-treated DM rats indicated these rats to have comparatively healthier hearts compared with the treatment group rats. Furthermore, biopsy by haematoxylin and eosin staining analysis did not show any differences in the hearts of the L. reuteri GMN-32-treated rats and control rats (Fig. 1). Therefore, the administration of L. reuteri GMN-32 is found to provide cardioprotection against DC in DM rats.
Normal blood glucose levels in healthy rats and humans are below 1000 mg/l(Reference Kawamori, Kadowaki and Ishida33). The average blood glucose level in the STZ-induced DM rats was found to be above 4470 mg/l, and L. reuteri GMN-32 treatment reduced the level to 3040 mg/l. Although blood glucose levels were not reduced to normal levels after L. reuteri GMN-32 treatment, the symptoms of DC syndrome were reduced. Thus, it can be interpreted that the risk of DC increases only when the blood glucose level rises above 3000 mg/l, and, therefore, control of blood glucose levels is critical in DM patients(Reference Poirier, Bogaty and Philippon34).
Diabetes is a strong risk factor for the development of cardiac hypertrophy, cavity dilation and heart failure in humans. The characteristic features of chronic heart failure include the progressive deterioration of LV function and the loss of cardiomyocytes via apoptosis or necrosis. Previous reports have shown that diabetes increases the apoptosis of cardiomyocytes in human hearts by 85-fold and causes a 30 % reduction in heart weight in rats(Reference Kuo, Chung and Liu35).
In our earlier studies, the activation of the cleavage of caspase 8 and apoptosis of cardiomyocytes by the Fas/FADD pathway was found to be the major cause of heart dysfunction(Reference Huang, Yang and Lin19, Reference Lee, Shyu and Cheng20, Reference Lee, Kuo and Ho21). Herein, we emphasise the same phenomenon with experiments carried out on the hearts of DM rats by evaluating the expression levels of the respective proteins (Fig. 2). Interestingly, cardiomyopathy-related symptoms were more noticeable after 12 weeks of induction in the STZ-induced DM experimental models used by Akula et al. (Reference Akula, Kota and Gopisetty14).
The Fas/FADD-induced caspase 8 cleavage and activation were significantly regulated with high-dose (109 cfu/d) and low-dose (107 cfu/d) L. reuteri GMN-32 treatments. However, biopsy analysis of the hearts of the STZ-induced DM rats showed a dose-dependent recovery after L. reuteri GMN-32 treatment (Fig. 1). Furthermore, the TUNEL/4,6-diamidino-2-phenylindole nucleic acid staining analysis showed that L. reuteri GMN-32 treatment decreased the incidence of apoptosis in cardiomyocytes (Fig. 3). The results showed that L. reuteri GMN-32 treatment reduced the blood glucose levels and thereby inhibited the Fas/FADD-mediated apoptotic pathway and prevented cardiomyopathy in DM rats. The mechanism of L. reuteri GMN-32-mediated blood glucose control in DM rats needs to be investigated further with additional experiments.
Diabetes is influenced by multiple factors, such as metabolism, oxidative stress, immune system and endocrine dysfunction(Reference Chbinou and Frenette36, Reference Lopes-Virella and Virella37). Therefore, the detrimental effects of STZ-induced diabetes and the protective effects of probiotic administration on cardiac changes cannot be attributed to one specific factor or any specific system, but to the direct or indirect regulation of various factors such as hyperglycaemia, oxidative stress, inflammation, and other unknown yet interacting factors.
In conclusion, because the results indicate that the administration of L. reuteri GMN-32 regulates blood glucose levels in DM rats to a reasonable threshold and reduces the DC risk, L. reuteri GMN-32 probiotic-assisted treatment can also potentially be used with other common treatments to control blood glucose levels in DM patients.
Acknowledgements
The present study was financially supported by the Taiwan Department of Health and Clinical Trial and Research Center of Excellence (DOH102-TD-B-111-004) and partly by China Medical University, Taichung, Republic of China (CMU99-NTU-01-1&2), without any direct role in the design and analysis of the study or in writing of this article.
The authors' contributions were as follows: C.-H. L., C.-C. L., M. A. S., C.-S. L., C.-H. K., F.-J. T., C.-H. T., C.-H. H. and C.-Y. H. designed the experiments; C.-H. L., C.-C. L., M. A. S., Y.-H. C. and C.-Y. H. analysed the results; M. A. S, C.-H. L. and C.-Y. H. prepared and edited the manuscript. All authors read and approved the final manuscript.
The authors declare that there is no conflict of interest.