Introduction
While studies on Early Middle Palaeolithic (EMP) archaeological assemblages in northwest Europe are focused on primary context archaeological sites (Hosfield & Chambers, Reference Hosfield and Chambers2004), many of these assemblages occur in secondary context (see e.g. Hosfield & Chambers, Reference Hosfield and Chambers2004; White et al., Reference White, Scott and Ashton2006; Scott et al., Reference Scott, Ashton, Lewis, Parfitt and White2011). These secondary context archaeological resources contain a wealth of information on hominin habitation history and are therefore critical for a better understanding of the EMP in northwestern Europe. One of these EMP secondary context assemblages occurs in the gravelly fluvial deposits in part of the ice-pushed ridges in the central Netherlands (Fig. 1) (e.g. Stapert, Reference Stapert, Ruegg and Zandstra1981, Reference Stapert1987, Reference Stapert and Ruegg1991; Van Balen, Reference Van Balen2006; Van Balen et al., Reference Van Balen, Busschers and Cohen2007). These fluvial sediments were deposited by a combined Rhine–Meuse fluvial system. The artefacts were made from gravel and cobbles that were transported by this fluvial system towards the central Netherlands (Stapert, Reference Stapert, Ruegg and Zandstra1981, Reference Stapert1987, Reference Stapert and Ruegg1991). Usage of locally available gravel was also inferred for other EMP archaeological sites in northwestern Europe (e.g. Roebroeks, Reference Roebroeks1988 for Maastricht-Belvédère; Tuffreau & Sommé, Reference Tuffreau and Sommé1988 for Biache-Saint-Vaast).
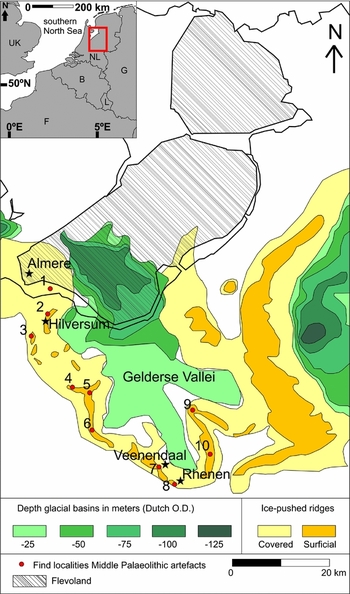
Fig. 1. Overview of glacial topography and find locations of EMP artefacts in the central Netherlands. Glacial topography after Busschers & Bakker (Reference Busschers and Bakker2009). Distribution of artefact find spots after Stapert (Reference Stapert1987) and Van Balen & Busschers (Reference Van Balen and Busschers2010): (1) Gooimeer (dredged finds); (2 & 3) Hilversum area (surface finds); (4) Tamme (Soesterberg); (5) Ecoduct Leusderheide; (6) Zanderij (Maarn); (7) Kwintelooijen (Veenendaal); (8) Kesteren (Rhenen); (9) De Goudsberg (Lunteren); (10) Fransche Kamp (Wageningen). Artefacts re-evaluated for this research were studied by Verpoorte (Reference Verpoorte2009) and Alink (Reference Alink2013). The red box in the inset shows the location of the study area within the Netherlands.
The EMP artefacts in fluvial deposits in the western part of the ice-pushed ridges in the central Netherlands have been the topic of several studies that deal with lithological characteristics (Ruegg, Reference Ruegg, Ruegg and Zandstra1981, Reference Ruegg and Ruegg1991) or technological analyses of the EMP assemblages (e.g. Stapert, Reference Stapert, Ruegg and Zandstra1981, Reference Stapert1987, Reference Stapert and Ruegg1991; Niekus, Reference Niekus1995; De Moor, Reference De Moor2008; Van Zon, Reference Van Zon2008; Van Homelen, Reference Van Homelen2009; Verpoorte, Reference Verpoorte2009; Alink, Reference Alink2013). In this study we investigate the relationship between the artefacts and the gravel and cobble distribution. One aim is to assess if there is a downstream decrease in artefact size, and, if so, to determine whether it can be explained by downstream fining of the fluvial deposits, being the source of the rocks. Another objective is to use the fluvial trends to infer whether artefacts could be present at other locations. In addition, we will discuss the palaeoenvironment of the hominins and the age range of the artefacts. We used a borehole database to determine the gravel and cobble distribution in the gravelly fluvial deposits of the ice-pushed ridges in the central Netherlands. For the borehole selection strategy of the buried part of these ice-pushed ridges we reconstructed the top of these ridges in the subsurface of the northwestern central Netherlands (southwest Flevoland; see Fig. 1).
Geographical and cultural setting
The (pre-)Saalian Rhine–Meuse deposits in the central Netherlands, which are part of the Sterksel- and Urk Formations (cf. Westerhoff et al., Reference Westerhoff, Wong, De Mulder, de Mulder, Geluk, Ritsema, Westerhoff and Wong2003), are dominated by gravelly medium to coarse sand (150–2000 µm) (Bosch et al., Reference Bosch, Weerts and Busschers2003; Westerhoff, Reference Westerhoff2003). The Urk Formation is distinguished from the older Sterksel Formation by its higher augite content (Zonneveld, Reference Zonneveld1956, Reference Zonneveld1958), related to the Eifel volcanism (~450 ka; Van den Bogaard & Schmincke, Reference Van den Bogaard, Schmincke and Schirmer1990; Boenigk & Frechen, Reference Boenigk and Frechen2006). In the central Netherlands the youngest part of the Urk Formation just pre-dates the maximum ice-sheet expansion (~150 ka; Busschers et al., Reference Busschers, Van Balen, Cohen, Kasse, Weerts, Wallinga and Bunnik2008). It consists of mixed Rhine–Meuse deposits (unit 3 of Busschers et al., Reference Busschers, Van Balen, Cohen, Kasse, Weerts, Wallinga and Bunnik2008). The base of these deposits contains gravel lag deposits with cobbles (Zandstra, Reference Zandstra, Ruegg and Zandstra1981; unit 5 of Ruegg, Reference Ruegg, Ruegg and Zandstra1981). In these gravel lags EMP artefacts are present (e.g. Stapert, Reference Stapert1987, Reference Stapert and Ruegg1991; Van Balen, Reference Van Balen2006; Van Balen et al., Reference Van Balen, Busschers and Cohen2007). During the maximum ice-sheet extent of the Saalian glaciation, towards the end of marine isotope stage (MIS) 6 (Van den Berg & Beets, Reference Van den Berg, Beets and Van der Meer1987; Busschers et al., Reference Busschers, Van Balen, Cohen, Kasse, Weerts, Wallinga and Bunnik2008), the Rhine–Meuse deposits, including the artefacts, were deformed into ice-pushed ridges. Subsequently they were eroded and partly covered by younger sediment, such as in the Almere region, southwest Flevoland (Fig. 1).
Find spots of artefact-bearing deposits
Early Middle Palaeolithic artefacts were found at various exposures in the central Netherlands (Fig. 1). Near Veenendaal (quarry Kwintelooijen), EMP flint artefacts have been found in a brown coarse sandy layer with gravels and cobbles (Stapert, Reference Stapert1987; unit 5 of Ruegg, Reference Ruegg, Ruegg and Zandstra1981). The lithology of the artefact-bearing deposit at Soesterberg (quarry Tamme) is similar to that found near Veenendaal (Stapert, Reference Stapert1987). While EMP artefacts have been found at Maarn (quarry Zanderij) (Stapert, Reference Stapert1987), their find context is unknown. Nevertheless, at quarry Zanderij layers of gravel are present that are assigned to the youngest part of the Urk Formation (Ruegg & Burger, Reference Ruegg and Burger1999). Most likely, these gravels were deposited by a braided fluvial system (Ruegg & Burger, Reference Ruegg and Burger1999). The presumable deposition of these gravels by a braided river, combined with the presence of deposits that were assigned to the youngest part of the Urk Formation, indicates that it is very likely that the gravel layers at quarry Zanderij are similar to the gravel lags found at Soesterberg and near Veenendaal. At several other find spots of EMP artefacts in the central Netherlands the stratigraphic context of the artefacts is similar to that of the examples above: a coarse-grained unit dominated by gravel (e.g. exposures A28 motorway: Stapert, Reference Stapert1987; Van Balen, Reference Van Balen2006; Busschers et al., Reference Busschers, Van Balen, Cohen, Kasse, Weerts, Wallinga and Bunnik2008; quarry Fransche Kamp: Ruegg, Reference Ruegg and Ruegg1991). In agreement with Stapert (Reference Stapert1987), we assume that throughout the find area these artefacts occur in one stratigraphic position, namely in the gravel lags at the base of a gravel-rich Rhine–Meuse deposit in the youngest part of the Urk Formation.
The artefact-bearing deposit also contains a combination of warm/temperate and cold climatic faunal remains. The combination of bones of different climatic periods is explained by reworking of older deposits (Stapert, Reference Stapert1987). Examples of cold climatic indicators that were found are Mammuthus primigenius, Coelodonta antiquitatis and Ovibos moschatus (open steppe biotope), and those of warm climate species are Elephas namadicus, Dicerorhinus kirchbergensis and Sus scrofa (wood/steppe landscape) (Van Kolfschoten, Reference Van Kolfschoten, Ruegg and Zandstra1981, Reference Van Kolfschoten and Ruegg1991). This mixture of warm and cold climate faunal remains makes it difficult to correlate EMP artefacts to palaeoclimatic conditions.
Early Middle Palaeolithic assemblages of the central Netherlands
While the Levallois method was often applied for artefact manufacture, some cores were hardly exploited (Stapert, Reference Stapert1987). The expedient style of core modification indicates that there was no scarcity of good-quality flint material (Stapert, Reference Stapert1987). Characteristic features of the EMP artefact assemblages are the high proportion of blades (Stapert, Reference Stapert, Ruegg and Zandstra1981), a dominance of scrapers among the tools (Stapert, Reference Stapert1987; Van Zon, Reference Van Zon2008) and the limited number of finished handaxes (Stapert, Reference Stapert1987).
Methods
Re-evaluation technological analyses of flint assemblages
For this paper Early Middle Palaeolithic flint artefacts from the quarries Kwintelooijen (near Veenendaal, N = 107), Tamme (Soesterberg, N = 64) and Zanderij (Maarn, N = 9), that were analysed by Verpoorte (Reference Verpoorte2009) and Alink (Reference Alink2013), are re-evaluated. Most of these artefacts were obtained by searching sieved sediment from the quarries (see Stapert, Reference Stapert1987 for Kwintelooijen; Alink, Reference Alink2013 for Tamme and Zanderij). An unknown part of the artefacts from Kwintelooijen was collected from the quarry surface (pers. comm. M.J.L. Franssen in De Moor, Reference De Moor2008). The artefacts from these quarries were obtained by various collectors over several decades (Kwintelooijen: M.J.L. Franssen between 1976 and 2001; Verpoorte, Reference Verpoorte2009; Zanderij: J. Offerman, E. Du Maine and A. Boelsma during the period 1970–1999; Tamme: J. Offerman and A. Boelsma between 1970 and 1985; Alink, Reference Alink2013). These collections are currently owned by the National Museum of Antiquities in Leiden (The Netherlands) (Verpoorte, Reference Verpoorte2009).
For this re-evaluation, only complete flakes were selected from the artefacts analysed by Verpoorte (Reference Verpoorte2009) and Alink (Reference Alink2013). For this study it is critical to focus on complete flakes because in contrast to cores and tools (e.g. handaxes), these flakes are not affected by use and therefore they are the most suitable artefact type to indicate a trend in metrical dimensions. In addition, flakes are the most common artefact type in the assemblages (see Verpoorte, Reference Verpoorte2009; Alink, Reference Alink2013), thereby providing a more robust statistical significance to indicate such a trend. These authors analysed flake dimension using the standard guidelines of De Loecker and Schlanger (Reference De Loecker and Schlanger2006). The largest flake size was used in this research, because this metrical dimension provides the best proxy to indicate the minimum size of the gravel particle from which the flake was produced. Abrasion was determined macroscopically by Verpoorte (Reference Verpoorte2009) and Alink (Reference Alink2013). The classification into none/light (sharp edges), medium (intermediate between sharp and heavily rounded edges) and heavy abrasion (overall heavily rounded edges) is relative, based on the studied material within a collection. Although there are other ways to categorise abrasion (e.g. Wymer, Reference Wymer1968; Shackley, Reference Shackley1975), the three-category subjective descriptor system based on edge damage is chosen in this study as edge damage can develop very rapidly via rolling or saltation (Hosfield & Chambers, Reference Hosfield and Chambers2004), providing insight in the percentage of artefacts that were transported short distances (less than 100 m; Hosfield & Chambers, Reference Hosfield and Chambers2004). This short fluvial transport distance is important for the discussion on the date of some of the EMP artefacts. In this research the largest sizes (median and range) of the selected flakes from the three quarries were compared. For the abrasion analysis, only selected flakes from Veenendaal and Soesterberg were taken into account, as the data on abrasion for Maarn include all flakes and not specifically complete flakes (see Alink, Reference Alink2013).
Mapping
A total of 164 selected digital core logs were used to construct a 50 m horizontal resolution elevation map of the top of the buried ice-pushed ridge in the subsurface of Almere (Fig. 2A). This map is important for the borehole location strategy used in this research as it shows the location of the top of this ridge, i.e. the area least likely to contain reworked Rhine–Meuse deposits. The selected core logs were obtained from the DINO database of TNO Geological Survey of the Netherlands (TNO, 2014). In all, 163 of these selected core logs contain Rhine–Meuse deposits. An additional core log (B25H0118) was selected following reinterpretation of the stratigraphy (see Supplementary Fig. S1 (available online at https://doi.org/10.1017/njg.2016.45)

Fig. 2. Digital elevation model of the central Netherlands. The maps have a ‘Rijksdriehoekstelsel’ coordinate system (surface elevation map obtained from www.ahn.nl). (A) Location of selected corings that were used for the construction of the elevation map of the top of the ice-pushed ridge in the subsurface of Almere (TNO, 2014). (B) Elevation of the top of the ice-pushed ridge in the subsurface of Almere in metres Dutch Ordnance Datum (O.D.) and overview of the core logs in the central Netherlands that were used in this research (see Supplementary Fig. S1 for detailed information on the corings).
At each core log, the elevation of the top of the Rhine–Meuse deposits is taken as the elevation of the top of the ice-pushed ridge. For four selected core logs this elevation was reinterpreted on the basis of the results of this study (core logs B25H0251, B25H0230, B25H0147 and B25H0120; Supplementary Fig. S1). The values of the top of the Rhine–Meuse deposits were interpolated to a grid of 50 m × 50 m using the kriging interpolation technique (Oliver & Webster, Reference Oliver and Webster1990; Goovaerts, Reference Goovaerts1997; Burrough & McDonnell, Reference Burrough and McDonnell1998). The newly created map provides for a more detailed topography of the ice-pushed ridge in the subsurface of Almere than has been published before (e.g. Jelgersma & Breeuwer, Reference Jelgersma, Breeuwer, Zagwijn and Van Staalduinen1975; Van den Berg & Beets, Reference Van den Berg, Beets and Van der Meer1987; Busschers et al., Reference Busschers, Van Balen, Cohen, Kasse, Weerts, Wallinga and Bunnik2008; Koopman & Pfeifer, Reference Koopman and Pfeifer2012).
Lithological analysis
After construction of the elevation map of the top of the ice-pushed ridge in Almere, 23 core logs were selected in the central Netherlands along the Almere–Rhenen transect that contain deposits assigned to the Urk Formation, the Sterksel Formation or to the Urk–Sterksel Formation if they cannot be classified to either the Urk or Sterksel Formation (cf. Fig. 2B and Supplementary Fig. S1). The borehole location strategy was specifically aimed at avoiding areas that could contain reworked deposits. Therefore, the selected cores are located on top of the ice-pushed ridges. The core logs were obtained from the DINO-core-database (TNO, 2014). Three of these selected core logs are located at the Early Middle Palaeolithic find localities of Soesterberg, Maarn and Veenendaal (cf. Figs 1 and 2B).
For 14 core logs the stratigraphic interpretation by TNO (2014) and Zandstra (Reference Zandstra, Ruegg and Zandstra1981) was slightly changed on the basis of the location of the corings (on top of the ridges), combined with lithostratigraphic indications and/or heavy mineral analysis results. Data on heavy mineral contents were obtained from Zandstra (Reference Zandstra, Ruegg and Zandstra1981) and the TNO Geological Survey database (TNO, 2015). Some of the core intervals were (re)assigned to the Urk, Urk–Sterksel or Sterksel Formation.
For some core logs the distinction between Urk and Sterksel Formation could not be made (e.g. core logs B26C0140, B25H0118, B26C0109, B32A0492, B32A0391, B32A0337, B32C0494 and B32C0559) (Supplementary Fig. S1). However, previous research indicates that there are no coarser deposits in the region than those assigned to these formations (Ruegg, Reference Ruegg, Ruegg and Zandstra1981, Reference Ruegg and Ruegg1991; Busschers et al., Reference Busschers, Van Balen, Cohen, Kasse, Weerts, Wallinga and Bunnik2008). Therefore, for each core interval that was assigned to the Urk, Urk–Sterksel or Sterksel Formation the coarsest gravel size and the presence and volume percentage of cobbles were determined. Data on the coarsest gravel size and the availability and volume percentage of cobbles of all selected core logs are used to determine the downstream fining trend. The different gravel sizes and the volume percentages of cobbles were categorised using TNO Geological Survey standard guidelines (Bosch, Reference Bosch2000). For this research these categories were numbered: (0) no gravel, (1) fine gravel (2–5.6 mm), (2) medium coarse gravel (5.6–16 mm) and (3) very coarse gravel (16–63 mm). To calculate per coring the percentage of each gravel category, the thickness of each core interval assigned to the Urk, Urk–Sterksel or Sterksel Formation is taken into account. Thicker intervals contribute more to the overall volume percentage of a gravel category than thinner intervals.
Results and interpretation
Comparison of the largest size and amount of abrasion of complete flakes from the selected flint assemblages
An overview of the largest size of the complete flakes from Veenendaal, Maarn and Soesterberg is shown in Figure 3. The amount of abrasion of these flakes from Veenendaal and Soesterberg is indicated in Figure 4. The median of the largest flake size is highest for the assemblage obtained from Veenendaal (5.6 cm), lower for the archaeological material from Maarn (3.9 cm) and lowest for the artefacts from Soesterberg (3.4 cm). The ranges in largest flake size are 3.3–10.8 cm for the artefacts from Veenendaal, 3.0–6.2 cm for those from Maarn and 1.9–6.2 cm for those from Soesterberg. Although for the Maarn assemblage the upper range of the largest flake size is similar to that of the artefacts from Soesterberg, this is not an integral part of the normal distribution of these assemblages, but related to the outliers that determine the extremes in the distributions. The majority of the flakes from Veenendaal have no or light abrasion (~71%), while for those from Soesterberg this is only ~36%. The most flakes with heavy abrasion were found at Soesterberg (25%) and the fewest at Veenendaal (9%). These results indicate that the more heavily abraded flakes are present in the downstream direction and that there is a downstream decrease in flake size.

Fig. 3. Boxplot of the largest flake size (in cm) of the EMP flint flakes from Veenendaal (N = 107), Maarn (N = 9) and Soesterberg (N = 64). The largest flake size is largest at Veenendaal and smallest at Soesterberg. The median is largest in Veenendaal and smallest at Soesterberg. Inset shows the values of the maximum (max.), third quartile (Q3), median, first quartile (Q1) and minimum (min.) in cm.

Fig. 4. Overview of amount of abrasion of the EMP flint flakes from Veenendaal (N = 107) and Soesterberg (N = 64). At Veenendaal the artefacts have the highest percentage of no/light abrasion and the lowest percentage of high abrasion. At Soesterberg the opposite is the case.
Lithological analysis
The results of the gravel size and cobble analysis of the 23 selected core logs from the Almere–Rhenen transect are shown in Figures 5 and 6. The gravel size distribution along this transect indicates a general gravel size fining in the NW direction. In the area around Rhenen, the gravel size is dominated by very coarse gravel. From Veenendaal up to Hilversum there is primarily medium coarse gravel. From Hilversum to Almere there is predominantly fine gravel or no gravel. The cobble analysis shows a similar fining trend. The majority of the cobbles (both in vol % and number of layers) are present in the southeastern part of the transect and this gradually decreases towards Hilversum. Although coring B32A0492 is an outlier when it comes to the number of layers that contain cobbles (three layers), the volume percentage of cobbles in those layers is very low (<1%). In the subsurface of Almere, cobbles are scarce.

Fig. 5. Overview percentage of coarsest gravel size category per core log and presence of cobbles along the Almere–Rhenen transect. These categories are based on standard guidelines (Bosch, Reference Bosch2000). The highest percentage of very coarse gravel is found around Rhenen and the lowest at Almere.

Fig. 6. Overview of the number of core intervals that contain cobbles and the volume percentage of cobbles per core log along the Almere–Rhenen transect. The majority of the cobbles are present near Rhenen. At Almere very few cobbles are present.
Discussion
Artefact size and downstream fining
Our analyses show that the northwestward decreasing trend in flake size corresponds with the downstream fining direction of the Rhine–Meuse system. The downstream decreasing size and abundance of locally available gravel and cobbles may explain the trend in artefact size. Several alternative explanations will be discussed below.
There were no large differences in the modification technique applied on the stone artefacts investigated in this research (see Stapert, Reference Stapert, Ruegg and Zandstra1981, Reference Stapert1987; Van Zon, Reference Van Zon2008; Verpoorte, Reference Verpoorte2009; Alink, Reference Alink2013). Also, the flint artefacts were all produced from the same flint type (Stapert, Reference Stapert1987, Reference Stapert and Ruegg1991). Differences in modification method or flint types can therefore not explain the decreasing flake size northwestward.
The trend in artefact size decrease is unlikely to be caused by differences in sample size, because the find locality with the smallest sample size (Maarn) has the second median largest flake size (Fig. 3). Another possible explanation for this trend is that smaller artefacts were transported further downstream than larger artefacts, because further downstream the percentage of heavily abraded flakes increases. Whether this increase is the result of a difference in transportation distance between smaller and larger artefacts or occurs because downstream a larger part of the artefact assemblage was fluvially reworked prior to deposition compared to upstream is currently unknown. However, transportation distance probably plays a limited role in the downstream fining trend of the artefact assemblages as there are still 36% of flakes with no or limited abrasion at Soesterberg (Fig. 4). These flakes could not have been transported over long distances, as experiments have shown that after 100 m of transport none of the artefacts is in mint condition (e.g. Harding et al., Reference Harding, Gibbard, Lewin, Macklin, Moss, Sieveking and Newcomer1987; Hosfield & Chambers, Reference Hosfield and Chambers2004).
Gravel of a comparable size to the smallest cores that have been produced at EMP archaeological sites in NW Europe (e.g. Roebroeks, Reference Roebroeks1988; De Loecker, Reference De Loecker2006; Scott, Reference Scott2006) is present along the entire transect between Almere and Rhenen (Figs 5 and 6), including the buried ice-pushed ridge beneath Almere, despite the downstream fining. This means that in the Almere region EMP artefacts could be present.
Artefact age
Based on stratigraphic observations and a tentative correlation to a warm climatic period, an age of 300–250 ka has been suggested for the artefacts by Stapert (Reference Stapert1987) and Niekus & Stapert (Reference Niekus, Stapert, Deeben, Drenth, Van Oorsouw and Verhart2005). Van Balen & Busschers (Reference Van Balen and Busschers2010) favour an age of 190–170 ka. They dated the artefact-bearing deposit by optically stimulated luminescence (OSL) at ~170 ka (Van Balen et al., Reference Van Balen, Busschers and Cohen2007; Busschers et al., Reference Busschers, Van Balen, Cohen, Kasse, Weerts, Wallinga and Bunnik2008). They argue that at least the hardly abraded part of the artefacts cannot be much older than the artefact-bearing deposit. The age implies cold climatic conditions during deposition of the artefact-bearing sediment, which is in agreement with the presence of periglacial phenomena in this deposit (i.e. syn-genetic ice-wedge casts, ice-rafted boulders and sedimentary structures indicative for a braided river) (Ruegg, Reference Ruegg and Ruegg1991, Reference Ruegg2008). In addition, the expedient flaking technique that was applied indicates that there was plenty of coarse gravel and cobbles available for artefact manufacture. An abundance of coarse gravel and cobbles in the river bed is more characteristic of a cold than a warm climatic period due to the sediment availability and energetic fluvial conditions (Van Balen & Busschers, Reference Van Balen and Busschers2010).
Although the hardly abraded artefacts most likely have a similar age to the deposits in which they are encased (Van Balen & Busschers, Reference Van Balen and Busschers2010), the abraded artefacts could be older as the sediments in which they were deposited may have been eroded and redeposited together with the MIS 6 deposits. This is indicated by the mix of mammal remains of both cold and warm climatic periods in the artefact layer (Stapert, Reference Stapert1987). In the former sand pit Fransche Kamp (Wageningen) (Fig. 1) a gravelly deposit was present beneath an MIS 7 clay interval underlying the artefact-bearing layer (Stapert, Reference Stapert1987). This gravel deposit contained no artefacts (Stapert, Reference Stapert1987), suggesting that the artefacts are younger than this deposit.
At the time of deposition of the MIS 7 clay interval, coarse gravels and cobbles were most likely scarce in the central Netherlands (Van Balen & Busschers, Reference Van Balen and Busschers2010), suggesting that local artefact manufacture was probably limited. However, artefacts could have been discarded in the region during MIS 7 as tools may have been transported by hominins to the central Netherlands from areas where coarse sediments were present (e.g. southern Limburg, southeast Netherlands; Van Balen, Reference Van Balen2006; Van Balen et al., Reference Van Balen, Busschers and Cohen2007). After these tools were discarded in the central Netherlands, fluvial erosion and aggradation of the floodplain and/or channel margin that occurs during an interglacial–glacial transition (Vandenberghe, Reference Vandenberghe1995; Bridgland, Reference Bridgland2000; Maddy et al., Reference Maddy, Bridgland and Westaway2001; Gibbard & Lewin, Reference Gibbard and Lewin2002) may have caused these artefacts to be encased in the MIS 6 sediments in which they are currently present. The abraded artefacts could therefore be as old as the MIS 7 clay layer. In the former sand pit Fransche Kamp (Wageningen) (Fig. 1), this layer contains faunal, pollen and mollusc remains indicative for interglacial conditions (De Jong, Reference De Jong and Ruegg1991; Meijer, Reference Meijer and Ruegg1991; Van Kolfschoten, Reference Van Kolfschoten and Ruegg1991). In this layer a more recent version of Arvicola terrestris is present than in sediments from Maastricht–Belvédère (Van Kolfschoten et al., Reference Van Kolfschoten, Roebroeks and Vandenberghe1993). At Maastricht–Belvédère, flint from the same layer as these faunal remains has been thermoluminescence-dated at ~250 ka (Huxtable, Reference Huxtable1993). Therefore, the clay layer at Fransche Kamp can be correlated to a warm, interglacial-like climatic period that is younger than 250 ka. The first warm period after 250 ka is dated between ~220 and 205 ka (MIS 7; Dutton et al., Reference Dutton, Bard, Antonioli, Esat, Lambeck and McCulloch2009), providing the maximum age for the EMP artefacts in the central Netherlands.
In conclusion, the artefacts in the central Netherlands most likely do not have a single age, but have an age range from 220 to 170 ka, corresponding to MIS 7 and early MIS 6. Sites dating to MIS 7 have been found throughout northwest Europe (see e.g. Callow, Reference Callow, Callow and Cornford1986; Roebroeks, Reference Roebroeks1988; Schäfer, Reference Schäfer1990; Huxtable, Reference Huxtable1993; Schreve, Reference Schreve1997; Adam, Reference Adam2002; Bahain et al., Reference Bahain, Gruppioni, Falguères, Dolo and Cliquet2008; Scott et al., Reference Scott, Ashton, Lewis, Parfitt and White2011; Faivre et al., Reference Faivre, Maureille, Bayle, Crevecoeur, Duval, Grün, Bemilli, Bonilauri, Coutard, Bessou, Limondin-Lozouet, Cottard, Deshayes, Douillard, Henaff, Pautret-Homerville, Kinsley and Trinkaus2014; Tizzard et al., Reference Tizzard, Bicket, Benjamin and Loecker2014). Although in the United Kingdom no sites are known dating to MIS 6 (see Scott et al., Reference Scott, Ashton, Lewis, Parfitt and White2011), in Belgium and western Germany archaeological sites dating to this period are present (see e.g. Haesaerts, Reference Haesaerts1978; Conard, Reference Conard1992; Pirson et al., Reference Pirson, Haesaerts, Di Modica, Di Modica and Jungels2009; Richter, Reference Richter2011). Part of the artefacts show hardly any abrasion and are therefore likely to have been made and left behind in the riverbed. These artefacts probably have approximately the same age as the artefact-bearing layer (~170 ka; Busschers et al., Reference Busschers, Van Balen, Cohen, Kasse, Weerts, Wallinga and Bunnik2008).
The southern North Sea area and its role in EMP hominin–environment interaction
Although sediments coarse enough for artefact manufacture are available in the subsurface of southwest Flevoland, the volume percentage of these lithics (very coarse gravel (16–63 mm) and cobbles (63–200 mm)) in the pre-glacial Rhine deposits is very small (<7%; Fig. 5). Due to the downstream fining of the combined Rhine–Meuse fluvial system, northwest of Flevoland the fluvial system most likely did not contain rocks coarse enough for artefact manufacture. As no other rock sources are known in the region that have material suitable for artefact manufacture by EMP hominins, the source for relatively coarse gravel and cobbles in southwest Flevoland is the most proximate to the eastern margin of the southern North Sea (i.e. western Netherlands and adjacent offshore area). Although EMP hominins could have visited the region northwest of Flevoland, they needed to return to the Flevoland area to refresh their lithic toolkit. This is of major importance to provide insight into EMP hominin landscape exploitation in the southern North Sea area as the mobility pattern is crucial in obtaining sufficient natural resources.
The role of the southern North Sea area in the exploitation pattern by EMP hominins in northwest Europe during MIS 7 and early MIS 6 is unknown. During the MIS 7 interglacial highstand, this role was most likely limited as a large part of the area was covered by water (Hijma et al., Reference Hijma, Cohen, Roebroeks, Westerhoff and Busschers2012). However, during lowstand situations the North Sea area was dry land (i.e. Rhine–Meuse delta; Cohen et al., Reference Cohen, Gibbard and Weerts2014). The accessibility of the area, combined with the availability of freshwater sources such as the Rhine and Meuse fluvial systems (Hijma et al., Reference Hijma, Cohen, Roebroeks, Westerhoff and Busschers2012), indicates that the southern North Sea area possibly played an important role in the subsistence economy of EMP hominins in northwest Europe (Roebroeks, Reference Roebroeks2014). Furthermore, due to the ameliorating environmental effect of the Atlantic Ocean, causing a reduced effect of seasonality due to limited changes in humidity (Van Koenigswald, Reference Van Koenigswald, Van Koenigswald and Werdelin1992), the most favourable region for habitation in northern Europe would be the North Sea area and its immediate surroundings, especially during the cool climatic conditions of early MIS 6. Several palaeo-environmental indicators exist to reconstruct the environmental setting of the central Netherlands and adjacent areas during early MIS 6. Sedimentological evidence shows that at that time the Rhine–Meuse fluvial system was a braided system (Ruegg, Reference Ruegg and Ruegg1991, Reference Ruegg2008; Busschers et al., Reference Busschers, Van Balen, Cohen, Kasse, Weerts, Wallinga and Bunnik2008). The Rhine–Meuse fluvial system must have been located in a low-lying position in the landscape, an incised valley, because later on, around 150 ka, the river valley was invaded by a glacier tongue of the Saalian ice sheet (Van den Berg & Beets, Reference Van den Berg, Beets and Van der Meer1987). To the north of the Rhine–Meuse system a mixed fluvio-aeolian environment existed (Van den Berg & Beets, Reference Van den Berg, Beets and Van der Meer1987; OSL-dated to ~190–150 ka by Kars et al., Reference Kars, Busschers and Wallinga2012). Involutions, frost wedges and a desert pavement indicate cold, periglacial conditions, likely a polar desert (Zagwijn, Reference Zagwijn1973).
EMP artefacts found in the southern North Sea area dating to MIS 7 and early MIS 6 were encountered in deposits from the rivers Rhine–Meuse (central Netherlands; e.g. Stapert, Reference Stapert, Ruegg and Zandstra1981, Reference Stapert1987, Reference Stapert and Ruegg1991), Thames (southeast UK; e.g. Scott et al., Reference Scott, Ashton, Lewis, Parfitt, White, Ashton, Lewis and Stringer2010a,Reference Scott, Ashton, Penkman, Preece and Whiteb) and the lower reaches of the palaeo-Yare (offshore of southeast England, Area 240; Tizzard et al., Reference Tizzard, Bicket, Benjamin and Loecker2014). The downstream fining trend and preservation of these deposits needs to be determined in order to assess the potential for future geoarchaeological research on EMP hominin activities in the North Sea area. This research is of major importance to provide insight into EMP hominin–environment interaction during MIS 7 and early MIS 6 in the southern North Sea area, which most likely contained very few rocks suitable for artefact manufacture, but probably contained a plethora of natural resources (e.g. freshwater and faunal species).
Conclusion
-
• Artefact size of EMP assemblages in the central Netherlands decreases northwestward.
-
• This trend in artefact size decrease corresponds to downstream fining of gravel and the downstream decrease of cobble abundance in Rhine–Meuse deposits in the central Netherlands.
-
• The gravel size and cobble abundance in the buried part of the ice-pushed ridges in the central Netherlands (Almere, southwest Flevoland) indicate that EMP artefacts could potentially be present in that area.
-
• Based on a literature review, the EMP artefacts in the central Netherlands have an age range from 220 to 170 ka (MIS 7 and early MIS 6).
-
• The downstream fining of the EMP artefact-bearing deposits in the southern North Sea and adjacent areas can possibly provide further insight into EMP hominin–environment interaction in these areas.
Acknowledgements
This article is part of the PhD research of Don F.A.M. van den Biggelaar at the VU University Amsterdam (VU). This research is part of the multidisciplinary ‘Biography of the New Land’ research programme of CLUE (VU), in collaboration with the Nieuw Land Heritage Centre (Lelystad, the Netherlands). This programme is jointly funded by the research institute CLUE (VU) and the Nieuw Land Heritage Centre (Lelystad, the Netherlands).
We thank TNO Geological Survey of the Netherlands (TNO) for facilitating access to their core- and heavy mineral database.
In addition, we thank Freek Busschers (TNO) for his support at TNO and discussing this topic with us. His comments greatly improved the quality of this research. Also, we would like to thank Roula Dambrink (TNO) and Jeroen Schokker (TNO) for their help with the TNO core-database.
Finally, we are very grateful for the constructive review by Jakob Wallinga (Wageningen University) and an anonymous reviewer. Their comments helped to improve the quality of this paper.
Supplementary material
Supplementary material is available online at https://doi.org/10.1017/njg.2016.45